Abstract
Diabetic cardiomyopathy (DCM), a serious complication of diabetes mellitus, is associated with changes in myocardial structure and function. This study sought to explore the ability of insulin-like growth factor-1 (IGF-1) to modulate DCM and its related mechanisms. Twenty-four male Wistar rats were injected with streptozotocin (STZ, 60 mg/kg) to mimic diabetes mellitus. Myocardial fibrosis and apoptosis were evaluated by histopathologic analyses, and relevant proteins were analyzed by Western blotting. Inflammatory factors were assessed by ELISA. Markers of oxidative stress were tested by colorimetric analysis. Rats with DCM displayed decreased body weight, metabolic abnormalities, elevated apoptosis (as assessed by the bcl-2/bax ratio and TUNEL assays), increased fibrosis, increased markers of oxidative stress (MDA and SOD) and inflammatory factors (TNF-α and IL-1β), and decreased phosphorylation of Akt and glycogen synthase kinase (GSK-3β). IGF-1 treatment, however, attenuated the metabolic abnormalities and myocardial apoptosis, interstitial fibrosis, oxidative stress and inflammation seen in diabetic rats, while also increasing the phosphorylation levels of Akt and GSK-3β. These findings suggest that IGF-1 ameliorates the pathophysiological progress of DCM along with an activation of the Akt/GSK-3β signaling pathway. Our findings suggest that IGF-1 could be a potential therapeutic choice for controlling DCM.
Diabetes mellitus (DM) is regarded as a dominant health problem. This metabolic disease is characterized by high levels of blood glucose reflecting defects in the insulin system, and it is associated with many deadly complications, including heart disease, kidney failure, and diabetic oculopathy [1]. The leading cause of death in diabetic patients, diabetic cardiomyopathy (DCM), is defined as changes in myocardial structure and function independent of the contribution of coronary artery disease, hypertension, valvular heart disease, or cardiac neuropathy [2]. In rats, DCM hearts exhibit disordered arrangements of myocardial cells with increased mitochondrial damage, apoptosis, and fibrosis [3]. In addition, diabetes has been shown to be an inflammatory disease, which was proved by excess production of inflammatory factors and reactive oxygen species [45].
It was reported that serum insulin-like growth factor-1 (IGF-1) is decreased in animals or patients with diabetes [67891011]. So, IGF-1 is a therapeutic target of diabetes. IGF-1 is an endogenous active polypeptide that shows a significant structural homology with proinsulin. IGF-1 is involved in the metabolic breakdown of three major nutrients and shows cardioprotective effects against oxidative stress, fibrosis and apoptosis [1012]. However, the underlying mechanisms involved are still poorly understood. IGF-1 has been shown to activate the phosphatidylinositol 3 kinase–Akt (PI3K–Akt) pathway [131415]. GSK-3β, a major downstream signaling molecule of Akt, mainly participates in the glycogen metabolism and insulin action, has a crucial role in DM-induced inflammation and apoptosis [1617].
Here, we tested whether the Akt/GSK-3β signaling pathway is involved in the IGF-1 induced amelioration of DCM in the streptozotocin (STZ)-induced model of diabetes in rats.
Twenty-four male Wistar rats (200~250 g) were obtained from the Yanbian University Laboratory Animal Center. Rats were kept in a temperature-controlled and air-conditioned conventional animal house with a 12-12 h light-dark cycle and free access to food and water. All surgical procedures and experimental protocols were approved by the Animal Care and Use Committee of Yanbian University Faculty of Medicine. The procedures were performed according to the recommendations of the Institutional Animal Care Committee.
Experimental rats were randomly divided into three groups: the normal control (Con), diabetes (DM), and IGF-1-treated DM (DM+IGF-1) groups. In the DM and DM+IGF-1 groups, diabetes was induced by a single intraperitoneal injection of STZ (60 mg/kg dissolved in 0.1 mol/L citrate buffer, pH 4.5; Sigma). The Con group was injected with the same amount of citrate buffer (0.1 mol/L). At 72 hours post-injection, blood samples were obtained by tail vein puncture, and blood glucose levels were measured using a hand-held glucometer (Shanghai Qiangsheng Medical Equipment, Shanghai, China). STZ-injected rats with blood glucose levels >16.7 mmol/L were used as DM animals. Rats randomized to the DM+IGF-1 group were treated with IGF-1 (1500 ng/kg, i.p.; Promega) daily for 10 weeks. The dose used was in the range of previous report [10]. All groups were supplied with basal diet for the 10 weeks of the experimental period.
