Abstract
The quantitative sudomotor axon reflex testing (QSART) is a classic test of routine postganglionic sudomotor function. We investigated sudomotor function by QSART after summer (July 2012) and winter (January 2013) seasonal acclimation (SA) in the Republic of Korea. QSART with acetylcholine (ACh) iontophoresis were performed to determine directly activated (DIR) and axon reflex-mediated (AXR1, 2) sweating rate. Onset time of axon reflex, activated sweat gland density (ASGD), activated sweat gland output (ASGO), tympanic and skin temperatures (Tty, Tsk), basal metabolic rate (BMR), and evaporative loss volume changes were measured. Tympanic and mean body temperature (Tb; calculated from Tty, Tsk) were significantly lower after summer-SA than that of winter-SA. Sweat onset time was delayed during winter-SA compared to that after summer-SA. BMR, AXR(1), AXR(2), and DIR sweat rates, ASGD and ASGO, and evaporative loss volume were significantly diminished after winter-SA relative to after summer-SA. In conclusion, changes in sweating activity measured by QSART confirmed the involvement of the peripheral nervous system in variation of sudomotor activity in seasonal acclimation.
Many studies have reported changes in heat resistance of residents as they move to different environments or experience seasonal change. Alteration of sudomotor activity is one of the major changes contributing to increased heat resistance in thermal acclimation. Changes of sudomotor activity are presented as changes of the threshold of body temperature to sweating [123456] and of sweat rate [178].
In Japan, where the four seasons are obvious, seasonal acclimation is observed in summer, which is a kind of short-trem acclimation. Comparing sweating reponse to heating in summer and winter, in summer, onset time of sweating is short and sweat rate is higher [910], and Na+ concentration of sweat is lower [9]. It is well known that basal metabolic rate (BMR) also shows seasonal variation of lower values in summer and higher values in winter [1112].
Sweating mechanisms involve both central and peripheral activity, and heat acclimation is a process that is mediated by both the central nervous system and peripheral effectors [13]. Central components are the preoptic area and anterior hypothalamus (PO/AH) and peripheral components are postganaglionic sympathetic nerve fibers and sweat glands [14]. Acetylcholine (ACh) is the primary neurotransmitter involved in this process [1516]. Nadel et al. explained the alterations in sudomotor activity suggesting an idealized model for the relationship between central drive and sweat rate [1]. The mathematical function of the correlation between sweat rate and central sweating drive is altered by horizontal shift or change in slope, resulting in alteration of sweating potential.
Increase in sweating observed in seasonal acclimation is related with augmentation of sudomotor center activity and sweat gland reactivity. Frequency of sweating expulsion can be used as an indicator of central sudomotor activity [17]. Sugenoya and Ogawa investigated frequency of sweating expulsion and body temperature before and after seasonal acclimation (heating rectal temperature to 38℃ for 90 minutes, 9 days) [18]. The results revealed that the relationship between body temperature and frequency of sweating expulsion, representing sudomotor center activity, shifted left, however, the relationship between frequency of sweating expulsion and sweat volume, representing sweat gland reactivity, in response to stimulation from the sudomotor center, didn't change, so Sugenoya and Ogawa claimed central factors are important [18].
Meanwhile, several studies revealed the role of peripheral factors. After seasonal acclimation induced, locally cooled region of the arm didn't experience increased sweating activity [19]. On the other hand, repeatedly submersion of the upper arm in hot water induced incremental increases in local sweating activity without thermal exposure of the whole body or physical training [20]. Administration of methacholine, a sudomotor agonist, repeatedly (twice a day for 10 days), resulted in augmentation of the local sweat response to methacholine in the region.
For all many studies have been conducted to implicate the roles of the central nervous system and peripheral nervous system in thermal acclimation. However, a comprehensive approach embracing both central and peripheral factors has not yet been executed sufficiently. Our prior study [21] confirmed changes in the central nervous system, derived by seasonal acclimation, affecting sweating activity under passive heating, in Korean young men. In line with this, in the present study, we investigated alterations in peripheral sensitivity to central drive to induce sweating, according to the onset of seasonal acclimation, for the same subjects and same environmental conditions, using quantitative sudomotor axon reflex testing(QSART) with ACh iontophoresis.
Fifteen healthy male subjects,wholived all of their lives in the city of Cheonan (Chungnam), Republic of Korea, participated in this study. Cheonanis located in southwestern Korea (126°52'N, 33.38'E) and extends northeast (130°4'N, 43.0'E). The mean annual ambient temperature during July 2012 and January 2013 was 11.45℃ with 73.55% relative humidity (Fig. 1). All individuals had been exposed to these environmental conditions at least 10 h each week (e.g. walking to class, driving to work, exercising outside). All subjects were university students who lived in Cheonan for 6 months before the experiment and during the experimental period (July, 2012~January, 2013). According to the replies to the questionnaire on their everyday life during the experimental period, we decided that all the individuals had acclimated to the weather conditions. General physical characteristics of the subjects are summarized in Table 1. Body surface area (BSA) was calculated in accordance with the Du Bois formula [22]. Body fat percentage was measured using the bio-impedance method (InBody770, Seoul, Korea). The subjects refrained from alcohol, caffeine, smoking, exercise, and medication use 24 h before the test. The subjects were given ~5–7 mL/kg tap water 4 h before testing. However, no subjects consumed water during passive heating in either summer or winter. This protocol was approved by the University of Soonchunhyang research committee. Written informed consent was obtained from all participants after a thorough explanation of the study including its purpose and risks, the procedures complied with the 2000 Declaration of Helsinki of the World Medical Association.
