Abstract
TWIK-related K+ channel-2 (TREK-2) and TWIK-related spinal cord K+ (TRESK) channel are members of two-pore domain K+ channel family. They are well expressed and help to set the resting membrane potential in sensory neurons. Modulation of TREK-2 and TRESK channels are involved in the pathogenesis of pain, and specifi c activators of TREK-2 and TRESK may be benefi cial for the treatment of pain symptoms. However, the effect of commonly used analgesics on TREK-2 and TRESK channels are not known. Here, we investigated the effect of analgesics on TREK-2 and TRESK channels. The effects of analgesics were examined in HEK cells transfected with TREK-2 or TRESK. Amitriptyline, citalopram, escitalopram, and fluoxetine significantly inhibited TREK-2 and TRESK currents in HEK cells (p<0.05, n=10). Acetaminophen, ibuprofen, nabumetone, and bupropion inhibited TRESK, but had no effect on TREK-2. These results show that all analgesics tested in this study inhibit TRESK activity. Further study is needed to identify the mechanisms by which the analgesics modulate TREK-2 and TRESK differently.
Pain is associated with a wide range of injury and disease. Worldwide, more than 1.5 billion people suffer from acute or chronic pain, as reported by Global Industry Analysts. Global Burden of Disease (GBD) study shows that burdens associated with chronic pain are increasing in many types of diseases and injuries [1]. In addition, pain causes depression and vice versa. There is a close relationship between pain and depression. Antidepressant medications help relieve pain and depression [2]. Numerous approaches for the treatment of chronic pain have been investigated, but the management of chronic pain with the available pharmacologic therapies is often unsatisfactory. New therapeutic approaches for the prevention and treatment of chronic pain are needed.
A large number of studies have shown that ion channels are closely connected with chronic pain [345]. TWIK-related K+ channel-2 (TREK-2) and TWIK-related spinal cord K+ (TRESK) channel are members of two-pore domain K+ (K2P) channel. These channels are the main contributors to the background current in dorsal root ganglion (DRG) neurons [67]. TREK-2 is involved in mechanical and osmotic pain and in cold allodynia [8]. In addition, TREK-2 expressed in IB4-binding C-fiber nociceptors limits spontaneous pain [9]. TRESK channels activated by the inflammatory mediator (lysophosphatidic acid) reduce nociceptive signaling in DRG neurons [10]. TRESK is associated with migraine [3711]. Familial migraine with aura is associated with a dominant-negative mutation in human TRESK channels. A dominant-negative mutation in TRESK induces hyperexcitability when expressed in TG neurons [11]. TRESK knockdown mice show increased pain and sensitivity in response to painful stimuli. Thus, a prominent physiological role of TREK-2 and TRESK has been attributed to pain sensation [12]. Therefore, an up-regulation of TREK-2 and TRESK channel activities may be a useful strategy in the development of new therapies for the treatment of many types of pain. Activators of the TREK and TRESK channel are expected to emerge as a novel class of analgesic agents [13].
Non-steroidal anti-inflammatory drugs (NSAIDs), such as ibuprofen and nabumetone, are widely used for pain relief. In addition to classical analgesics, antidepressants are useful therapeutic strategies for pain [131415]. Amitriptyline, bupropion, and fluoxetine produced antiallodynic effects in chronic constriction injured mice [16]. Of antidepressants, tricyclic antidepressants (TCAs) are the most established drugs in the treatment of chronic pain [1718], but selective serotonin reuptake inhibitors (SSRIs) or selective norepinephrine reuptake inhibitors (SNRIs) are also effective against pain [1819].
A better understanding of the relationships between these drugs and the changes they induce in TREK-2 and TRESK channels should help to identify more effective treatments for pain. This study was performed to identify the effect of analgesics that are currently used in clinics on TREK-2 and TRESK channels.