Rats were fasted for 24 hours, and blood glucose, blood triglycerides (TG), blood total cholesterol (TC), urine volume were measured. The rats were then subjected to deep anesthesia (a single intraperitoneal injection of 3% sodium pentobarbital, 50 mg/kg body weight) and sacrificed by exsanguination. Body weight was measured using a scale.
A portion of heart tissue was fixed by immersion in 10% buffered formalin (pH 7.4) for 24 hours. The fixed tissue was dehydrated in graded ethanol, paraffin-embedded, and sectioned at a thickness of 4 µm. Paraffin-embedded tissue slices were baked at 60℃ for 1 hour, routinely dewaxed, rinsed, and stained with H&E.
Paraffin-embedded tissue slices were stained with Masson's trichrome, as described by the manufacturer (Nanjing SenBeiJia Biological Technology, Nanjing, China). This technique dyed the collagen fibers blue, the myocardium red, and the cell nuclei black. Ten randomly selected fields of Masson trichrome stained myocardial tissue slides were scored in three sections of each animal. Image analysis software (Image-Pro Plus 6.0) was used to calculate collagen area fraction and the average value was taken.
About 1 mm3 of fresh tissue was sampled from the left ventricle, immediately immersed in 2.5% glutaraldehyde for 2 hours, washed with PBS (0.1 mol/L, pH 7.4), fixed with 1% osmium tetroxide, and washed once more with PBS. Each sample was then dehydrated through graded ethanol (50%, 70%, 90%, 90%) followed by acetone, and finally embedded in Epon 812. Thin sections (60 nm) were cut with an ultramicrotome and stained with uranyl acetate and lead citrate. The ultrastructural changes of the tissues were observed by transmission electron microscope (Nihon Kohden, Japan).
Blood samples were obtained from the abdominal artery and sera were separated. The serum levels of interleukin-1 beta (IL-1β) and tumor necrosis factor-alpha (TNF-a) were assessed using ELISA kits (Nanjing SenBeiJia Biological Technology, Nanjing, China) according to the manufacturer's instructions.
SOD and MDA levels in left ventricular tissues were assayed using respective commercial kits (Nanjing Jiancheng Bioengineering Institute, Nanjing, China). The SOD activity was measured through the inhibition of nitroblue tetrazolium reduction by oxygen, which was generated by the xanthine/xanthine oxidase system. One SOD activity unit was defined as the enzyme amount causing 50% inhibition in a 1-mL reaction solution per milligram of tissue protein, and the result was expressed as U/mg protein.
The MDA concentration of the homogenate was measured using the thiobarbituric acid method. The amount of lipid peroxide measured by the production of MDA, which in combination with thiobarbituric acid, formed a pink chromogen compound with an absorbance of 532 nm. The result was expressed as nmol/mg protein.
TUNEL was performed using a commercially available kit (Promega, Wisconsin, USA) according to the manufacturer's instructions. Briefly, paraffin sections were conventionally dewaxed, hydrated, incubated with 20 µg/ml proteinase K at room temperature, and covered with equilibration buffer. The slices were then incubated with rTdT incubation buffer for 1 hour, and stained with 1 µg/ml propidium iodide.
Heart tissues were frozen, suspended in lysis buffer and phenylmethanesulfonyl fluoride (PMSF) at 4℃ for 30 minutes, boiled at 97℃ for 10 min, and then centrifuged for 10 min (13,000 rpm, 4℃). A bicinchoninic acid (BCA) protein assay kit (Thermo Fisher Scientific, Rockford, IL, USA) was utilized to measure the protein concentration in the supernatant. The proteins were resolved and transferred to poly vinylidene fluoride (PVDF) membranes, and Western blotting was performed using antibodies against bcl-2, bax, p-Akt, Akt, p-GSK-3β, GSK-3β, and β-actin (all from Santa Cruz Biotechnology, Santa Cruz, CA, USA). The blots were incubated with horseradish peroxidase-conjugated secondary antibodies for 2 h and developed with an ECL kit (Pierce Biosciences, USA). The bands were visualized using an enhanced chemiluminescence reagent kit (Pierce Biosciences, USA). Immunoreactive bands were visualized by enhanced chemiluminescence, and densitometry was performed using the Quantity One software (Bio-Rad Laboratories, Hercules, CA, USA).
Table 1 presents the metabolic changes observed among the experimental rats. Compared with control rats, diabetic rats had significantly lower body weights (p<0.05 for DM vs. Con; 297.50±4.58 g and 566.30±8.26 g, respectively) and higher blood glucose levels (p<0.05 for DM vs. Con; 33.10±2.12 mmol/L and 6.65±0.56 mmol/L, respectively). The body weights of rats in the DM+IGF-1 group were significantly higher than those of the DM group (p<0.05 for DM+IGF-1 vs. DM; 338.10±3.35 g and 297.50±4.58 g). In contrast, there was no marked difference in blood glucose between the DM and DM+IGF-1 groups. The DM group showed significant increases in TG, TC and urine volume compared to the controls, and these increases significantly were rescued by IGF-1 treatment. These results indicate that IGF-1 at least partially rescues the abnormalities of fat metabolism in DM rats.