All experiments were carried out in an automated climate chamber (temperature, 24.0±0.5℃; relative humidity, 40±3%;<1 m/sec air velocity) between 2 and 5 PM to minimize the influence of circadian rhythms. Upon arrival to the climate chamber, the subjects were dressed in light clothing and rested quietly for 60 min before the experiment commenced. The QSART was performed to quantitatively evaluate glandular ACh-sensitivity [1623]. The QSART capsule consists of three concentric compartments [1623]. Iontophoretically applied ACh stimulates the underlying sweat glands in the outer compartment directly while the sweat glands in the central compartment of the capsule are activated indirectly via axon reflexes [1623]. The sweating response was measured from the directly activated and axon reflex-mediated sweat responses resulting from iontophoresis. Two sets of QSART capsules were attached to the volar aspect of the forearm with rubber bands, one at the mid portion between the wrist and elbow joint and the other 10 cm proximal to the first [1623]. The outer compartment of the first capsule was filled with a 10% ACh (Ovisot, Daiichi Pharmaceutical Co., Ltd., Tokyo, Japan) solution. Two mA of direct current was applied for 5 min between an electrode on the ACh cell (anode) and a flexible plate-electrode (HV-BIGPAD, Omron, Kyoto, Japan) (cathode) attached to the forearm skin just proximal to the wrist joint [1623]. The central compartment of the ACh capsule served as the site of the sudomotor axon reflex [AXR(1)] measurement during the 5 min of iontophoresis [1623]. The sweat capsules were detached immediately after cessation of current loading, and the skin covered by the ACh capsule was wiped; then the positions of the capsules were exchanged [1623]. This procedure took <20 sec. Data were acquired for an additional 5 min to permit simultaneous observation of DIR and AXR(2) sweating rates without iontophoresis [1623]. The data included sweat onset-time, latent period for sweating after current loading, 5 min sweat volume, area under the sweating curve (0~5 min for AXR(1), and 6~11 min for AXR(2), and DIR sweating rate (mg/cm2 per min) [1623].
Sweat rates were measured by the capacitance hygrometer-ventilated capsule method [1623]. In brief, nitrogen gas flowed into each compartment at a constant flow rate of 0.3 L/min. The change in the relative humidity of the effluent gas was detected by a hygrometer (H211, Technol Seven, Yokohama, Japan). The oral (sublingual) and skin temperatures just beside and 10cm away from the ACh capsule were monitored using thermistors (PXK-67, Technol Seven) connected to a data logger (K-720, Technol Seven). Sweating rates and temperatures were recorded with a personal computer (model CF-T1, Panasonic, Tokyo, Japan) every 5 sec. Sweat gland density was measured using the iodine-impregnated paper method. The iodine-starch paper was placed opposite to the skin surface where the ACh was applied. Blue-black pigmented spots were counted in 0.5 cm×0.5 cm areas under a microscope in triplicate, and average sweat gland density (count/cm2) was calculated [1623]. Sweat output per single gland (µg/min/single gland) was obtained by dividing the DIR sweating rate (mg/cm2/min) by sweat gland density [1623].
BMR was measured with an expired air gas analyzer (Cosmed; Quark Pulmonary Function Testing Lung Volume Module 2 ergo, Rome, Italy).
Tty was assessed in the left ear by inserting a thermistor probe (TSK7+1, Songkitopia, Inchen, Republic of Korea) with a small spring in the ear canal (K923, Takara, Yokohama, Japan), which was connected to a personal computer (model CF-T1, Panasonic, Tokyo, Japan) and a data logger (K-720, Technol Seven, Yokohama, Japan). When the thermistor probe contacted the tympanic membrane, the subject felt slight discomfort and could hear a scratching noise. The inner pinna was filled with small cotton balls to fix the probe in the ear [21].
Skin temperatures (Ts) of the chest (Tschest), upper arm (Tsarm), thigh (Tsthigh), and leg (Tsleg) were measured using thermistor thermometers (TSK7+1, Songkitopia) connected to a data logger (K-720, Technol Seven). Tsk was calculated in accordance with the Ramanathan equation: (Ramanathan, 1964) Tsk=0.3 · (Tschest+Tsarm)+0.2 · (Tsthigh+Tsleg). Tb was calculated according to Ramanathan's formula [24] as well as to Sugenoya and Ogawa [18] from Tty) and Tsk by the following equation: Tb)=(0.9 · Tty)+0.1 · Tsk).
Evaporative loss volume (µg/cm2/min) was measured with a Tewameter (model No. TM 210; Courage and Khazaka, Cologne, Germany) according to the manufacturer'srecommendations and as described previously in detail [25]. Briefly, the measurement involved placing the probe device on the volar aspect of the forearm [1621].
BMR was 40.18±9.30 kcal/m2/h (summer-SA) and 49.80±12.45 kcal/m2/h (winter-SA). BMR was significantly lower by 19.32% after summer-SA compared to that after winter-SA (p<0.001).
A significant difference was observed in onset time of the axon reflex. Onset time of summer-SA (1.57±0.53 min) was shorter than that of winter-SA (1.87±0.38 min) (p<0.001) (Fig. 2). The AXR (1) sweat rate of summer-SA was greater than that of winter-SA (0.61±0.26 vs. 0.49±0.21 mg/cm2/min) (p<0.01) (Fig. 3). The AXR (2) sweat rate of summer-SA was greater than that of winter-SA (0.71±0.32 vs. 0.52±0.27 mg/cm2/min) (p<0.01) (Fig. 4). DIR sweat rate of summer-SA was greater than that of winter-SA (1.07±0.35 vs. 0.85±0.28 mg/cm2/min) (p<0.001) (Fig. 5). The mean ASGD of summer-SA was greater than that of winter-SA (105±20.2 vs. 93±16.5 count/cm2) (p<0.001) (Fig. 6). Additionally, mean ASGO of summer-SA was greater than that of winter-SA (10.19±1.2 vs. 9.14±1.08 µg/min/single gland) (p<0.001) (Fig. 7).
As shown in Fig. 8, evaporative loss volume was significantly lower after winter-SA than that after summer-SA on the chest (8.15±1.80 vs. 10.30±2.18 µg/cm2/min), upper back (8.11±1.85 vs. 11.27±2.64 µg/cm2/min), thigh (7.01±1.73 vs. 9.92±1.66 µg/cm2/min) and forearm (6.09±1.18 vs. 9.47±1.34 µg/cm2/min) (p<0.001).