All of the chemicals used in this study were purchased from Sigma Chemical Company (St. Louis, MO, USA) unless otherwise specified. Stock solutions of escitalopram (20 mM), fluoxetine (100 mM), and ibuprofen (100 mM) were prepared in dimethyl sulfoxide (DMSO) and then diluted in experimental solution to a working concentration. Acetaminophen (500 mM) was dissolved in ethanol. Amitriptyline (100 mM), bupropion (100 mM), citalopram (10 mM), and nabumetone (100 mM) were dissolved in distilled water. When DMSO or ethanol was used as a solvent, a solution containing an equivalent concentration was used as a control.
HEK293 cells were seeded at a density of 2×105 cells per 35 mm dish 24 h prior to transfection in Dulbecco's modified Eagle's medium (DMEM) containing 10% FBS. HEK293 cells were co-transfected with DNA fragments encoding mouse TRESK (NM_207261) or rat TREK-2 (NM_023096) and green fluorescent protein (GFP) in pcDNA3.1 using LipofectAMINE2000 and OPTI-MEM I Reduced Serum Medium (Life technologies, Grand Island, NY, USA). Green fluorescence from cells expressing GFP was detected with the aid of a Nikon microscope equipped with a mercury lamp light source. Cells were used 2~3 days after transfection.
Electrophysiological recording was performed using a patch clamp amplifier (Axopatch 200, Axon Instruments, Union City, CA, USA). Pipette tip resistances were 4~6 MΩ. Wholecell current was recorded in response to a voltage ramp (–120 to +60 mV; 865 ms duration) from a holding potential of –80 mV in physiological solution containing 5 mM KCl. Currents were filtered at 2 kHz, and the currents measured at +60 mV were obtained and analyzed. Bath solution contained (mM): 135 NaCl, 5 KCl, 1 CaCl2, 1 MgCl2, 5 glucose and 10 HEPES (pH 7.4), and pipette solutions contained (mM): 150 KCl, 1 MgCl2, 5 EGTA and 10 HEPES (pH 7.3). The pH was adjusted to desired values with HCl or NaOH. All experiments were performed at ~25℃.
Application of the NSAIDs (ibuprofen and nabumetone) and acetaminophen to TREK-2 and TRESK channels that were overexpressed in HEK-293 cells produced different effect in TREK-2 and TRESK currents. As shown in Fig. 1, acetaminophen (100 µM), ibuprofen (100 µM), and nabumetone (100 µM) inhibited TRESK by 27±7%, 21±9%, and 32±11%, respectively, but they did not affect TREK-2. The IC50 for inhibition of TRESK by acetaminophen, ibuprofen, and nabumetone was 220±26 µM, 889±10 µM, and 557±35 µM.
Antidepressants used in this study, except bupropion, inhibited both TREK-2 and TRESK currents. Amitriptyline (a TCA, 30 µM) inhibited TREK-2 and TRESK currents by 71±10% and 54±16%, respectively. The inhibitory effects of amitriptyline were higher on TREK-2 current than on TRESK current. The IC50 for inhibition of TREK-2 and TRESK by amitriptyline was 16±4 µM and 26±5 µM, respectively (Fig. 2A). Bupropion, a specific dopamine reuptake inhibitor, had no effect on TREK-2, but inhibited TRESK currents by 30±17%. The IC50 for inhibition of TRESK by bupropion was 160±17 µM (Fig. 2B). Bar graphs show the effect of amitriptyline and bupropion on TREK-2 and TRESK. Also, SSRIs (fluoxetine, citalopram, and escitalopram) inhibited both TREK-2 and TRESK currents. Citalopram, escitalopram, and fluoxetine inhibited TREK-2 currents by 49±10%, 55±12%, and 71±8% at the concentration of 100 µM (Fig. 3A). Citalopram, escitalopram, and fluoxetine also inhibited TRESK currents by 30±6%, 72±7%, and 92±10%, respectively (Fig. 3B). The IC50 for inhibition of TREK-2 by citalopram, escitalopram, and fluoxetine was 110±9 µM, 102±9 µM, and 31±7 µM, respectively. The IC50 for inhibition of TRESK by citalopram, escitalopram, and fluoxetine was 168±11 µM, 49±7 µM, and 17±5 µM, respectively. The inhibitory effect of escitalopram and fluoxetine was higher on TRESK current than on TREK-2 current. The inhibitory effect of citalopram on TREK-2 was similar to that on TRESK. The currents recorded from GFP-transfected cells were ~10 pA/pF. All drugs had no effect on the currents (data not shown).
