Abstract
Myometrial relaxation of mouse via expression of two-pore domain acid sensitive (TASK) channels was studied. In our previous report, we suggested that two-pore domain acid-sensing K+ channels (TASK-2) might be one of the candidates for the regulation of uterine circular smooth muscles in mice. In this study, we tried to show the mechanisms of relaxation via TASK-2 channels in marine myometrium. Isometric contraction measurements and patch clamp technique were used to verify TASK conductance in murine myometrium. Western blot and immunehistochemical study under confocal microscopy were used to investigate molecular identity of TASK channel. In this study, we showed that TEA and 4-AP insensitive non-inactivating outward K+ current (NIOK) may be responsible for the quiescence of murine pregnant longitudinal myometrium. The characteristics of NIOK coincided with two-pore domain acid-sensing K+ channels (TASK-2). NIOK in the presence of K+ channel blockers was inhibited further by TASK inhibitors such as quinidine, bupivacaine, lidocaine, and extracellular acidosis. Furthermore, oxytocin and estrogen inhibited NIOK in pregnant myometrium. When compared to non-pregnant myometrium, pregnant myometrium showed stronger inhibition of NIOK by quinidine and increased immunohistochemical expression of TASK-2. Finally, TASK-2 inhibitors induced strong myometrial contraction even in the presence of L-methionine, a known inhibitor of stretch-activated channels in the longitudinal myometrium of mouse. Activation of TASK-2 channels seems to play an essential role for relaxing uterus during pregnancy and it might be one of the alternatives for preventing preterm delivery.
Myometrial contraction is regulated by many factors such as protein kinases and changes in ionic conductance [12]. Myometrial contraction occurs throughout pregnancy. However, its effectiveness is highly increased at term especially by the increase of cell-to-cell communications via high expression of gap junction proteins [3]. Generally, these processes are known to be responsible for coordinated contractility during labor. In fact, myometrial contractility is controlled by many hormones with complicated mechanisms [4]. Normal labor at term associated with the development of maternal acidemia [56] might be due to ischemia [7], which is responsible for the occlusion of blood vessels by strong uterine contractions [8], complicating hormonal effects during pregnancy. Quiescency during pregnancy and organized strong contractions at labor are attributed to increased progesterone [9] and estrogen, respectively [1011].
Potassium channels are ubiquitous in the cellular membranes of mammals. K+ conductance is involved in the maintenance of cell properties such as membrane excitability and cell growth. Usually, negative potentials are produced by the activation of K+ channels that oppose membrane excitability [12131415]. There are many types of K+ channels in smooth muscles such as Ca2+- and voltage-activated K+, and ATP-sensitive K+ channels (KCa, KV, and KATP) which play an important role in regulating the resting membrane potential (RMP) and its excitability [12151617]. Among the many types of K+ channels, the two-pore domain K+ (K2P) channel was identified most recently [181920].
K2P channels can be divided into six subfamilies [20]. One of them, TASK (TWIK (two-pore domain weak inwardly rectifying K+ channel)-related acid-sensing K+ channels) channels have been identified in both electrically excitable and non-excitable tissues [2021]. TASK-1 and 2 channels can be inhibited pharmacologically by several inhibitors [20], and they are very sensitive to changes in extracellular pH [22]. Since myometrium tissue is specifically adapted to stretches, TREK-1 channels activated by membrane stretch are also known to be important in the regulation of myometrial contractility [2022].
In our recent study, we reported that the TASK-2 channel might be one of the candidates for the regulation of uterine circular smooth muscles in mice. TASK-2 inhibitors including extracellular acidosis produced contraction in circular muscle of murine uterus. In addition, contractility and immunohistochemical expression of TASK-2 was significantly increased in pregnant uterus. In this study, we showed that non-inactivating outward K+ current (NIOK) might be responsible for the quiescence of murine pregnant longitudinal myometrium via the activation of TASK-2 channels.
Female non-pregnant ICR (10~12 weeks old) and pregnant ICR mice (19 days) were anaesthetized with isoflurane and sacrificed by cervical dislocation. All experiments were performed in accordance with the guidelines for animal care and use approved by Chungbuk National University. The uteri were cut open from the neck to the end of the uterine horns. Tissues were rinsed in Krebs-Ringer bicarbonate (KRB) solution and then pinned down on a Sylgard plate to maintain their original shape and length. Connective tissue from the uteri was removed and the muscle layers were isolated from the endometrium. For the measurement of mechanical contractions, strips (0.2×1 cm) of longitudinal muscle were mounted in an organ bath (25 ml) of an isometric contractile measuring system. In this system, one end of the tissue was tied tightly to a fixed holder and the other side was linked by a hook type holder to a force transducer (Harvard Apparatus, Holliston, MA, USA). The force transducer was connected to a PowerLab-Data Acquisition System and Charter v5.5 software (ADInstruments, Boulder, CO, USA) with an IBM compatible computer to measure isometric contractions. To rule out nerve-mediated responses, a nerve blocker cocktail (0.4 µM tetrodotoxin (TTX), 1 µM guanethidine, and 1 µM atropine (ATR)) was applied 10 minutes before application of the stimulators (and/or agonists). Each strip was stretched gradually for 1.5~2 hr to achieve resting tension, and then the contractile response was tested by applying a high K+ (50 mM) solution to the strip, which was repeated three or four times until the responses reached a maximum level.
Longitudinal muscle from mice was dissected in Ca2+-free physiological salt solution (Ca2+-free PSS and chopped into small segments (1×2 mm). These segments were incubated in Ca2+-free PSS for 30 min at 4℃. Then, they were incubated in 0.1% papain solution for 15 min and then for 15~25 min again at 35℃ in a digestion medium containing 0.1% collagenase (Wako, Osaka, Japan), 0.05% dithioerythreitol, 0.1% trypsin inhibitor, and 0.2% bovine serum albumin. After digestion, the supernatant was discarded, and the softened muscle segments were transferred to Ca2+-free PSS for 30 min at 4℃. Single cells were then dispersed by gentle agitation using a wide-bore glass pipette. Isolated myometrial cells were maintained in Ca2+-free PSS at 4℃ until use. All experiments were carried out within 4 hr of harvesting the cells and ere performed at room temperature.