The subjects had lower Tty and Tb after summer-SA than winter-SA, which is consistent with a previous study [21]. This is related to the relatively low BMR and set-point temperature. Lower BMR is more favorable for maintaining a lower body temperature in a hot environment and for having greater tolerance to heat [26].
Short-term heat exposure reduces sweating onset time and increases the amount of sweating [27]. Short-term acclimation occurs in a short period of time (days to weeks) after exposure [2829]. The present study obtained measurements for Exp 1 (after summer-SA) in July, and short-term acclimation seemed to be provoked by increased temperatures. In our previous study on central factors, passive heating in half bath for an hour a day, for 10 days, lead to increased sweat rate [14].
The changes in sweating activity measured by QSART using ACh iontophoresis showed alteration of reactivity of the peripheral nervous system, and the direction of changes were accordant with a previous study concerning central nervous system [21]. This confirms that the peripheral nervous system contributes to changes in sweating activity by heat acclimation, in parallel with the contribution of the central system which was demonstrated in our previous study [21].
In the results from the QSART, sweat onset time of winter-SA was significantly delayed relative to that of summer-SA. Longer local sweat onset time after summer-SA is associated with decreased cholinergic sensitivity of sweat glands [30].
Concerning sweat rates obtained by QSART, all compartments (AXR(1), AXR(2), and DIR) were diminished after winter-SA relative to after summer-SA, which means decreased peripheral sensitivity to ACh derived from low atmospheric temperature of the winter season.
Lower sweat rate after winter-SA relative to after summer-SA is the result of decay of acclimation. Low air temperature decreases endogenous ACh concentration and sweat gland sensitivity to ACh. Short-term acclimation is the process of gaining heat tolerance and is known to decay after 2 weeks from the last heat exposure date [31]. Also in other studies, the duration of decay was reported as 6 to 21 days [832]. The decay of acclimation is not a singular process, but has stages of progression. Studies on decay of short-term acclimation have reported that heart rate and sweat loss are altered more rapidly than core (rectal) temperature and heat tolerance [833].
Higher activated sweat gland density and sweat gland output after summer-SA showed that not only the reactivity, but also the anatomy of sweat glands are involved in alteration of sudomotor activity. Higher single sweat gland ouput may be associated with both sensitivity to ACh and the size of involved sweat glands. Our previous study reported that after 10 days of passive heating using half bath, activated sweat gland density and sweat gland output volume increased as well as the evaporated quantity of sweat [14]. In agreement with these reports, Taylor [34] and Sato et al. [35] showed that short-term acclimation makes sweat glands bigger and more sensitive to methacholine stimulus.
When short-term acclimation is complete, the increase in the volume of sweat allows the body to compensate for the increase in body temperature [31]. The reduction of the Na+ concentration reduces the loss of Na+ due to sweating [936]. In addition to changes in sweating, lowered heart rate and slower rise in core temperature during heating aspects of heat resistance acquired by short-term acclimation [15637].
In long-term acclimation, the peripheral nervous system also plays an important role. The direction in the changes to sudomotor activity is opposite to that of short-term acclimation. Several studies have confirmed the reduced sweating response after long-term acclimation [2,6,38]. In particular, tropical natives who have acclimatized to high air temperatures have lower sweat gland density and lower sweat gland output per gland [16]. Lee et al. [16] suggested that these effects of long-term acclimation are mainly due to the peripheral sympathetic nervous system blunting nerve terminals to incoming cholinergic signals.
Furthermore, reduction in sweating acitivity can be achieved within shorter periods of direct stimulation. Chen and Elizondo [19] administered ACh 4 times a day, for 9 days, and then found that subjects' local sweating under passive heating was almost completely suppressed. This result implies that decreased thermal sweating can result from desensitization of the nerve-gland interface derived by repetitive stimulation.
Prior studies have shown that peripheral mechanisms play a predominant role, compared to the central nervous system, in the suppression or augmentation of sweating [2039]. However, other studies, including our previous study [21], have identified the engagement of the central nervous system in heat acclimation. In temperate regions of Japan, which have similar seasonal changes to temperate regions of Korea, Nakamura and Okamura [40] measured the annual variation in body temperature (core and skin temperature) and integrated sweat loss by passive heating. As a result, skin temperature was found to be proportional to air temperature and core temperature was lower in summer than in winter. Sweat loss volume is greater in the summer. This shows a corresponding tendency, presenting the role of central mechanism in seasonal acclimation.
In this study, the results confirmed the involvement of the peripheral nervous system in variation of sudomotor activity during seasonal acclimation. In line with the changes of set-point temperature and sweating threshold which were also revealed in our previous study [21], sweat glands, peripheral nerves, and their interfaces are modified to become activated after summer-SA and deactivated after winter-SA. In conclusion, our two consecutive studies comprehensively identified that a central mechanism and a peripheral mechanism act simultaneously to regulate sweating activity.
Acknowledgements
We thank the subjects whose participation made this study possible. We appreciate K.S. Song providing thermistor probes. This work was supported by the Soonchunhyang University Research Fund.
Notes
References
1. Nadel ER, Pandolf KB, Roberts MF, Stolwijk JA. Mechanisms of thermal acclimation to exercise and heat. J Appl Physiol. 1974; 37:515–520. PMID: 4414615.