This study reports the effects of therapeutic compounds for pain on TREK-2 and TRESK currents. We hypothesized that analgesics commonly used in clinics may activate TREK-2 and TRESK, because a loss-of function in TREK-2 and TRESK induces depolarization and results in pain [78910]. However, the most of analgesics tested in this study inhibited TREK-2 and TRESK currents. These compounds included amitriptyline, citalopram, escitalopram, and fluoxetine. Acetaminophen, bupropion, ibuprofen, and nabumetone showed different effect on TREK-2 and TRESK; they inhibited TRESK currents, but had no effect on TREK-2.
The concentrations of compounds used in this study were lower than adult dose, as reported by U.S. Food and Drug Administration (FDA), but slightly higher than their blood concentrations. However, in the case of acetaminophen (15 µg/mL), ibuprofen (20 µg/mL), and nabumeton (22.8 µg/mL), the concentrations were similar to their blood concentrations ranging 5 to 20 µg/mL [20], 8.4 µg/mL when receiving 400 mg/day ibuprofen [21], and 12~16 mg after administration of 2000 mg/day nabumetone (U.S. FDA, NDA 19-538/S-023), respectively. The dose of ibuprofen can be increased to 1200 mg/day. The blood concentration of nabumetone is calculated with its principal active metabolite 6-methoxy-2-naphthylacetic acid (6MNA) detected in the blood after administration of nabumetone. The blood concentrations of amitriptyline, bupropion, citalopram, escitalopram, and fluoxetine are 0.34 µg/ mL, 818±500 ng/mL, 49 ng/mL, 11.6~46.4 ng/mL, and 97±51 ng/mL after oral administration of 10 mg/day [22], 252 mg/ day [23], 20 mg/day citalopram [24], 5~40 mg/day [25], and 20 mg/day [26], respectively. Their blood concentrations were lower than concentrations used in this study (amitriptyline (8.4 µg/mL), bupropion (23.9 µg/mL), citalopram (32.4 µg/mL), escitalopram (41.4 µg/mL), and fluoxetine (30.9 µg/mL)). The low blood concentration of antidepressants will not be likely to inhibit TRESK and TREK-2 currents in vivo. However, the dose can be increased depending on symptom improvement. In addition, the concentrations of drugs are different among organs (e.g. fluoxetine concentration is different between blood and brain; the concentration is higher in brain than in blood [27]). Acetaminophen, ibuprofen, and nabumetone have a possibility that they affect TRESK in in vivo.
Acetaminophen and NSAIDs could modulate K+ channels. NSAIDs activate the Kv7 channel and large-conductance Ca2+-activated K+ channel in vascular smooth muscle cells [2829]. In contrast, NSAIDs inhibit the surface expression of Kv1.4 and Kv1.6 and depolarize membrane potentials in intestinal epithelial cells [30]. Acetaminophen protects estramustineinduced cytotoxicity on cultured fibroblast by blocking inhibition of cellular K+ channel ion transport [31]. These drugs affect K+ channels expressed in different type of cells differently. Acetaminophen, ibuprofen, and nabumetone can be used to distinguish TREK-2 and TRESK expressed in sensory neurons. Further study will be needed to identify the mechanism by which acetaminophen, ibuprofen, and nabumetone modulate TREK-2 and TRESK current differently.
Antidepressants, which have analgesic effects, modulate many types of K+ channels. Within K2P channel family, TREK- 1 and TASK-3 have been suggested as potential targets for antidepressants [323334]. These channels are inhibited by fluoxetine. Our previous study reported that TREK-2 and TRESK currents were inhibited by fluoxetine [35,36]. The present study demonstrates that TREK-2 and TRESK are also inhibited by other antidepressants, such as amitriptyline, citalopram, and escitalopram. Bupropion showed different effect on TREK- 2 and TRESK. Bupropion, which inhibits the reuptake of norepinephrine and dopamine, improves pain relief [37]. In addition, bupropion interacts with nicotinic receptor [38]. Bupropion blocks KATP channel as well as TRESK [39]. TREK- 2 and TRESK show different effect in response to some signals. Acetylcholine (carbachol) inhibits TREK-2 currents, but activates TRESK current [354041]. Aristolochic acid, a traditional medicine used in the treatment of pain, activates TREK-2, but inhibits TRESK activity [42]. TREK-2 channels are activated by low