H&E staining of cardiac sections from normal control rats showed healthy cardiomyocytes with regular structures, uniform cytoplasmic staining, and uniformly sized nuclei, round or oval shapes, ranking in the central region of the cells (Fig. 1A~I). The DM group, in contrast, showed disordered myocardial cells (Fig. 1A~II) characterized by mitochondrial swelling, vacuolization, and the disappearance of cristae (Fig. 1C~II).
Masson's trichrome staining of control sections revealed that the myocardial collagen fibers were clearly visible, evenly distributed, thin, and lightly stained (Fig. 1B~I). DM rats showed significant myocardial interstitial and vascular fibrosis (Fig. 1B~II). Compared with the control group, collagen volume fraction was increased significantly (p<0.01, DM group vs. Con group) in the DM group. Whereas the myocardial structural damage (Fig. 1C~III) and the collagen volume fraction was significantly reduced (p<0.01, DM+IGF-1 group vs. DM group) and myocardial structural damage (Fig. 1C~III) were significantly improved by IGF-1 treatment. These findings show that IGF-1 treatment mitigates the structural damage to the myocardium of STZ-induced DM rats.
As shown in Fig. 2, the levels of the inflammatory factors, IL-1β and TNF-α, were increased in the DM group compared to the control group (p<0.05 for DM vs. Con; 18.69±0.90 ng/L vs. 12.98±0.94 ng/L and 290.00±9.414 ng/L vs. 146.90±9.13 ng/L respectively), but this was significantly rescued by IGF-1 treatment (p<0.05 for DM+IGF-1 vs. DM; 16.86±0.78 ng/L vs. 18.69±0.90 ng/L and 207.50±9.98 ng/L vs. 290.00±9.41 ng/L respectively).
DM group rats increased accumulation of lipid peroxides, increased MDA expression, and decreased SOD activity, compared to controls (Fig. 3; p<0.05 for DM vs. Con; 14.86±0.71 nmol/mg vs. 7.36±0.49 nmol/mg and 26.48±1.60 U/mg vs. 34.51±1.82 U/mg, respectively). Diabetic rats treated with IGF-1 showed significant rescue of the MDA content and SOD activity compared to the DM group (p<0.05 for DM+IGF-1 vs. DM; 12.28±0.47 nmol/mg vs. 14.86±0.71 nmol/mg and 34.14±0.67 U/mg vs. 26.48±1.60 U/mg, respectively).
TUNEL staining revealed that the DM group clearly exhibited more apoptotic cells than the control group (Fig. 4, AII and B), whereas this apoptosis was significantly ameliorated by IGF-1 treatment (Fig. 4, AIII and B). Western blotting-based assessment of the anti-apoptotic protein, bcl-2, versus the pro-apoptotic protein, bax, showed that their ratio was decreased (indicating the relative enhancement of bax versus bcl-2) in the DM group (Fig. 4C), but that this was rescued by IGF-1 treatment (Fig. 4C; p<0.05 vs. the DM group).
Akt is known to critically modulate glycogen synthesis, cell growth, and cell survival. The activation of GSK-3β, which is a key downstream molecule of Akt, can be induced by Akt phosphorylation. To begin addressing a possible mechanism for the potential cardioprotective effect of IGF-1 in our system, we tested the effects of IGF-1 on the phosphorylation (activation) levels of Akt and GSK-3β. As shown in Fig. 5, the levels of p-Akt and p-GSK-3β were significantly inhibited in the hearts of DM rats versus controls (p<0.05 in both cases), but both of these effects were rescued in the DM+IGF-1 group (p<0.05 vs. the DM group in both cases). These results suggest that IGF-1 activates the Akt-GSK-3β signaling pathway.
In recent years, the roles of IGF-1 in prevention/treatment of diabetes and associated complications are the subject of interest. In the present study, we tested the effects of IGF-1 and assessed underlying mechanisms in the STZ-induced rat model of type 1 DM [18]. We herein report that IGF-1 ameliorates the STZ-induced derangements of ventricular structure and abnormalities in the metabolic parameters and inflammatory, oxidative and fibrosis processes and cell survival with concomitant activation of the Akt-GSK-3β signaling.
DCM, which is defined by ventricular dysfunction, is frequently seen in both humans and animals [19]. Under our experimental conditions, DM rats presented with hyperglycemia and lipid accumulation, and cardiac inflammation, oxidative stress, fibrosis, and apoptosis. Previous studies have shown that hyperglycemia and inactivation of the pro-survival pathways mediated by Akt/GSK-3β may induce myocardial inflammation, endoplasmic reticulum stress, oxidative stress, subsequently promoting myocardial fibrosis, apoptosis, and mitochondrial damage [1720]. IGF-1 is known to critically regulate the complex effects of DM, and decreased IGF-1 signaling is known to decrease natural cardioprotection in DM, leading to DCM [21].