2. Lee JB, Matsumoto T, Othman T, Kosaka M. Suppression of the sweat gland sensitivity to acetylcholine applied iontophoretically in tropical Africans compared to temperate Japanese. Trop Med. 1997; 39:111–121.
3. Griefahn B. Acclimation to three different hot climates with equivalent wet bulb globe temperatures. Ergonomics. 1997; 40:223–234. PMID: 9118933.


4. Li X, Tokura H, Midorikawa T. The effects of two different types of clothing on seasonal warm acclimatization. Int J Biometeorol. 1995; 38:111–115. PMID: 7744523.


5. Nielsen B. Heat acclimation--mechanisms of adaptation to exercise in the heat. Int J Sports Med. 1998; 19(Suppl 2):S154–S156. PMID: 9694425.
6. Ogawa T, Sugenoya J. Pulsatile sweating and sympathetic sudomotor activity. Jpn J Physiol. 1993; 43:275–289. PMID: 8230848.


7. Nielsen B, Hales JR, Strange S, Christensen NJ, Warberg J, Saltin B. Human circulatory and thermoregulatory adaptations with heat acclimation and exercise in a hot, dry environment. J Physiol. 1993; 460:467–485. PMID: 8487204.


8. Williams CG, Wyndham CH, Morrison JF. Rate of loss of acclimatization in summer and winter. J Appl Physiol. 1967; 22:21–26. PMID: 6017648.


9. Hori S, Tanaka N. Adaptive changes in physiological responses of men to heat induced by heat acclimatization and physical training. Jpn J Trop Med Hyg. 1993; 21:193–199.