intracellular pH (pHi) and arachidonic acid, and inhibited by PKC activation [4043]. In contrast, TRESK channels are inhibited by low pHi and arachidonic acid [44], and human (not murin) TRESK is activated by PKC [45]. In this study, we tested effects of analgesics and antidepressants on rat TREK-2 and mouse TRESK. Species difference could explain the different effects of the drugs on TREK-2 and TRESK. In addition, the state of phosphorylation is different between TREK-2 and TRESK. TRESK is constitutively phosphorylated under resting conditions [46]. TREK-2 phosphorylated by PKC shows low channel activity [40], whereas TRESK dephosphorylated by calcineurin shows high channel activity [41]. These factors may cause different effect of acetaminophen, ibuprofen, nabumetone, and bupropion on TREK-2 and TRESK. The mechanism that modulates these currents will need to be identified to develop specific drugs for depression and pain.
However, at this point, several questions remain to be answered. Are TREK-2 and TRESK inhibitions related to pain? What do TREK-2 and TRESK inhibitions by analgesics used in clinics indicate? DRG and TG neurons predominantly express TREK-2 and TRESK channels among K2P channel family [647]. Single or a combination of TREK-2 and TRESK has been considered as promising therapeutic targets for pain management [12]. The blockade of both TREK-2 and TRESK currents in DRG and TG neurons would cause cell depolarization and increase cell excitability. These agents might result in a reduced effect of pain medication. In this study, we could not determine the relationship between blockade of TREK-2 and TRESK by analgesics and pain. The inhibition of TREK-2 and TRESK channels by analgesics should be considered when assessing the various pharmacological effects produced by analgesics. People respond differently to pain medications because each pain condition is unique. The different effects observed among people could result from TREK-2 and TRESK inhibitions. Further, TREK-2 and TRESK expression could be different among individuals. On the other hand, activation of inhibitory interneurons could reduce pain transmission [48]. Depolarization of resting membrane potential by blockade of TREK-2 and TRESK affect interneurons. To our knowledge, however, there is no report about the expression of TREK-2 and TRESK in inhibitory interneurons. So far, there are no specific modulators of TREK-2 and TRESK channel. Few compounds are known to inhibit TREK-2 and TRESK current. Our data add to the number of compounds that inhibit TREK-2 and TRESK currents and provide new tools to identify TREK-2 and TRESK channels expressed in primary cells, such as TG and DRG neurons.
In conclusion, we found that the drugs with anti-pain effects did not activate TREK-2 and TRESK. Our study addresses the pharmacological modulation of TREK-2 and TRESK channels with special respect to the possible clinical application of alleviating or preventing pain. Future studies should take into account the therapeutic effect of TREK-2 and TRESK on pain, and the mechanism by which analgesics inhibit TREK-2 and TRESK currents.
ACKNOWLEDGEMENTS
This work was supported by grants from the National Research Foundation of Korea (NRF-2015R1D1A3A01017856 and NRF-2015R1A-5A2-008833), which are funded by the Korean Government (Ministry of Education, Science and Technology and Ministry of Science, ICT and Future Planning), and from Academy-oriented Research Funds of Development Fund Foundation, Gyeongsang National University (GNUDFF-2013-1126).
References
1. Global Burden of Disease Study 2013 Collaborators. Global, regional, and national incidence, prevalence, and years lived with disability for 301 acute and chronic diseases and injuries in 188 countries, 1990-2013: a systematic analysis for the Global Burden of Disease Study 2013. Lancet. 2015; 386:743–800. PMID: 26063472.
2. Hall-Flavin DK, Winner JG, Allen JD, Jordan JJ, Nesheim RS, Snyder KA, Drews MS, Eisterhold LL, Biernacka JM, Mrazek DA. Using a pharmacogenomic algorithm to guide the treatment of depression. Transl Psychiatry. 2012; 2:e172. PMID: 23047243.