Isolated single cells were transferred to a small chamber on the stage of an inverted microscope (IX-70 or IX-71, Olympus, Japan). The chamber was perfused with PSS (2~3 ml/min). Glass pipettes with a resistance of 2~5 MΩ were used to make a giga seal of 5~10 GΩ, by using standard patch clamp techniques [23]. Step depolarizing voltages in 20 mV increments were applied from –100 mV to 80 mV for 450 msec in every 10 sec (Vh=–80 mV). Ramp-depolarizing pulse from –120 mV to +80 mV was also applied for 1900 msec in every 12 sec (Vh=–80 mV). Reversal potential (Erev) of the outward current was evaluated using protocols to determine the reversal of instantaneous tail currents. Membrane currents were elicited in cells by a double-pulse protocol in which membrane potential was depolarized from –80 mV to 0 or 10 mV for 5~7 ms and then stepped to potentials ranging from –30 to +60 mV. Membrane currents were measured after capacitance transients relaxed. Using these protocols, outward current and instantaneous tail currents of murine myometrial cells was recorded and analyzed. Membrane currents were amplified with a 200B patch-clamp amplifier (Axon Instruments, Calif., USA), and the data were digitized with Digidata 1220 or Digidata 1322 and stored directly and digitized on-line using pClamp software (version 5.5.1 or 9.2). Data were displayed on a digital oscilloscope, pen recorder (Harvard Universal Oscillograph, USA; Gould, Cleveland, Ohio, USA) and a computer monitor, and were analyzed using pClamp 6.0 (pClamp 9.2) and Origin software 8.
We used immunoreactivity to visualize cells expressing TASK-2. Single cells from non-pregnant and pregnant tissues were also labeled with the same antibody [23]. For detecting TASK-2 immunoreactivity, cells were incubated for an hour in PSS containing monoclonal antibodies raised against the TASK-2 (diluted 1:200, SantaCruze, Dallas, Texas, U.S.A.). Cells were centrifused (800 rpm, 2 min) and the suspended solution was washed 5 times with PSS. The cells were incubated for an hour in anti-rat IgG antibody solution labeled with a fluorescent marker (goat anti-rat IgG Texas Red, 1:500, Molecular probes, USA). Negative controls were prepared by omitting the primary antibodies or by replacing them with non-immune serum in order to check the specificity of the immunostaining. The tissues and cells labeled with antibodies were examined with a Leica Type TCS SP2 AOBS confocal microscope (Leica Microsystems, Heidelberg GmbH, Germany) under Leica inverted microscope (Leica DMIRE2, Leica, Germany). Optical lens of dry type of 40.0× and NA (0.75) was used (Leica Microsystems, Germany). Confocal microscope captured images with an excitation wave length appropriate for Texas Red (488 nm). Final images were constructed with Leica Confocal Software Ver. 3.0 (Leica Microsystem).
Tissues were fresh-frozen in liquid nitrogen until all time point samples were collected, and were homogenized in homogenation buffer containing 0.01% (v/v) protease inhibitor cocktail (Sigma Chemical Co., St. Louis, MI, U.S.A.). Proteins were measured by a Bradford protein assay (Bio-Rad Laboratories, Richmond, CA) using BSA as the standard. Equal amounts (20~40 µg) of soluble proteins were separated using 8% sodium dodecyl sulfate polyacrylamide gel electrophoresis (SDS–PAGE) at 100 V for 90 min and transferred to a PVDF membrane at 0.25A for 2 hr in a Mini Trans Blot Cell (Bio-Rad, Hercules, CA) at 4℃. The PVDF membranes were blocked with 5% skim-milk in TBS buffer solution (25 mM Tris-HCl (pH7.4) and 150 mM NaCl) overnight at 4℃ with gentle shaking, followed by incubation with TASK-1, 2, and 3 (SantaCruze, Dallas, Texa, U.S.A., 1:500) antibodies diluted in TBS buffer containing 1% skim-milk at room temperature for another 1 hr. After 3 washes with TBS buffer containing 0.1% Tween-20 (TBST), the membranes were incubated with horseradish peroxidase-conjugated secondary antibody (1:5000) diluted in TBS containing 1% skim-milk at room temperature for 1 hr, followed by 3 washes with TBST. Na+/K+-ATPase α (SantaCruze, Dallas, Texa, U.S.A., 1:20000) and Goat anti-Mouse IgG (FC) secondary antibody (Thermo Scientific, 1:20000) were also used. To detect the reaction, membranes were further treated with ECL(ELPIS) reagent for 1 min and subsequently imaged using an Lass 3000 (FUJIFILM).
Ca2+ PSS contained the followings (in mM): NaCl 140, KCl 4.5, CaCl2 2, MgCl2 1, glucose 10, and HEPES (N-[2-hydroxyethyl] piperazine-N'-[2-ethanesulphonic acid]) 10, and its pH was adjusted to 7.4 with NaOH. Equimolar concentrations of external Na+ were replaced with K+ to make high K+ solution. Ca2+ was simply omitted from Ca2+ PSS for Ca2+-free PSS solution. For whole-cell path clamp technique, the pipette solution containing (in mM) 140 KCl, 5 MgCl2, 0.1 Na2GTP, 2.5 creatine phosphate (disodium salt), 5 HEPES and 11 EGTA was used adjusted to pH 7.2 by Tris. Phosphate-added cold Tyrode's solution contained (in mM) NaCl 145, KCl 5, MgCl2 2, CaCl2 2, glucose 10, NaH2PO4 0.42, Na2HPO4 1.81, and HEPES 10, adjusted to pH 7.4. KRB solution (CO2/bicarbonate-buffered Tyrode) contained (in mM) NaCl 122, KCl 4.7, MgCl2 1, CaCl2 2, NaHCO3 15, KH2PO4 0.93, and glucose 11 (pH 7.3~7.4, bubbled with 5% CO2/95% O2). The membrane impermeable pH buffer, 2-N-morpholinoethanesulphonic acid (MES, Sigma Chem Co., St Louis, MO, USA) was used to adjust the KRB solution to pH 6.4 when necessary. Equimolar concentrations of external Na+ were replaced by K+ for 50 mM K+ solution. The external solution was changed to the next solution already pre-incubated (bubbled with 5% CO2/95% O2, 36℃) in water bath before application. All drugs used in this study were purchased from Sigma.
Data were expressed as means±standard errors of the mean (means±SEM). The Student's t-test (paired and unpaired) was used wherever appropriate to evaluate differences in data. Wilcoxon rank-sum test and Mann-Whitney test was also used in molecular experiments. p values less than 0.05 were regarded as statistically significant.
OXT treatment produced tonic contractions superimposed with phasic contractions. Initial peak contractions induced by OXT were 1.5±0.18 g (non-pregnant, n=48, 38) and 2.1±0.20 g (pregnant, n=32, 38), respectively. Tonic contractions were 0.5±0.07 g (non-pregnant, n=48, 46) and 2.0±0.20 g (pregnant, n=32, 38) (Fig. 1A). Tonic contractions induced by OXT were significantly different in non-pregnant and pregnant myometrial tissues.
The effect of quinidine, an inhibitor of the K2P channel, on myometrium was studied in uterine longitudinal muscle. As shown in Fig. 1B, quinidine (10, 20, and 30 µM) provoked contractions and increased their frequencies in a concentration-dependent manner in pregnant and non-pregnant myometrial preparations (Fig. 1B, 1C). At high concentrations, quinidine-induced contractions began to merge with tonic contractions, and this tendency was stronger in pregnant myometrium. At a concentration of 50 µM, quinidine significantly increased the magnitude of tonic contractions (0.15±0.05 g, non-pregnant; 0.4±0.09 g, pregnant; data not shown).
We also examined the effect of quinidine on myometrial contractility in the presence of tetraethylammonium (TEA) and 4-aminopyridine (4-AP), which are known inhibitors of KCa and KV channels, respectively. As shown in Fig. 1C, TEA (10 mM) produced strong phasic contractions in non-pregnant myometrium (1.2±0.15 g) but did not produce contractions in pregnant tissue. The magnitude of quinidine-induced contractions was compared in the presence of 10 mM TEA. Quinidine (10, 20, and 30 µM) produced much larger phasic contractions in pregnant tissue than in non-pregnant tissue (0.9±0.16, 0.8±0.13, 0.2±0.05 g, non-pregnant; 1.5±0.23, 1.6±0.27, 1.1±0.18 g, pregnant; Fig. 1C). Quinidine elicited contractions in the presence of nerve blockers (see Methods) (data not shown). Thus, these contractions were not mediated by neurotransmitters.