A previous study showed that although myocardial IGF-1 expression decreased in diabetic rats, the addition of exogenous IGF-1 could protect the damaged myocardium and improve myocardial contractility and diastole by regulating myocardial metabolism and inhibiting oxidative stress [22]. Indeed, IGF-1 appears to confer three major cardioprotective actions. First, IGF-1 appears to improve metabolic abnormalities in diabetic rats through its abilities to confer bi-directional regulation of blood glucose levels by promoting peripheral tissue glucose uptake [2324] and inhibiting hepatic production of glucose [2526]. Second, IGF-1 could indirectly inhibit intracardiac RAS activation in diabetic rats, thereby improving left ventricular structure and function by reducing the actions of angiotensin II [27]. Third, IGF-1 activates the Akt/GSK-3β signaling pathway [141522], thereby improving DCM.
The present study shows that IGF-1 improves the characteristics of the diabetic cardiomyopathy, such as increased levels of triglycerides and total cholesterol, inflammatory factors, IL-1β and TNF-α, oxidative parameters, SOD and MDA, apoptotic processes, TUNEL and the ratio of Bcl-2/Bax and left ventricular fibrosis, along with accentuation of the survival Akt-GSK-3β signaling pathway. Diabetes mellitus are characterized by hyperglycemia and show relative or absolute lack of insulin action. Hyperglycemia-, hyperlipidemia- and inflammation-induced oxidative stress is considered to be related to the induction of DCM [2028]. Chronic, but not acute, treatment with insulin normalized blood glucose levels and left ventricular contractile dysfunction in DCM of rats [29]. Exogenous IGF-1 has been shown to reduce blood glucose levels with an increase of its incorporation into tissue glycogen in rats [30]. The IGF-1-induced data shown in the present study may be closely related to its own IGF-1 receptor. Previously, it was shown that high dose of IGF-1 resulted in hypoglycemia even KO-mice for the insulin receptor gene [31].
The Akt signaling controls cellular functions including survival/apoptosis and glucose metabolism [161732]. The substrates of Akt include the Bcl-2 family member Bad. Akt also mediates effect of insulin on glucose metabolism through modulation of glycogen synthase kinase and glucose transporter activity. Previously, it was presented that IGF-1 mediated induction of the PI3K-Akt signaling pathway promotes survival of cultured neuronal cells [13]. This finding suggests that Akt is a critical mediator of IGF-1-induced cell survival (IGF-1-PI3K-Akt signaling). The survival signaling pathway PI3K-Akt activation by IGF-1 phosphorylates the Bcl-2 family member Bad Ser-136 which leads inhibition of cell death and promotes cell survival [33] (IGF-1-PI3K-Akt-Bcl-2 signaling). It was shown that activation of Akt-GSK-3β pathway is involved in the improvements of DCM by attenuation of metabolic disorder, oxidative stress, inflammation, cell death and fibrosis [1634]. This suggests that inactivation of GSK-3β plays a critical role in preventing DCM. (Phosphorylation of the GSK-3β by Akt activation leads the enzyme inactivation). Further, the role of IGF-1-Akt-mTOR signaling may not be excluded in IGF-1-induced effects in diabetic heart. It was previously shown that inhibition of mTORC1, one of the two complexes of mTOR, with endogenous mTORC1 inhibitor proline rich Akt substrate, PRAS40, prevents the development of DCM and improves hepatic insulin sensitivity and reduces hyperglycemia in obese mice fed high fat diet [35]. Also, the PI3KAkt-mTOR pathway was suggested as a part of IGF-1-induced protection against glucose toxicity in cultured rat ventricular cardiomyocytes [36]. These findings suggest that IGF-1 can also improve DCM through the Akt-mTOR signaling pathway.
In summary, the main effects of IGF-1 in our experimental model of DCM were decreases in the observed metabolic abnormalities, myocardial apoptosis, inflammatory cytokine levels, and peroxide infiltration along with an activation of the Akt-GSK-3β signaling pathway. These findings suggest that IGF-1 ameliorates the pathophysiological progress of DCM in the tested model, perhaps by activating Akt/GSK-3β signaling. We therefore suggest that IGF-1 could be considered as a therapeutic candidate for the treatment of DCM.
ACKNOWLEDGEMENTS
This research was supported by the National Natural Science Foundation of China (81160022, 81460056).
Notes
References
1. Kitabchi AE, Umpierrez GE, Miles JM, Fisher JN. Hyperglycemic crises in adult patients with diabetes. Diabetes Care. 2009; 32:1335–1343. PMID: 19564476.