4. Cohen GL. Migraine prophylactic drugs work via ion channels. Med Hypotheses. 2005; 65:114–122. PMID: 15893128.


5. Tsantoulas C, McMahon SB. Opening paths to novel analgesics: the role of potassium channels in chronic pain. Trends Neurosci. 2014; 37:146–158. PMID: 24461875.


6. Kang D, Kim D. TREK-2 (K2P10.1) and TRESK (K2P18.1) are major background K+ channels in dorsal root ganglion neurons. Am J Physiol Cell Physiol. 2006; 291:C138–C146. PMID: 16495368.
7. Lafrenière RG, Cader MZ, Poulin JF, Andres-Enguix I, Simoneau M, Gupta N, Boisvert K, Lafrenière F, McLaughlan S, Dubé MP, Marcinkiewicz MM, Ramagopalan S, Ansorge O, Brais B, Sequeiros J, Pereira-Monteiro JM, Griffiths LR, Tucker SJ, Ebers G, Rouleau GA. A dominant-negative mutation in the TRESK potassium channel is linked to familial migraine with aura. Nat Med. 2010; 16:1157–1160. PMID: 20871611.


8. Pereira V, Busserolles J, Christin M, Devilliers M, Poupon L, Legha W, Alloui A, Aissouni Y, Bourinet E, Lesage F, Eschalier A, Lazdunski M, Noël J. Role of the TREK2 potassium channel in cold and warm thermosensation and in pain perception. Pain. 2014; 155:2534–2544. PMID: 25239074.


9. Acosta C, Djouhri L, Watkins R, Berry C, Bromage K, Lawson SN. TREK2 expressed selectively in IB4-binding C-fiber nociceptors hyperpolarizes their membrane potentials and limits spontaneous pain. J Neurosci. 2014; 34:1494–1509. PMID: 24453337.


10. Kollert S, Dombert B, Döring F, Wischmeyer E. Activation of TRESK channels by the inflammatory mediator lysophosphatidic acid balances nociceptive signalling. Sci Rep. 2015; 5:12548. PMID: 26224542.


11. Liu P, Xiao Z, Ren F, Guo Z, Chen Z, Zhao H, Cao YQ. Functional analysis of a migraine-associated TRESK K+ channel mutation. J Neurosci. 2013; 33:12810–12824. PMID: 23904616.
12. Mathie A, Veale EL. Two-pore domain potassium channels: potential therapeutic targets for the treatment of pain. Pflugers Arch. 2015; 467:931–943. PMID: 25420526.


13. Wolkerstorfer A, Handler N, Buschmann H. New approaches to treating pain. Bioorg Med Chem Lett. 2016; 26:1103–1119. PMID: 26774577.


14. Mika J, Zychowska M, Makuch W, Rojewska E, Przewlocka B. Neuronal and immunological basis of action of antidepressants in chronic pain - clinical and experimental studies. Pharmacol Rep. 2013; 65:1611–1621. PMID: 24553009.


15. Khouzam HR. Psychopharmacology of chronic pain: a focus on antidepressants and atypical antipsychotics. Postgrad Med. 2016; 128:323–330. PMID: 26821680.


16. Jesse CR, Wilhelm EA, Nogueira CW. Depression-like behavior and mechanical allodynia are reduced by bis selenide treatment in mice with chronic constriction injury: a comparison with fluoxetine, amitriptyline, and bupropion. Psychopharmacology (Berl). 2010; 212:513–522. PMID: 20689938.


17. Moore R, Derry S, Aldington D, Cole P, Wiffen PJ. Amitriptyline for neuropathic pain in adults. Cochrane Database Syst Rev. 2015; 7:CD008242. PMID: 26146793.


18. Gilron I, Baron R, Jensen T. Neuropathic pain: principles of diagnosis and treatment. Mayo Clin Proc. 2015; 90:532–545. PMID: 25841257.