Because quinidine is a potent inhibitor of two-pore domain channels (TASK; IC50=22 µM for TASK-2 of expressed cell) [24], we also studied the effects of other TASK channel inhibitors such as lidocaine (1 mM), bupivacaine (100 nM), and [pH]o=6.4. All of these TASK channel inhibitors increased contractility in pregnant longitudinal myometrium (Fig. 2) [21]. To rule out the involvement of other K+ channels, TEA (10 mM) and 4-AP (5 mM) was also used. TASK channels in pregnant myometrium were inhibited with bupivacaine and [pH]o=6.4 in the presence of TEA (10 mM) and 4-AP (5 mM). As shown in Fig. 2A and 2D, [pH]o=6.4 and bupivacaine provoked contractions in the presence of TEA and 4-AP. [pH]o=6.4 and bupivacaine also provoked contractions in the presence of nerve blockers as well as TEA and 4-AP (Fig. 2B and 2D).
The increased expression and activation of stretch-dependent K2P channels (TREK-1) has been reported in pregnant myometrium [182526]. Therefore, to rule out the involvement of TREK-1, we studied the effect of TASK-2 in the presence of L-methionine, a TREK-1 inhibitor, on pregnant longitudinal myometrial contractility (Fig. 2C and 2E). L-methionine (1 mM) produced transient contraction (0.8±0.20 g, n=11), which decayed to a low level (0.2±0.12 g, n=11) within 10~15 min (Fig. 2C and 2E). Further robust contractions were produced in the presence of L-methionine, bupivacaine, lidocaine, and quinidine. Bupivacaine (100 and 500 nM) and lidocaine (1 mM) produced contractions of 1.2±0.21 g (n=8), 1.2±0.25 g (n=10), and 1.1±0.24 g (n=10), respectively. Quinidine (20 µM) produced phasic contractions of 1.2±0.34 g (n=9), and 50 µM produced phasic contractions (0.9±0.19 g, n=10) superimposed on tonic contractions (0.5±0.15 g, n=9) (Fig. 2E). Among these inhibitors, quinidine produced tonic contractions in pregnant myometrium. The observation that TASK-2 inhibitors produced strong contractions even in the presence of L-methionine, strongly suggests that TASK-2 activity increases during pregnancy due to the increase of its expression and/or functional activity and that it might play a role in stabilizing membrane excitability, hence, facilitating relaxation during pregnancy and contraction at parturition.
We studied outward currents from single isolated murine longitudinal myometrial cells. Non-inactivating outward K+ currents (NIOK) in pregnant cells using a step depolarizing pulse (see Methods) were inhibited to 43.6±3.50% by TEA (n=5) and to 41.8±5.17% by 4-AP plus TEA (n=5) at +80 mV (data not shown). The remaining current, which coincides with TEA and 4-AP resistant current (TEA and 4-AP insensitive non-inactivating outward K+ current (NIOK)), is shown in the left panel of Fig. 3A. The reversal potentials (Erev) of TEA and 4-AP resistant currents were briefly evaluated by changing the external K+ concentration from 5 to 30 mM (see Methods ; Fig. 4). Erev shifted to new expected values at 30 mM of external K+. Using these protocols, instantaneous tail currents of outward current in non-pregnant and pregnant myometrial cells were reversed at –36.8±7.04 mV (from –60.6±2.17 mV; n=3) and –40.6±4.50 mV (from –64.6±4.14 mV; n=5) by 30 mM K+. Therefore, this result suggests that outward current remained after treatment with K+ channel blockers and was a Ca2+-insensitive NIOK current. The characteristic of Ca2+-insensitivity of NIOK is responsible for independence of intra- and extracellular Ca2+ including the block of KCa channels by TEA in experimental condition (see methods).
In the next step, we studied NIOK currents using pharmacological blockers of the TASK-2 channel. As shown in Fig. 3A, 3B, quinidine (50 µM) inhibited the current to 61±9.2% of the control (n=5) at +80 mV in non-pregnant cells. The corresponding inhibition in pregnant cells was to 35±5.4% of the control (n=5). Thus, inhibitory potency was significantly greater in pregnant cells than in non-pregnant cells (Fig. 3B). Because the TASK channel was inhibited by decreased pHo and bupivacaine, we also studied the effect of pHo and bupivacaine on NIOK currents. As shown in Fig. 3C, pHo (pHo=6.4) and bupivacaine (500 nM) inhibited NIOK. NIOK was inhibited to 86±1.57% and 81±2.86% in pregnant cells at +80 mV (n=5 and 6; p<0.05) (Fig. 3C and 3E). Meanwhile, extracellular alkalosis (pHo=8.4) increased NIOK to 126±9.69% in pregnant cells (n=5, p<0.05). These inhibitory effects are summarized in the current/voltage (I/V) relationship (Fig. 3D). In addition, Ba2+ (3 mM) also inhibited NIOK currents to 82±6.41% of the control (n=3; Fig. 3E) [24].
Development of the pregnant myometrium and parturition is regulated by sex hormones. Therefore, understanding the mechanism of parturition in terms of ion channels such as TASK-2 is very promising for managing labor at term and developing target medicines. As shown in Fig. 3C~3E, OXT (10 and 50 nM) inhibited the NIOK current to 87±1.94% and 85±2.93% in pregnant cells in a reversible manner, respectively (p<0.05). Estrogen (1 µM) also inhibited the NIOK current to 68±7.65% (n=4; p<0.05).
The electrophysiology and mechanical findings led us to study TASK-2 channel expression. Confocal images at 2 µm intervals showed localization of the TASK-2 channel on the cell membrane of pregnant myometrial cells (Fig. 5A). As shown in Fig. 5B, we found highly increased immunoreactivity for the TASK-2 antibody in single pregnant myometrial cells compared to that in non-pregnant cells by confocal microscopy. The increased expression of TASK-2 in pregnancy compared to the control was also observed by Western blot (595±243.88 vs 100±19.61, p<0.05; n=3 and 4) (Fig. 5C). Although data not shown, TASK-1 was also expressed in mouse but not significantly different in two groups (data not shown). Meanwhile, TASK-3 was not expressed in murine myometrium under Western blot.
K2P channels are regulated by various factors such as extracellular pH [22] and mechanical stretch [27]. The pharmacological characteristics of TASK-2, one of the K2P channels, are inhibited by quinidine, bupivacaine, lidocaine, and extracellular acidosis [20]. Our experiment showed similar results and also showed increased TASK-2 expression by immunohistochemistry and Western blot during pregnancy. Furthermore, TASK-2 had functional characteristics directly controlled by OXT and estrogen, which increase at delivery. This is the first study proposing sex hormone and extracellular pH-sensitive K+ conductance as key regulators of pregnant myometrium.
As shown in Fig. 3A and 3B, quinidine inhibited TEA and 4-AP insensitive NIOK in murine myometrium. The inhibitory effect of quinidine on NIOK was more potent in pregnant myometrial cells compared to that in non-pregnant cells. NIOK in the presence of TEA and 4-AP was inhibited by quinidine to 61% in non-pregnant cells and to 35% in pregnant cells. Quinidine potently inhibited TASK-2 and TRESK-2 channels. Quinidine (100 µM) inhibits TRESK-2 and TASK-2 channels completely and by 65% in transfected cells, respectively [2428].