2. Huynh K, McMullen JR, Julius TL, Tan JW, Love JE, Cemerlang N, Kiriazis H, Du XJ, Ritchie RH. Cardiac-specific IGF-1 receptor transgenic expression protects against cardiac fibrosis and diastolic dysfunction in a mouse model of diabetic cardiomyopathy. Diabetes. 2010; 59:1512–1520. PMID: 20215428.


3. Jiang LH, Yuan XL, Yang NY, Ren L, Zhao FM, Luo BX, Bian YY, Xu JY, Lu DX, Zheng YY, Zhang CJ, Diao YM, Xia BM, Chen G. Daucosterol protects neurons against oxygen-glucose deprivation/reperfusion-mediated injury by activating IGF1 signaling pathway. J Steroid Biochem Mol Biol. 2015; 152:45–52. PMID: 25864625.


4. Setshedi M, Longato L, Petersen DR, Ronis M, Chen WC, Wands JR, de la Monte SM. Limited therapeutic effect of N-acetylcysteine on hepatic insulin resistance in an experimental model of alcohol-induced steatohepatitis. Alcohol Clin Exp Res. 2011; 35:2139–2151. PMID: 21790669.


5. Shen Y, Qin J, Bu P. Pathways involved in interleukin-1beta-mediated murine cardiomyocyte apoptosis. Tex Heart Inst J. 2015; 42:109–116. PMID: 25873819.
6. Norby FL, Wold LE, Duan J, Hintz KK, Ren J. IGF-I attenuates diabetes-induced cardiac contractile dysfunction in ventricular myocytes. Am J Physiol Endocrinol Metab. 2002; 283:E658–E666. PMID: 12217882.
7. Han HJ, Kang CW, Park SH. Tissue-specific regulation of insulin-like growth factors and insulin-like growth factor binding proteins in male diabetic rats in vivo and in vitro. Clin Exp Pharmacol Physiol. 2006; 33:1172–1179. PMID: 17184497.


8. Ren J, Duan J, Thomas DP, Yang X, Sreejayan N, Sowers JR, Leri A, Kajstura J, Gao F, Anversa P. IGF-I alleviates diabetes-induced RhoA activation, eNOS uncoupling, and myocardial dysfunction. Am J Physiol Regul Integr Comp Physiol. 2008; 294:R793–R802. PMID: 18199585.


9. Kim MS, Lee DY. Serum insulin-like growth factor-binding protein-3 level correlated with glycemic control and lipid profiles in children and adolescents with type 1 diabetes. J Pediatr Endocrinol Metab. 2014; 27:857–861. PMID: 24825082.


10. Li J, Lin J, Song Y, Xiang L, Wu Z. Effects of insulin-like growth factor-1 on the myocardium in diabetic rats. Zhonghua Yi Xue Za Zhi. 2014; 94:3329–3333. PMID: 25622634.
11. Kim MS, Lee DY. Insulin-like growth factor (IGF)-I and IGF binding proteins axis in diabetes mellitus. Ann Pediatr Endocrinol Metab. 2015; 20:69–73. PMID: 26191509.


12. Kajstura J, Fiordaliso F, Andreoli AM, Li B, Chimenti S, Medow MS, Limana F, Nadal-Ginard B, Leri A, Anversa P. IGF-1 overexpression inhibits the development of diabetic cardiomyopathy and angiotensin II-mediated oxidative stress. Diabetes. 2001; 50:1414–1424. PMID: 11375343.


13. Dudek H, Datta SR, Franke TF, Birnbaum MJ, Yao R, Cooper GM, Segal RA, Kaplan DR, Greenberg ME. Regulation of neuronal survival by the serine-threonine protein kinase Akt. Science. 1997; 275:661–665. PMID: 9005851.