10. Matsumoto T, Kosaka M, Yamaguchi M, Nakamura K, Yang GJ, Amador JJ. Seasonal variation of thermal sweating. Trop Med. 1990; 32:73–80.
11. Warwick PM, Busby R. Influence of mild cold on 24 h energy expenditure in 'normally' clothed adults. Br J Nutr. 1990; 63:481–488. PMID: 2383527.


12. Plasqui G, Kester AD, Westerterp KR. Seasonal variation in sleeping metabolic rate, thyroid activity, and leptin. Am J Physiol Endocrinol Metab. 2003; 285:E338–E343. PMID: 12857676.
13. Horowitz M. Heat acclimation: a continuum of process. In : Mercer J, editor. Thermal Physiology. Amsterdam: Elsevier Science Publishers B.V;1989.
14. Lee JB, Kim TW, Shin YO, Min YK, Yang HM. Effect of the heat-exposure on peripheral sudomotor activity including the density of active sweat glands and single sweat gland output. Korean J Physiol Pharmacol. 2010; 14:273–278. PMID: 21165324.


15. Vetrugno R, Liguori R, Cortelli P, Montagna P. Sympathetic skin response: basic mechanisms and clinical applications. Clin Auton Res. 2003; 13:256–270. PMID: 12955550.
16. Lee JB, Bae JS, Matsumoto T, Yang HM, Min YK. Tropical malaysians and temperate koreans exhibit significant differences in sweating sensitivity in response to iontophoretically administered acetylcholine. Int J Biometeorol. 2009; 53:149–157. PMID: 19048305.


17. Shibasaki M, Inoue Y, Kondo N. Mechanisms of underdeveloped sweating responses in prepubertal boys. Eur J Appl Physiol Occup Physiol. 1997; 76:340–345. PMID: 9349649.


18. Sugenoya J, Ogawa T. Characteristics of central sudomotor mechanism estimated by frequency of sweat expulsions. Jpn J Physiol. 1985; 35:783–794. PMID: 4079134.


19. Chen WY, Elizondo RS. Peripheral modification of thermoregulatory function during heat acclimation. J Appl Physiol. 1974; 37:367–373. PMID: 4370254.


20. Ogawa T, Asayama M, Miyagawa T. Effects of sweat gland training by repeated local heating. Jpn J Physiol. 1982; 32:971–981. PMID: 7169703.


21. Lee JB, Kim TW, Min YK, Yang HM. Seasonal acclimatization in summer versus winter to changes in the sweating response during passive heating in korean young adult men. Korean J Physiol Pharmacol. 2015; 19:9–14. PMID: 25605991.


22. Du Bois D, Du Bois EF. Clinical calorimetry: tenth paper a formula to estimate the approximate surface area if height and weight be known. Arch Intern Med. 1916; XVII(6_2):863–871.
23. Lee JB, Lee IH, Shin YO, Min YK, Yang HM. Age-and sex-related differences in sudomotor function evaluated by the quantitative sudomotor axon reflex test(QSART) in healthy humans. Clin Exp Pharmacol Physiol. 2014; 41:392–399. PMID: 24684442.
24. Ramanathan NL. A new weighting system for mean surface temperature of the human body. J Appl Physiol. 1964; 19:531–533. PMID: 14173555.


25. Pinnagoda J, Tupker RA, Agner T, Serup J. Guidelines for transepidermal water loss (TEWL) measurement. A report from the standardization group of the european society of contact dermatitis. Contact Dermatitis. 1990; 22:164–178. PMID: 2335090.
27. Kuno Y. Human perspiration. Springfield: Thomas;1956.
28. Pandolf KB. Time course of heat acclimation and its decay. Int J Sports Med. 1998; 19(Suppl 2):S157–S160. PMID: 9694426.


29. Sawka MN, Wenger CB. Thermoregulatory responses to acute exercise heat stress and heat acclimation. In : Sawka MN, Wenger CB, Pandolf KB, editors. Comprehensive Physiology. 1996.
30. Kim TW, Shin YO, Lee JB, Min YK, Yang HM. Caffeine increases sweating sensitivity via changes in sudomotor activity during physical loading. J Med Food. 2011; 14:1448–1455. PMID: 21883004.


31. Lind AR, Bass DE. Optimal exposure time for development of acclimatization to heat. Fed Proc. 1963; 22:704–708. PMID: 13930724.
32. Pandolf KB, Burse RL, Goldman RF. Role of physical fitness in heat acclimatisation, decay and reinduction. Ergonomics. 1977; 20:399–408. PMID: 908323.


33. Armstrong LE, Maresh CM. The induction and decay of heat acclimatisation in trained athletes. Sports Med. 1991; 12:302–312. PMID: 1763248.