However, we found that TRESK-2 was not expressed in murine pregnant myometrium (data not shown). Therefore, TASK-2 activity not TRESK-2 in the pregnant myometrium much more increased compared to that from non-pregnant tissue. In addition, NIOK was inhibited by bupivacaine, extracellular acidosis, and sex hormones such as estrogen (Fig. 3C~3E), which are key hormones for producing myometrial contractions at parturition. Estrogen including OXT regulate K+ channels via the activation of second messengers [2930] and/or the open channel block mechanism [31]. Barium also inhibits TASK-2 but has no significant effect on TASK-1 [2024]. We found an inhibitory effect of barium (3 mM) on NIOK in murine myometrium (82±6.41% of the control; n=3; Fig. 3E). In 2011, however, inhibitory effect of external barium on TREK-1 was reported. Therefore, it should be careful interpretation too [32]. Even though, we did not insert data, we also found increasing effect of progesterone on NIOK in 3 cells from 5 tested cells. Furthermore, pregnant myometrium showed increased TASK-2 expression by immunohistochemistry (Fig. 5B). Western blot also revealed that the expression of TASK-2 channel protein was increased in mice and was particularly strong in pregnant myometrium (595±243.88 vs 100±19.61, p<0.05; n=3 and 4) (Fig. 5C). Therefore, increased TASK-2 expression is thought to play an important role in pregnant myometrium, which relaxes during the gestation period and contracts at parturition.
Generally, it is hard to separate the effect of [pH]o from [pH]i since the internal and external environments interact with each other [3334]. Since plasma pH tends to be altered during pregnancy and at labor [5635], the change of pH is regarded as one of the targets for regulation of myometrial contractility. Our data indicated that the relaxation of murine longitudinal uterine muscle was produced by the activation of acid-sensitive TASK-2 channels during pregnancy. Especially, we found that decreased pHo enhanced myometrial contraction combined with decreased NIOK in murine myometrium. It was also reported that decreased pHo abolished phasic contractions in humans [3637]. Meanwhile, increased pHo produces relaxation by inhibiting voltage-dependent L-type Ca2+ channels (VDCCL) [34].
However, pregnant myometrium is kept quiescent by increased progesterone levels [9] and the inhibition is lifted by increased estrogen from mid-gestation to birth to coordinate contractions at labor [1011]. Progesterone decreases the expression level of the myometrial OXT receptor (OXTR) indirectly [38]. Thus, progesterone withdrawal [39] induces estrogen activation at term, which initiates parturition by transforming the myometrium into a highly organized contractile state. Because NIOK was inhibited (Fig. 3C~3E) by estrogen during pregnancy, estrogen seems to have a dual effect in regulating myometrial contractility.
Myometrial contractile responses in mammals are generated from changes in ionic conductance [12]. Although KCa and KV channels are ubiquitous in smooth muscle and important for the regulation of its excitability, K2P channels are also known to contribute to the RMP [1]. As activation of K+ channels suppresses membrane excitability in vessels [1317] as well as the myometrium at labor [16,404142], we examined the effect of TASK-2 inhibitors on myometrial contractility in the presence of TEA and 4-AP. In accordance with previous reports showing that most K2P channels are insensitive to TEA and 4-AP [20], application of TEA and/or 4-AP produced no contractions except for weak transient contractions in some pregnant muscle cells (Figs. 1C, 2A~2C) [30]. As shown in Fig. 2, TASK-2 inhibitors such as quinidine, acidosis, bupivacaine, and lidocaine produced robust contractions even in the presence of TEA, 4-AP, and/or L-methionine. This result suggests that TASK-2 might play a central role in the regulation of myometrial contractility in longitudinal muscle. In our previous study, we reported that the TASK-2 channel might be one of the targets regulating the murine circular myometrium [21].
TASK-1 is also known to be a [pH]o-sensitive K2P channel [20]. Although TASK-1 is not sensitive to quinidine, it was also observed in murine myometrium under Western blot. Since TASK channels are sensitive to the alteration of external pH, TASK-1 including TASK-2 channels could be targets for myometrial relaxation. Nevertheless, we believe that our electrophysiological results imply that TASK-2 is much more strongly linked to the physiological regulation of murine myometrial contractility than TASK-1. Even though the data is not presented here, we also found TASK-1 and TASK-2 channel expression in human myometrium (data not shown). Up to date, only very weaker and variable expression of TASK-2 channels compared to other K2P channel of human myometrium was reported under RT-PCR study [18]. However, we also found TASK-2 channel expression of human myometrium and have evidence of enhanced expression of TASK-2 channel expression in pregnant tissues compared to that from non-pregnant tissues of human in Western blot. In addition, we also studied the expression TASK-3 since its characteristic is also similar to that of TASK-2. In murine uterus, however, it was not expressed in Western Blot. In addition, we also studied the expression of other kinds of K2P channels such as such as TRESK, and TREK-2 under PCR. Its expression was decreased in pregnant myometrium compared to non-pregnant myometrium (data not shown). Therefore, we need to elucidate the differences in the physiological function of humans and mice including the expression patterns of TASK-1 and TASK-2 channels in the future.
Uterine longitudinal muscles showed spontaneous contractions in non-pregnant (1.5±0.10 g, 0.7±0.05 cycles/min; n=53) and pregnant myometrium (1.8±0.18 g, 0.7±0.09 cycles/min; n=18, 17). However, the contractions were abolished with stretching (see Methods) in both tissues (data not shown). This phenomenon can be explained by TREK-1. The increased expression and function of stretch-dependent K2P channels (TREK-1) has been reported in the myometrium during pregnancy [182526]. In addition to the role of stretch activated K2P channels [2526], this is the first report relating the conductance of [pH]o-sensitive TASK-2 to a functional mechanism of relaxation during pregnancy. We noted strong contractions using TASK-2 inhibitors in the presence of TREK-1 inhibitors (Fig. 2C and 2E). Therefore, the increased expression of TASK-2 might also be a reasonable explanation for uterine accommodation of the growing fetus during pregnancy rather than the increase in fetus-induced passive response to stretch-mediated channels.
ACKNOWLEDGEMENTS
Kyu-Sang Kyeong, Seung Hwa Hong, Young Chul Kim equally contributed to this work. This work was supported by the research grant of the Chungbuk National University in 2014.
Notes
Author contributions: K.S.K., S.H.W., Y.C.K., R.Y.Y. and H.S. performed tissue & cell experiment. K.S.K., Y.C.K., W.C., S.C.M., M.Y.L., R.Y.Y., C.H.K., Y.J.P., H.K. and I.W.J performed molecular and histochemical study. Y.C.K., S.Y.K., H.S., E.H.J., H.S.K., S.W.L., S.J.L. and I.W.J. performed clinical and pharmacological assistance and wrote paper.
References
1. Parkington HC, Coleman HA. Excitability in uterine smooth muscle. Front Horm Res. 2001; 27:179–200. PMID: 11450426.