14. Sanchez-Calderon H, Milo M, Leon Y, Varela-Nieto I. A network of growth and transcription factors controls neuronal differentation and survival in the developing ear. Int J Dev Biol. 2007; 51:557–570. PMID: 17891717.


15. Magariños M, Aburto MR, Sánchez-Calderón H, Muñoz-Agudo C, Rapp UR, Varela-Nieto I. RAF kinase activity regulates neuroepithelial cell proliferation and neuronal progenitor cell differentiation during early inner ear development. PLoS One. 2010; 5:e14435. PMID: 21203386.


16. Wang Y, Feng W, Xue W, Tan Y, Hein DW, Li XK, Cai L. Inactivation of GSK-3beta by metallothionein prevents diabetes-related changes in cardiac energy metabolism, inflammation, nitrosative damage, and remodeling. Diabetes. 2009; 58:1391–1402. PMID: 19324938.
17. Yu W, Zha W, Ke Z, Min Q, Li C2, Sun H, Liu C. Curcumin Protects Neonatal Rat Cardiomyocytes against High Glucose-Induced Apoptosis via PI3K/Akt Signalling Pathway. J Diabetes Res. 2016; 2016:4158591. PMID: 26989696.


18. Ganda OP, Rossini AA, Like AA. Studies on streptozotocin diabetes. Diabetes. 1976; 25:595–603. PMID: 132382.


19. Tarquini R, Lazzeri C, Pala L, Rotella CM, Gensini GF. The diabetic cardiomyopathy. Acta Diabetol. 2011; 48:173–181. PMID: 20198391.


20. Liu Q, Wang S, Cai L. Diabetic cardiomyopathy and its mechanisms: Role of oxidative stress and damage. J Diabetes Investig. 2014; 5:623–634.


21. Shan YX, Yang TL, Mestril R, Wang PH. Hsp10 and Hsp60 suppress ubiquitination of insulin-like growth factor-1 receptor and augment insulin-like growth factor-1 receptor signaling in cardiac muscle: implications on decreased myocardial protection in diabetic cardiomyopathy. J Biol Chem. 2003; 278:45492–45498. PMID: 12970367.
22. Yamashita K, Kajstura J, Discher DJ, Wasserlauf BJ, Bishopric NH, Anversa P, Webster KA. Reperfusion-activated Akt kinase prevents apoptosis in transgenic mouse hearts overexpressing insulin-like growth factor-1. Circ Res. 2001; 88:609–614. PMID: 11282895.


23. Russell-Jones DL, Bates AT, Umpleby AM, Hennessy TR, Bowes SB, Hopkins KD, Jackson N, Kelly J, Shojaee-Moradie F, Jones RH, et al. A comparison of the effects of IGF-I and insulin on glucose metabolism, fat metabolism and the cardiovascular system in normal human volunteers. Eur J Clin Invest. 1995; 25:403–411. PMID: 7656918.


24. Katz LE, DeLeón DD, Zhao H, Jawad AF. Free and total insulin-like growth factor (IGF)-I levels decline during fasting: relationships with insulin and IGF-binding protein-1. J Clin Endocrinol Metab. 2002; 87:2978–2983. PMID: 12050283.


25. Luo J, Murphy LJ. Differential expression of the insulin-like growth factor binding proteins in spontaneously diabetic rats. J Mol Endocrinol. 1992; 8:155–163. PMID: 1381181.


26. Lang CH, Vary TC, Frost RA. Acute in vivo elevation of insulin-like growth factor (IGF) binding protein-1 decreases plasma free IGF-I and muscle protein synthesis. Endocrinology. 2003; 144:3922–3933. PMID: 12933666.


27. Groban L, Pailes NA, Bennett CD, Carter CS, Chappell MC, Kitzman DW, Sonntag WE. Growth hormone replacement attenuates diastolic dysfunction and cardiac angiotensin II expression in senescent rats. J Gerontol A Biol Sci Med Sci. 2006; 61:28–35. PMID: 16456192.


28. Giacco F, Brownlee M. Oxidative stress and diabetic complications. Circ Res. 2010; 107:1058–1070. PMID: 21030723.


30. Zapf J, Hauri C, Waldvogel M, Froesch ER. Acute metabolic effects and half-lives of intravenously administered insulinlike growth factors I and II in normal and hypophysectomized rats. J Clin Invest. 1986; 77:1768–1775. PMID: 3711334.