19. Saarto T, Wiffen PJ. Antidepressants for treating neuropathic pain. Cochrane Database Syst Rev. 2007; CD005454. PMID: 17943857.
20. Nielsen JC, Bjerring P, Arendt-Nielsen L, Petterson KJ. Analgesic efficacy of immediate and sustained release paracetamol and plasma concentration of paracetamol. Double blind, placebo-controlled evaluation using painful laser stimulation. Eur J Clin Pharmacol. 1992; 42:261–264. PMID: 1577043.


21. Mehlisch DR, Sykes J. Ibuprofen blood plasma levels and onset of analgesia. Int J Clin Pract Suppl. 2013; (178):3–8. PMID: 23163542.


22. Baeck SK, Lim MA, Park SY, Lee JS, Lee HS, Koo KS. Blood concentrations of amitriptyline and its metabolite in rats after acute oral administration of amitriptyline. J Anal Toxicol. 2000; 24:271–274. PMID: 10872574.


23. Laib AK, Brünen S, Pfeifer P, Vincent P, Hiemke C. Serum concentrations of hydroxybupropion for dose optimization of depressed patients treated with bupropion. Ther Drug Monit. 2014; 36:473–479. PMID: 24452068.


24. Paulzen M, Gründer G, Veselinovic T, Wolf B, Hiemke C, Lammertz SE. Duloxetine enters the brain - But why is it not found in the cerebrospinal fluid. J Affect Disord. 2016; 189:159–163. PMID: 26437230.


25. Reis M, Chermá MD, Carlsson B, Bengtsson F. Therapeutic drug monitoring of escitalopram in an outpatient setting. Ther Drug Monit. 2007; 29:758–766. PMID: 18043473.


26. Amsterdam JD, Fawcett J, Quitkin FM, Reimherr FW, Rosenbaum JF, Michelson D, Hornig-Rohan M, Beasley CM. Fluoxetine and norfluoxetine plasma concentrations in major depression: a multicenter study. Am J Psychiatry. 1997; 154:963–969. PMID: 9210747.
27. Karson CN, Newton JE, Livingston R, Jolly JB, Cooper TB, Sprigg J, Komoroski RA. Human brain fluoxetine concentrations. J Neuropsychiatry Clin Neurosci. 1993; 5:322–329. PMID: 8369643.
28. Brueggemann LI, Mani BK, Mackie AR, Cribbs LL, Byron KL. Novel actions of nonsteroidal anti-inflammatory drugs on vascular ion channels: accounting for cardiovascular side effects and identifying new therapeutic applications. Mol Cell Pharmacol. 2010; 2:15–19. PMID: 20689646.
29. Martin DK, Schyvens CG, Wyse KR, Bursill JA, Owe-Young RA, Macdonald PS, Campbell TJ. Role of non-steroidal antiinflammatory drugs (NSAIDs) in modulating vascular smooth muscle cells by activating large-conductance potassium ion channels. Patch Clamp Technique. 2012; 14:283–300.
30. Freeman LC, Narvaez DF, McCoy A, von Stein FB, Young S, Silver K, Ganta S, Koch D, Hunter R, Gilmour RF, Lillich JD. Depolarization and decreased surface expression of K+ channels contribute to NSAID-inhibition of intestinal restitution. Biochem Pharmacol. 2007; 74:74–85. PMID: 17499219.
31. Heldestad A, Jonsborg S, Henriksson R, Grankvist K. Acetaminophen protection against estramustine-induced cytotoxicity on cultured fibroblasts. Pharmacol Toxicol. 1998; 82:128–131. PMID: 9553990.


32. Heurteaux C, Lucas G, Guy N, El Yacoubi M, Thümmler S, Peng XD, Noble F, Blondeau N, Widmann C, Borsotto M, Gobbi G, Vaugeois JM, Debonnel G, Lazdunski M. Deletion of the background potassium channel TREK-1 results in a depression-resistant phenotype. Nat Neurosci. 2006; 9:1134–1141. PMID: 16906152.