2. Sanborn BM. Ion channels and the control of myometrial electrical activity. Semin Perinatol. 1995; 19:31–34. PMID: 7538700.


3. Garfield RE, Sims S, Daniel EE. Gap junctions: their presence and necessity in myometrium during parturition. Science. 1977; 198:958–960. PMID: 929182.


4. Wray S, Noble K. Sex hormones and excitation-contraction coupling in the uterus: the effects of oestrous and hormones. J Neuroendocrinol. 2008; 20:451–461. PMID: 18266942.


5. Cerri V, Tarantini M, Zuliani G, Schena V, Redaelli C, Nicolini U. Intravenous glucose infusion in labor does not affect maternal and fetal acid-base balance. J Matern Fetal Med. 2000; 9:204–208. PMID: 11048829.


6. Sjostedt S. Acid-base balance of arterial blood during pregnancy, at delivery, and in the puerperium. Am J Obstet Gynecol. 1962; 84:775–779. PMID: 13913556.
7. Harrison N, Larcombe-McDouall JB, Earley L, Wray S. An in vivo study of the effects of ischaemia on uterine contraction, intracellular pH and metabolites in the rat. J Physiol. 1994; 476:349–354. PMID: 8046648.


8. Brinkman CR. Circulation in the pregnant uterus. In : Carsten ME, Miller JD, editors. Uterine function: molecular and cellular aspects. New York: Plenum Press;1990. p. 519–537.
10. Tulchinsky D, Hobel CJ, Yeager E, Marshall JR. Plasma estrone, estradiol, estriol, progesterone, and 17-hydroxyprogesterone in human pregnancy. I. Normal pregnancy. Am J Obstet Gynecol. 1972; 112:1095–1100. PMID: 5025870.
11. Walsh SW, Stanczyk FZ, Novy MJ. Daily hormonal changes in the maternal, fetal, and amniotic fluid compartments before parturition in a primate species. J Clin Endocrinol Metab. 1984; 58:629–639. PMID: 6230368.