31. Di Cola G, Cool MH, Accili D. Hypoglycemic effect of insulin-like growth factor-1 in mice lacking insulin receptors. J Clin Invest. 1997; 99:2538–2544. PMID: 9153298.


32. Sen P, Mukherjee S, Ray D, Raha S. Involvement of the Akt/PKB signaling pathway with disease processes. Mol Cell Biochem. 2003; 253:241–246. PMID: 14619975.
33. Datta SR, Dudek H, Tao X, Masters S, Fu H, Gotoh Y, Greenberg ME. Akt phosphorylation of BAD couples survival signals to the cell-intrinsic death machinery. Cell. 1997; 91:231–241. PMID: 9346240.


34. Yu W, Wu J, Cai F, Xiang J, Zha W, Fan D, Guo S, Ming Z, Liu C. Curcumin alleviates diabetic cardiomyopathy in experimental diabetic rats. PLoS One. 2012; 7:e52013. PMID: 23251674.


35. Völkers M, Doroudgar S, Nguyen N, Konstandin MH, Quijada P, Din S, Ornelas L, Thuerauf DJ, Gude N, Friedrich K, Herzig S, Glembotski CC, Sussman MA. PRAS40 prevents development of diabetic cardiomyopathy and improves hepatic insulin sensitivity in obesity. EMBO Mol Med. 2014; 6:57–65. PMID: 24408966.


36. Li SY, Fang CX, Aberle NS 2nd, Ren BH, Ceylan-Isik AF, Ren J. Inhibition of PI-3 kinase/Akt/mTOR, but not calcineurin signaling, reverses insulin-like growth factor I-induced protection against glucose toxicity in cardiomyocyte contractile function. J Endocrinol. 2005; 186:491–503. PMID: 16135669.