34. Taylor NA. Eccrine sweat glands. Adaptations to physical training and heat acclimation. Sports Med. 1986; 3:387–397. PMID: 3538269.
35. Sato F, Owen M, Matthes R, Sato K, Gisolfi CV. Functional and morphological changes in the eccrine sweat gland with heat acclimation. J Appl Physiol(1985). 1990; 69:232–236. PMID: 2203723.


36. Nielsen B, Strange S, Christensen NJ, Warberg J, Saltin B. Acute and adaptive responses in humans to exercise in a warm, humid environment. Pflugers Arch. 1997; 434:49–56. PMID: 9094255.


37. Wyndham CH. Effect of acclimatization on the sweat rate-rectal temperature relationship. J Appl Physiol. 1967; 22:27–30. PMID: 6017649.


38. Matsumoto T, Kosaka M, Yamauchi M, Tsuchiya K, Ohwatari N, Motomura M, Otomasu K, Yang GJ, Lee JM, Boonayathap U, Praputpittaya C, Yongsiri A. Study on mechanisms of heat acclimatization due to thermal sweating: comparison of heat tolerance between Japanese and Thai subjects. Trop Med. 1993; 35:23–34.
39. Collins KJ, Weiner JS. Observations on arm-bag suppression of sweating and its relationship to thermal sweat-gland 'fatigue'. J Physiol. 1962; 161:538–556. PMID: 13880598.