12. Hong SH, Kyeong KS, Kim CH, Kim YC, Choi W, Yoo RY, Kim HS, Park YJ, Ji IW, Jeong EH, Kim HS, Xu WX, Lee SJ. Regulation of myometrial contraction by ATP-sensitive potassium (KATP) channel via activation of SUR2B and Kir 6.2 in mouse. J Vet Med Sci. 2016; 78:1153–1159. PMID: 27086859.
13. Park JK, Kim YC, Sim JH, Choi MY, Choi W, Hwang KK, Cho MC, Kim KW, Lim SW, Lee SJ. Regulation of membrane excitability by intracellular pH (pHi) changers through Ca2+-activated K+ current (BK channel) in single smooth muscle cells from rabbit basilar artery. Pflugers Arch. 2007; 454:307–319. PMID: 17285302.
14. Smith RC, McClure MC, Smith MA, Abel PW, Bradley ME. The role of voltage-gated potassium channels in the regulation of mouse uterine contractility. Reprod Biol Endocrinol. 2007; 5:41–52. PMID: 17980032.


15. Wray S, Burdyga T, Noble D, Noble K, Borysova L, Arrowsmith S. Progress in understanding electro-mechanical signalling in the myometrium. Acta Physiol (Oxf). 2015; 213:417–431. PMID: 25439280.


16. Anwer K, Oberti C, Perez GJ, Perez-Reyes N, McDougall JK, Monga M, Sanborn BM, Stefani E, Toro L. Calcium-activated K+ channels as modulators of human myometrial contractile activity. Am J Physiol. 1993; 265:C976–C985. PMID: 8238323.
17. Brayden JE, Nelson MT. Regulation of arterial tone by activation of calcium-dependent potassium channels. Science. 1992; 256:532–535. PMID: 1373909.


18. Bai X, Bugg GJ, Greenwood SL, Glazier JD, Sibley CP, Baker PN, Taggart MJ, Fyfe GK. Expression of TASK and TREK, two-pore domain K+ channels, in human myometrium. Reproduction. 2005; 129:525–530. PMID: 15798028.
19. Ketchum KA, Joiner WJ, Sellers AJ, Kaczmarek LK, Goldstein SA. A new family of outwardly rectifying potassium channel proteins with two pore domains in tandem. Nature. 1995; 376:690–695. PMID: 7651518.


20. O'Connell AD, Morton MJ, Hunter M. Two-pore domain K+ channels-molecular sensors. Biochim Biophys Acta. 2002; 1566:152–161. PMID: 12421546.
21. Hong SH, Sung R, Kim YC, Suzuki H, Choi W, Park YJ, Ji IW, Kim CH, Myung SC, Lee MY, Kang TM, You RY, Lee KJ, Lim SW, Yun HY, Song YJ, Xu WX, Kim HS, Lee SJ. Mechanism of relaxation via TASK-2 channels in uterine circular muscle of mouse. Korean J Physiol Pharmacol. 2013; 17:359–365. PMID: 23946696.


22. Duprat F, Lesage F, Fink M, Reyes R, Heurteaux C, Lazdunski M. TASK, a human background K+ channel to sense external pH variations near physiological pH. EMBO J. 1997; 16:5464–5471. PMID: 9312005.
23. Kim YC, Suzuki H, Xu WX, Hashitani H, Choi W, Yun HY, Park SM, Youn SJ, Lee SJ, Lee SJ. Voltage-dependent Ca2+ current identified in freshly isolated interstitial clls of cajal (ICC) of Guineapig stomach. Korean J Physiol Pharmacol. 2008; 12:323–330. PMID: 19967074.
24. Reyes R, Duprat F, Lesage F, Fink M, Salinas M, Farman N, Lazdunski M. Cloning and expression of a novel pH-sensitive two pore domain K+ channel from human kidney. J Biol Chem. 1998; 273:30863–30869. PMID: 9812978.
25. Buxton IL, Singer CA, Tichenor JN. Expression of stretch-activated two-pore potassium channels in human myometrium in pregnancy and labor. PLoS One. 2010; 5:e12372. PMID: 20811500.


26. Monaghan K, Baker SA, Dwyer L, Hatton WC, Sik Park K, Sanders KM, Koh SD. The stretch-dependent potassium channel TREK-1 and its function in murine myometrium. J Physiol. 2011; 589:1221–1233. PMID: 21224218.


27. Miller P, Kemp PJ, Lewis A, Chapman CG, Meadows HJ, Peers C. Acute hypoxia occludes hTREK-1 modulation: re-evaluation of the potential role of tandem P domain K+ channels in central neuroprotection. J Physiol. 2003; 548:31–37. PMID: 12611912.
28. Kang D, Mariash E, Kim D. Functional expression of TRESK-2, a new member of the tandem-pore K+ channel family. J Biol Chem. 2004; 279:28063–28070. PMID: 15123670.
29. Che T, Sun H, Li J, Yu X, Zhu D, Xue B, Liu K, Zhang M, Kunze W, Liu C. Oxytocin hyperpolarizes cultured duodenum myenteric intrinsic primary afferent neurons by opening BK(Ca) channels through IP3 pathway. J Neurochem. 2012; 121:516–525. PMID: 22356163.
30. Soriano S, Ripoll C, Fuentes E, Gonzalez A, Alonso-Magdalena P, Ropero AB, Quesada I, Nadal A. Regulation of K(ATP) channel by 17β-estradiol in pancreatic β-cells. Steroids. 2011; 76:856–860. PMID: 21470558.