33. Gotter AL, Santarelli VP, Doran SM, Tannenbaum PL, Kraus RL, Rosahl TW, Meziane H, Montial M, Reiss DR, Wessner K, McCampbell A, Stevens J, Brunner JI, Fox SV, Uebele VN, Bayliss DA, Winrow CJ, Renger JJ. TASK-3 as a potential antidepressant target. Brain Res. 2011; 1416:69–79. PMID: 21885038.


34. Borsotto M, Veyssiere J, Moha Ou, Devader C, Mazella J, Heurteaux C. Targeting two-pore domain K+ channels TREK-1 and TASK-3 for the treatment of depression: a new therapeutic concept. Br J Pharmacol. 2015; 172:771–784. PMID: 25263033.
35. Kang D, Kim GT, Kim EJ, La JH, Lee JS, Lee ES, Park JY, Hong SG, Han J. Lamotrigine inhibits TRESK regulated by G-protein coupled receptor agonists. Biochem Biophys Res Commun. 2008; 367:609–615. PMID: 18190784.


36. Kim CW, Choe C, Kim EJ, Lee JI, Yoon SY, Cho YW, Han S, Tak HM, Han J, Kang D. Dual effects of fluoxetine on mouse early embryonic development. Toxicol Appl Pharmacol. 2012; 265:61–72. PMID: 23022515.


37. Semenchuk MR, Davis B. Efficacy of sustained-release bupropion in neuropathic pain: an open-label study. Clin J Pain. 2000; 16:6–11. PMID: 10741812.


38. Vázquez-Gómez E, Arias HR, Feuerbach D, Miranda-Morales M, Mihailescu S, Targowska-Duda KM, Jozwiak K, García-Colunga J. Bupropion-induced inhibition of α7 nicotinic acetylcholine receptors expressed in heterologous cells and neurons from dorsalraphe nucleus and hippocampus. Eur J Pharmacol. 2014; 740:103–111. PMID: 25016090.
39. Yang Y, Shimomura K, Sakuma K, Maejima Y, Iwasaki Y, Galvanovskis J, Dezaki K, Nakata M, Yada T. Bupropion can close KATP channel and induce insulin secretion. J Pediatr Endocrinol Metab. 2013; 26:343–346. PMID: 23348218.


40. Kang D, Han J, Kim D. Mechanism of inhibition of TREK-2 (K2P10.1) by the Gq-coupled M3 muscarinic receptor. Am J Physiol Cell Physiol. 2006; 291:C649–C656. PMID: 16672694.


41. Czirják G, Tóth ZE, Enyedi P. The two-pore domain K+ channel, TRESK, is activated by the cytoplasmic calcium signal through calcineurin. J Biol Chem. 2004; 279:18550–18558. PMID: 14981085.
42. Veale EL, Mathie A. Aristolochic acid, a plant extract used in the treatment of pain and linked to Balkan endemic nephropathy, is a regulator of K2P channels. Br J Pharmacol. 2016; 173:1639–1652. PMID: 26914156.


43. Bang H, Kim Y, Kim D. TREK-2, a new member of the mechanosensitive tandem-pore K+ channel family. J Biol Chem. 2000; 275:17412–17419. PMID: 10747911.
44. Kang D, Mariash E, Kim D. Functional expression of TRESK-2, a new member of the tandem-pore K+ channel family. J Biol Chem. 2004; 279:28063–28070. PMID: 15123670.
45. Rahm AK, Gierten J, Kisselbach J, Staudacher I, Staudacher K, Schweizer PA, Becker R, Katus HA, Thomas D. PKC-dependent activation of human K2P 18.1 K+ channels. Br J Pharmacol. 2012; 166:764–773. PMID: 22168364.
46. Enyedi P, Czirják G. Properties, regulation, pharmacology, andfunctions of the K2p channel, TRESK. Pflugers Arch. 2015; 467:945–945. PMID: 25366493.
47. Callejo G, Giblin JP, Gasull X. Modulation of TRESK background K+ channel by membrane stretch. PLoS One. 2013; 8:e64471. PMID: 23691227.
48. Guo D, Hu J. Spinal presynaptic inhibition in pain control. Neuroscience. 2014; 283:95–106. PMID: 25255936.