Fig. 1
Monthly mean daily average ambient temperatures from July 2012 to January 2013 in Cheonan (126°52'N, 33.38'E; Republic of Korea).
Cheonan is located in a temperate zone (four distinct geopolitical seasons). The monthly mean daily average ambient temperature during the experimental period was from July 20, 2012 (Exp 1) to January 20, 2013 (Exp 2). Exp 1;Experiment 1 hot summer (July. 2012; temperature, 25.8±1.7℃; relative humidity, 78.9±5.5%) and Exp 2; Experiment 2 cold winter (January. 2013; temperature, –3.6±4.9℃; humidity, 69.7±11.6%).
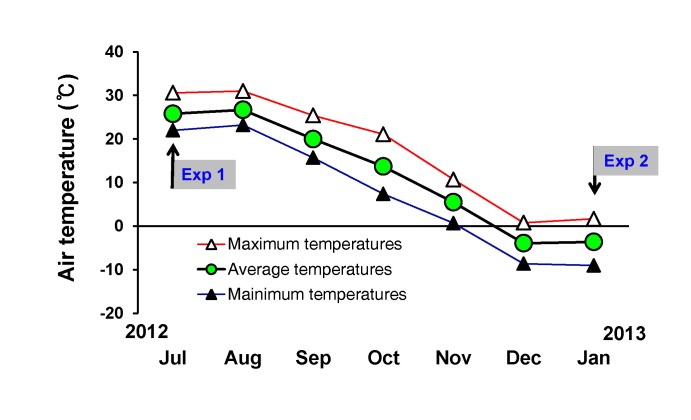
Fig. 2
Axon reflex sweat onset time during acetylcholine (ACh) iontophoresis in summer-SA and winter-SA.
Values are mean±SD. Statistically significant differences were set at ***p<0.001.
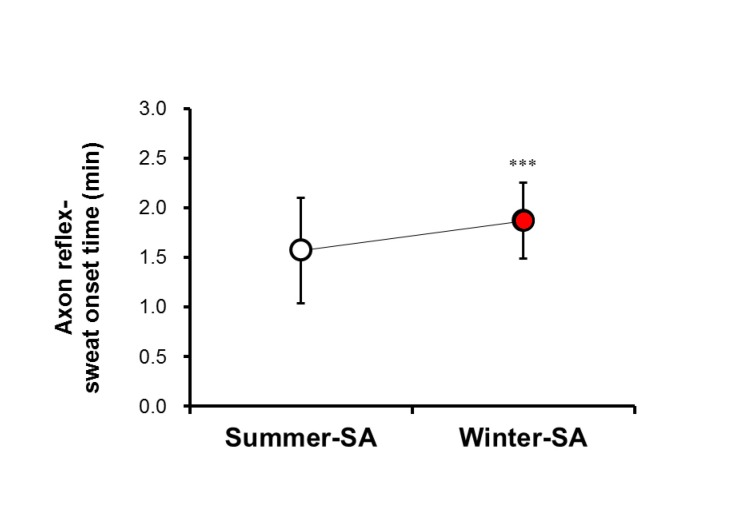
Fig. 3
Axon reflex-mediated [AXR (1), indirect sudomotor activity caused by activating nicotinic receptors] sweat rate during QSART with acetylcholine (ACh) iontophoresis in summer-SA and winter-SA.
Values are mean±SD. Statistically significant differences were set at **p<0.01.
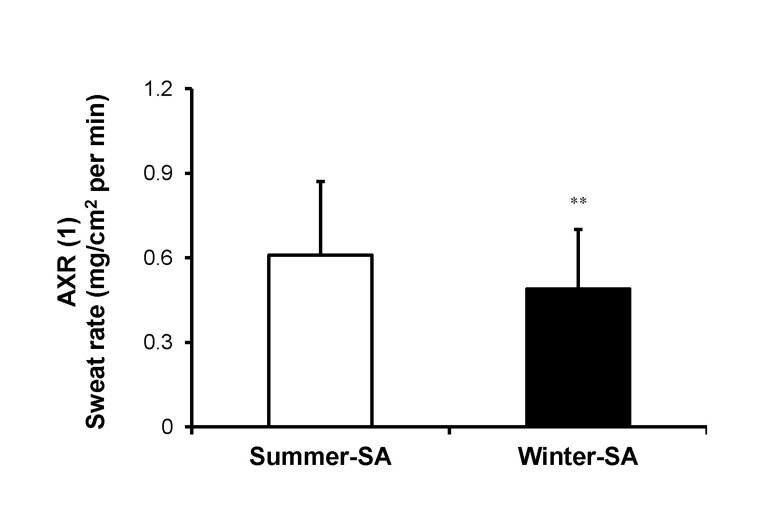
Fig. 4
Axon reflex-mediated [AXR (2), indirect sudomotor activity caused by activating nicotinic receptors] sweat rate during QSART without acetylcholine (ACh) iontophoresis in summer-SA and winter-SA.