31. Druzin M, Malinina E, Grimsholm O, Johansson S. Mechanism of estradiol-induced block of voltage-gated K+ currents in rat medial preoptic neurons. PLoS One. 2011; 6:e20213. PMID: 21625454.
32. Ma XY, Yu JM, Zhang SZ, Liu XY, Wu BH, Wei XL, Yan JQ, Sun HL, Yan HT, Zheng JQ. External Ba2+ block of the two-pore domain potassium channel TREK-1 defines conformational transition in its selectivity filter. J Biol Chem. 2011; 286:39813–39822. PMID: 21965685.
33. Naderali EK, Wray S. Modulation of force induced by pH in the guinea-pig uterus examined at two stages of the oestrous cycle. J Reprod Fertil. 1999; 117:153–157. PMID: 10645256.


34. Taggart MJ, Sheader EA, Walker SD, Naderali EK, Moore S, Wray S. External alkalinization decreases intracellular Ca++ and spontaneous contractions in pregnant rat myometrium. Am J Obstet Gynecol. 1997; 177:959–963. PMID: 9369852.


35. Rooth G, Sjostedt S. The placental transfer of gases and fixed acids. Arch Dis Child. 1962; 37:366–370. PMID: 14493734.


36. Parratt JR, Taggart MJ, Wray S. Functional effects of intracellular pH alteration in the human uterus: simultaneous measurements of pH and force. J Reprod Fertil. 1995; 105:71–75. PMID: 7490717.


37. Taggart M, Wray S. Simultaneous measurement of intracellular pH and contraction in uterine smooth muscle. Pflugers Arch. 1993; 423:527–529. PMID: 8351202.


38. Soloff MS, Fernstrom MA, Periyasamy S, Soloff S, Baldwin S, Wieder M. Regulation of oxytocin receptor concentration in rat uterine explants by estrogen and progesterone. Can J Biochem Cell Biol. 1983; 61:625–630. PMID: 6313158.


39. Mesiano S, Welsh TN. Steroid hormone control of myometrial contractility and parturition. Semin Cell Dev Biol. 2007; 18:321–333. PMID: 17613262.


40. Khan RN, Matharoo-Ball B, Arulkumaran S, Ashford ML. Potassium channels in the human myometrium. Exp Physiol. 2001; 86:255–264. PMID: 11429642.


41. Khan RN, Smith SK, Morrison JJ, Ashford ML. Ca2+ dependence and pharmacology of large-conductance K+ channels in nonlabor and labor human uterine myocytes. Am J Physiol. 1997; 273:C1721–C1731. PMID: 9374660.
Fig. 1
Effect of quinidine on the isometric contraction of uterine longitudinal muscle in mice.
(A) Oxytocin (OXT) produced initial and tonic contractions; however, tonic contractions were not sustained in non-pregnant myometrium (NP, non-pregnant; TP, term-pregnant). (B) Quinidine (10, 20, and 30 µM) produced concentration-dependent phasic contractions. Tonic contractions were also produced at high concentrations of quinidine in pregnant myometrium. (C) Quinidine was applied to myometrium in the presence of TEA (10 mM). TEA (10 mM) produced strong phasic contractions in non-pregnant myometrium (1.2±0.15 g) but not in pregnant myometrium. Quinidine (10~30 µM), even in the presence of TEA, increased contraction frequency in non-pregnant myometrium and produced stronger contractions in pregnant myometrium.

Fig. 2
Regulation of pregnant mouse myometrial contractility by TASK-2 channel inhibitors in the presence of TEA and 4-AP.
The TASK-2 inhibitors produced contractions in pregnant myometrium in the presence of TEA and 4-AP. (A) Extracellular acidosiso (pHo=6.4) produced contractions in pregnant myometrium. (B) Bupivacaine (100 nM) also produced robust contractions even in the presence of nerve blockers. (C) L-methionine (1 mM), which inhibits stretch-dependent K2P channels (TREK-1), produced contractions that spontaneously decayed to near baseline values within 10~15 min. However, lidocaine produced robust contraction in the presence of L-methionine. (D~E) Data are summarized.