Fig. 1
IGF-1 attenuated pathological change in the heart of experimental diabetic rats.
(A) Typical pictures of myocardial tissue sections of left ventricular specimens stained with hematoxylin and eosin (magnification=400×). n=8 per group. (B) Typical pictures of myocardial tissue sections stained with masson's trichrome (magnification=400×). Arrows in the figures indicate myocardial interstitial Fibrosis. n=8 per group. (C) Typical transmission electron micrographs (magnification=20000×). Arrows in the figures indicate mitochondrial damage, n=8 per group. (D) Ratio of fibrosis area to total area (%), n=8 per group; Data are mean±SEM. *p<0.05 vs. control group; #p<0.05 vs. DM group.
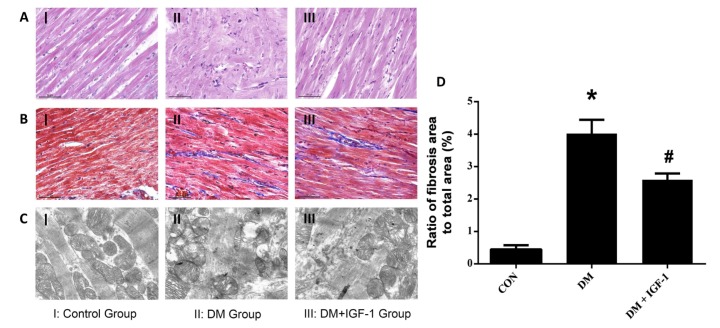
Fig. 2
IGF-1 relieves inflammatory markers in diabetic rats.
(A) Levels of IL-1β. (B) Levels of TNF-α. Values are means±SEM; *p<0.05 vs. control group; #p<0.05 vs. DM group. Number of experiments, n=8 per group.
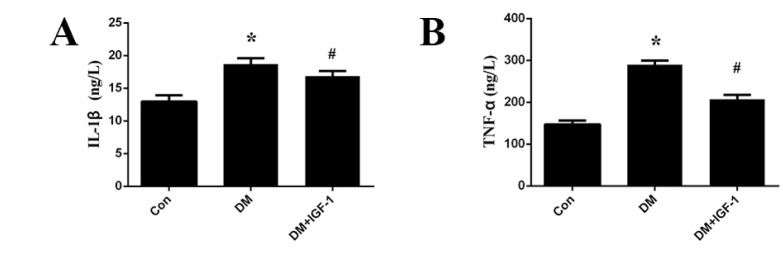
Fig. 3
IGF-1 elevates the activity of superoxide dismutase (SOD) and content of malondialdehyde (MDA) in the heart of experimental diabetic rats.
(A) Activity of SOD. (B) Content of MDA; Data are means±SEM; *p<0.05 vs. control group; #p<0.05 vs. MD group. Number of experiments, n=8 per group.
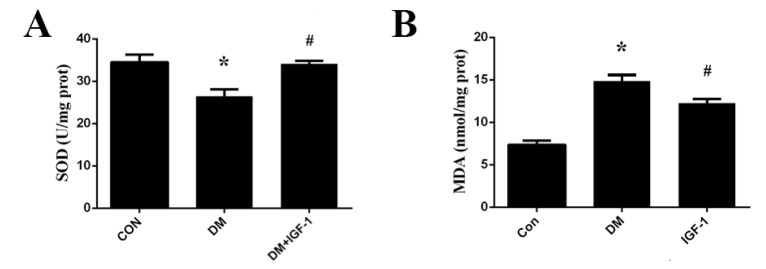
Fig. 4
IGF-1 attenuates apoptosis in the heart of experimental diabetic rats.
(A) Typical pictures of myocardial tissue sections of left ventricular specimens stained with TUNEL (magnification=200×). Number of experiments, n=8 per group. I. Control group; II, DM group; III, DM+IGF-1 group. (B) The mean density of TUNEL stain. Number of experiment, n=8 per group. (C) The expression of Bcl-2 and Bax of experimental rats. Number of experiments, n=8 per group. Values are mean±SD. *p<0.05 vs. control group; #p<0.05 vs. DM group. Con, control group; DM, diabetic group; DM+IGF-1, DM+ IGF-1 group.
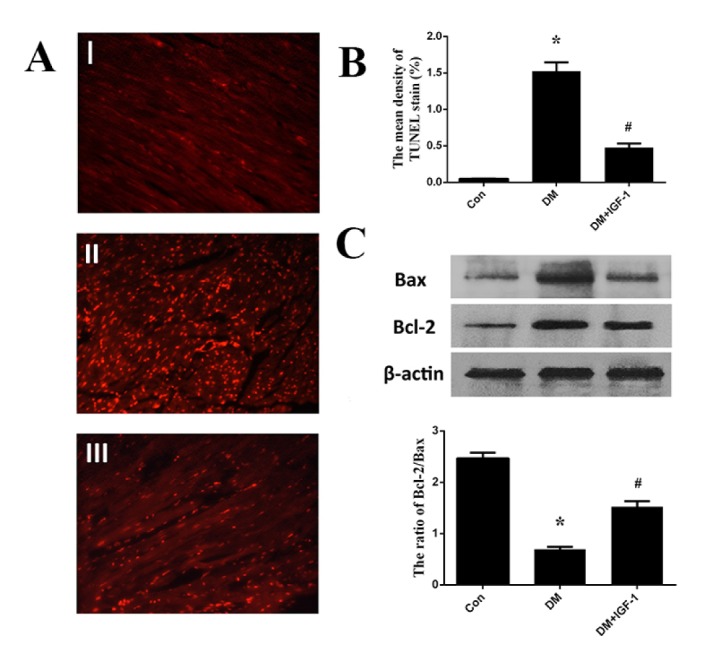
Fig. 5
IGF-1 activates Akt-GSK-3β signaling pathway in the heart of experimental diabetic rats.
(A) p-Akt/Akt. (B) p-GSK-3β/GSK-3β; Values are means±SEM; *p<0.05 vs. control group; #p<0.05 vs. DM group. Number of experiments, n=8 per group.
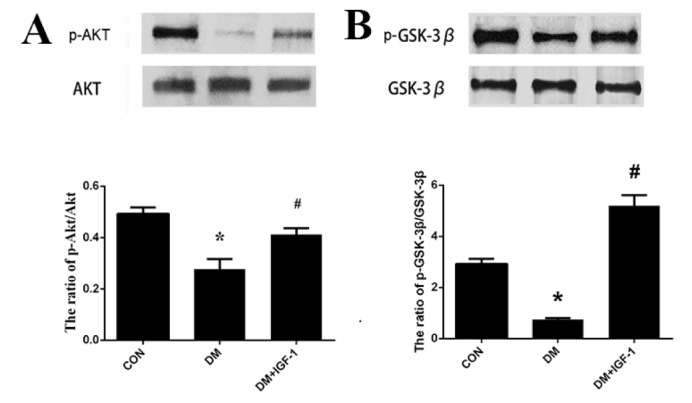
Table 1
IGF-1 prevents metabolic abnormalities in experimental diabetic rats

TG, triglycerides; TC, total cholesterol; Body weight was measured on the day the rats were killed. Blood glucose, urine volume, TG and TC levels were measured in the basal fasting state on the day the rats were killed. Data are means±SEM. n=8 per group; *p<0.05 vs. control group; #p<0.05 vs. DM group.