Values are mean±SD. Statistically significant differences were set at **p<0.01.
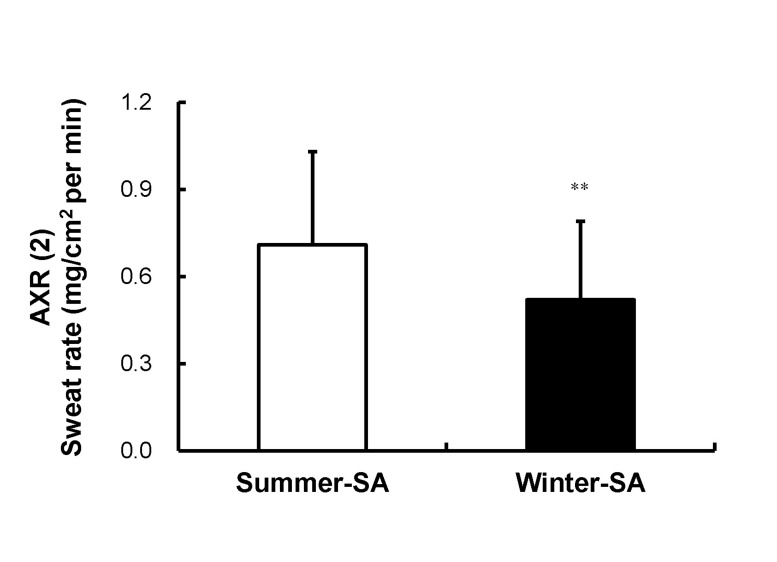
Fig. 5
Directly activated [DIR, induces direct sudomotor activity caused by activating muscarinic receptors] sweat rate in summer-SA and winter-SA.
Values are mean±SD. Statistically significant differences were set at ***p<0.001.
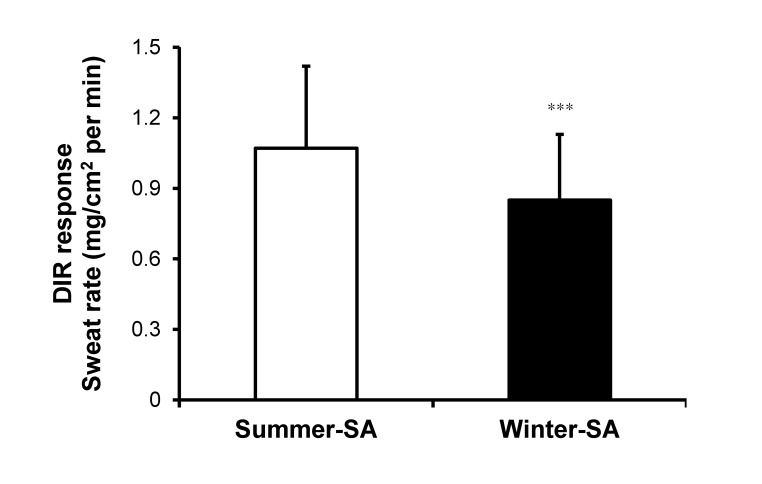
Fig. 6
Activated sweat gland density (ASGD) of DIR (direct sudomotor activity caused by activating muscarinic receptors) sweating in summer-SA and winter-SA.
Values are mean±SD. Statistically significant differences were set at ***p<0.001.
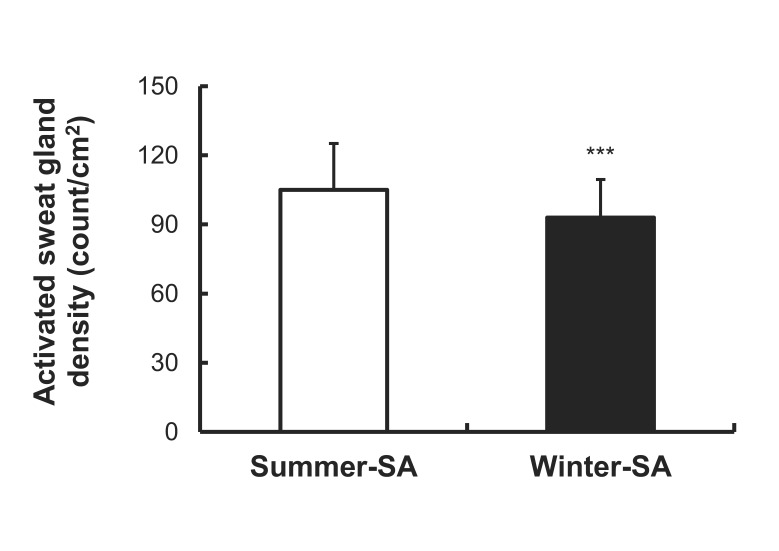
Fig. 7
Activated sweat gland output (ASGO) of activated sweat gland density by DIR sweating.
ASGO of summer-SA and winter-SA during QSART. Values are mean±SD. Statistically significant differences were set at ***p<0.001.
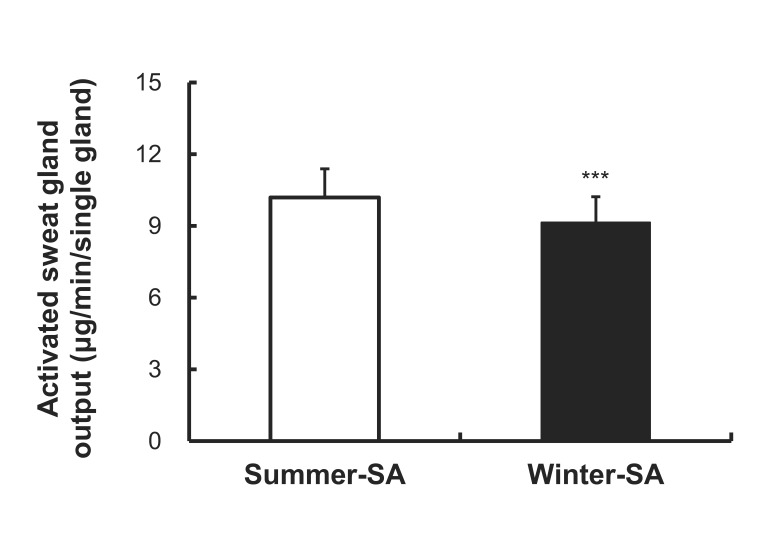
Fig. 8
Volume of skin evaporative loss volume (evaporative rate) during passive heating.
Values are mean±standard deviation. Statistically significant differences were set at ***p<0.001.
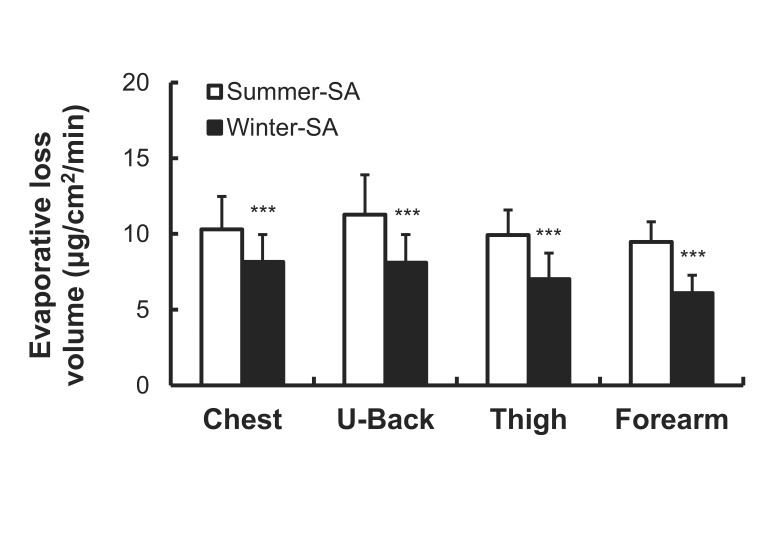