Fig. 3
Regulation of TEA and 4-AP insensitive non-inactivating outward current (NIOK) in single mouse myometrial cells.
(A, B) In the presence of TEA (10 mM) and 4-AP (5 mM), NIOK were observed under whole-cell voltage clamp. Quinidine (50 µM) inhibited NIOK currents more significantly in pregnant cells. (C) NIOK was inhibited by pHo=6.4, bupivacaine, oxytocin (OXT), and estrogen in pregnant myometrial cells. (D) Representative I/V relationships are shown. (E) Data are summarized. NIOK was inhibited to 126, 86, 87, 85, 81, 68 and 78% of the control by pHo=8.4, pHo=6.4, OXT (10 and 50 nM), bupivacaine (500 nM), estrogen (1 µM), and Ba2+ (3 mM), respectively (n= 5, 5, 4, 3, 6, 4 and 3, respectively; *p<0.05). Such as TASK-2 is very promising for managing labor at term and developing target medicines. As shown in Fig. 3C~3E, OXT (10 and 50 nM) inhibited the NIOK current to 87±1.94% and 85±2.93% in pregnant cells in a reversible manner, respectively (p<0.05). Estrogen (1 µM) also inhibited the NIOK current to 68±7.65% (n=4; p<0.05).
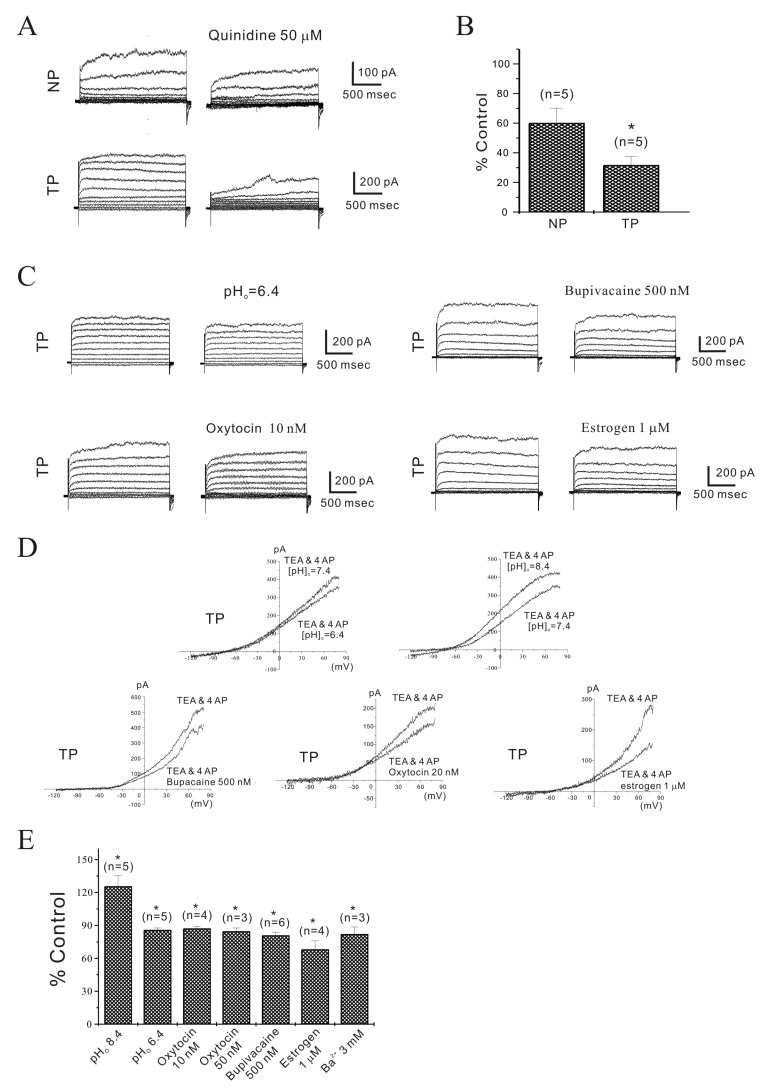
Fig. 4
Identification of NIOK by reversal potentials (Erev).
The reversal potentials (Erev) of NIOK were evaluated by changing the external K+ concentration from 4.5 to 30 mM. Erev shifted to new expected values at 30 mM of external K+. Instantaneous tail currents in the NIOK of non-pregnant and pregnant myometrial cells were reversed at –37 mV (n=3) and –41 mV (n=5) by 30 mM K+.
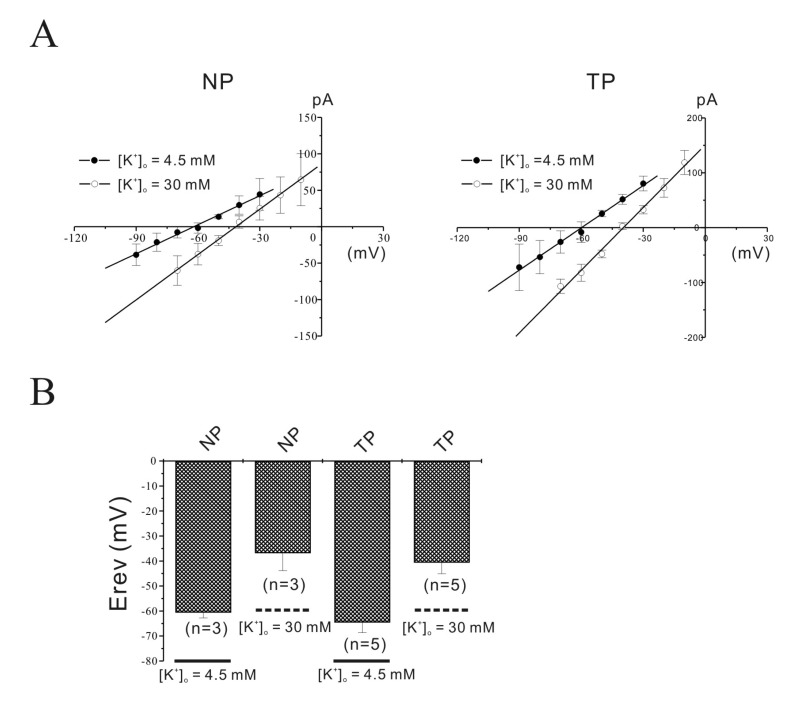
Fig. 5
Identification of TASK-2 by immunohistochemistry and Western blot in mouse myometrium.
(A, B) Laser scanning confocal micrograph shows TASK-2 immunoreactivity on the membrane of a single pregnant cell. Confocal images at 2 µm intervals showed localization of the TASK-2 channel on the cell membrane of pregnant myometrial cells. Highly increased immunoreactivity for the TASK-2 antibody in single pregnant myometrial cells ws observed compared to that in non-pregnant cells. (C) TASK-2 was increased in pregnancy compared to the control under Western blot (595 vs 100, p<0.05; n=3 and 4).
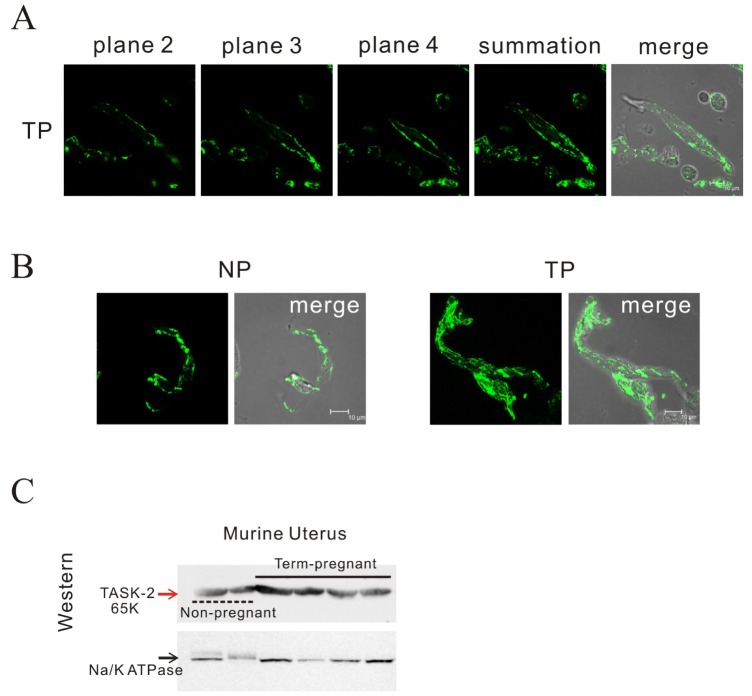