Fig. 1
Comparison of effect of analgesics on TREK-2 and TRESK.
Wholecell currents were recorded in 5 mM KCl, and the current levels at +60 mV were determined and analyzed. (A) No effect of acetaminophen, ibuprofen, and nabumetone on TREK-2 currents. (B) Inhibitory effect of acetaminophen, ibuprofen, and nabumetone on TRESK currents. Dose-response curves of analgesics are shown on the right of representative current traces. The bar graphs show the effect of analgesics on TREK-2 and TRESK currents. Data represent the mean±SD of five repeated experiments. *p<0.05 compared to the corresponding control.

Fig. 2
Inhibition of TREK-2 and TRESK currents by amitriptyline.
(A and B) Effect of amitriptyline and bupropion on TREK-2 and TRESK currents. Doseresponse curves of amitriptyline and bupropion are shown on the right of representative current traces. The bar graphs show the effect of amitriptyline and bupropion on TREK-2 and TRESK channels. Data represent the mean±SD of five repeated experiments. *p<0.05 compared to the corresponding control.
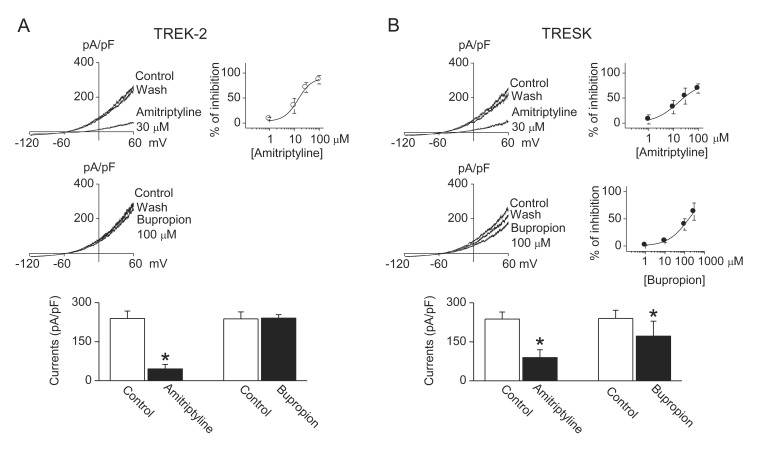
Fig. 3
Inhibition of TREK-2 and TRESK currents by SSRIs antidepressants.
(A and B) Inhibitory effect of citalopram, escitalopram, and fluoxetine on TREK- 2 and TRESK currents. Dose-response curves of SSRI antidepressants are shown on the right of representative current traces. The bar graphs show the effects of analgesics on TREK-2 and TRESK. Data represent the mean±SD of five repeated experiments. *p<0.05 compared to the corresponding control.
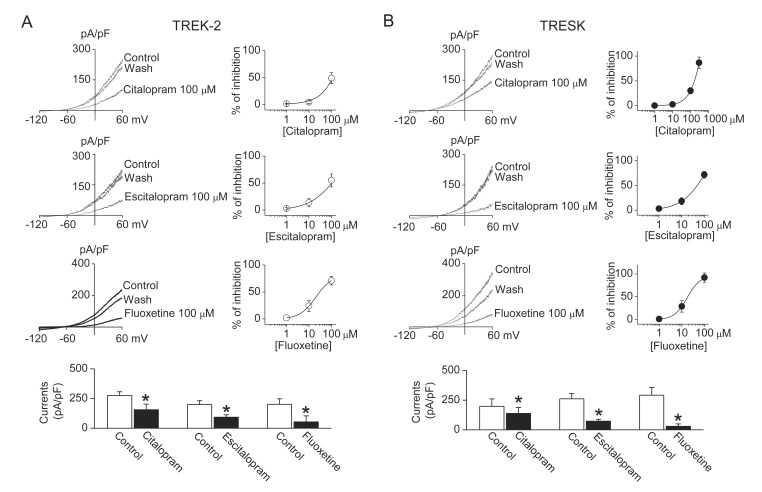