Abstract
Previously, we found that KTH-13 isolated from the butanol fraction of Cordyceps bassiana (Cb-BF) displayed anti-cancer activity. To improve its antiproliferative activity and production yield, we employed a total synthetic approach and derivatized KTH-13 to obtain chemical analogs. In this study, one KTH-13 derivative, 4-(tert-butyl)-2,6-bis(1-phenylethyl)phenol (KTH-13-t-Bu), was selected to test its anti-cancer activity. KTH-13-t-Bu diminished the proliferation of C6 glioma, MDA-MB-231, LoVo, and HCT-15 cells. KTH-13-t-Bu induced morphological changes in C6 glioma cells in a dose-dependent manner. KTH-13-t-Bu also increased the level of early apoptotic cells stained with annexin V-FITC. Furthermore, KTH-13-t-Bu increased the levels of cleaved caspase-3 and -9. In contrast, KTH-13-t-Bu upregulated the levels of pro- and cleaved forms of caspase-3, -8, and -9 and Bcl-2. Phospho-STAT3, phospho-Src, and phospho-AKT levels were also diminished by KTH13-t-Bu treatment. Therefore, these results strongly suggest that KTH-13-t-Bu can be considered a novel anti-cancer drug displaying pro-apoptotic activity.
Like Panax ginseng, Cordyceps is a genus of insect-parasitizing fungus that is used as a traditional herbal medicine in Korea and China [123]. Treatment with Cordyceps species relieves various immunological disorders including respiratory, pulmonary, and cardiovascular diseases [23]. Systemic studies have found that Cordyceps species have numerous biological activities including anti-metastatic, anti-oxidative, anti-inf lammatory, antimicrobial, anti-aging, insecticidal, hypolipidemic, hypoglycemic, and neuroprotective effects. Furthermore, Cordyceps has been considered a promising drug for treating cancer due to its antiproliferative effect [1234].
Cordyceps bassiana is a Cordyceps species that can be used as an effective herbal remedy. The butanol fraction of C. bassiana (Cb-BF) has been reported to inhibit interleukin (IL)-12 and tumor necrosis factor (TNF)-α by suppression of inflammatory signaling cascades including spleen tyrosine kinase (Syk), janus kinase (JAK)-2, and extracellular signal-regulated kinase (ERK) [5]. In addition, Cb-BF was revealed to diminish the proliferation of splenic lymphocytes, and to ameliorate various symptoms in atopic dermatitis [6]. From the same fraction, we also isolated an anti-cancer inhibitory compound with pro-apoptotic activity, KTH-13 [4-isopropyl-2,6-bis(1-phenylethyl)phenol] (Fig. 1), as well as other non-active compounds such as (E)-2-(2-(3-acetoxy-2-(acetoxymethyl)propyl)-5-(((2-hydroxyethoxy)methyl)amino)-5-oxopent-3-en-1-yl)propane-1,3-diyl diacetate (Bassiamide A), (E)-2-(15-(3-acetoxy-2-(acetoxymethyl)propyl)-5-(acetoxymethyl)-2,12-dioxo-3,6,8,11-tetraoxahexadec-13-en-16-yl)propane-1,3-diyl diacetate (Bassiamate), 1-(N-methylbenzamido)-3-(tetradecanoyloxy)propan-2-yl benzoate, and (Z)-1-acetoxy-3-(oleoyloxy)propan-2-yl 4-isopropylcyclohex-2-enecarboxylate [7]. To improve the anti-proliferative activity and production yield, we employed a total synthetic approach and further derivatized KTH-13 to obtain chemical analogs. By this process, we synthesized an analog of KTH-13, KTH-13-t-Bu [4-(tert-butyl)-2,6-bis(1-phenylethyl)phenol (Fig. 1)], as a new synthetic compound. In this study, we examined its anti-cancer activity to demonstrate the biological effect of this compound in terms of inhibiting proliferation and promoting apoptosis in various cancer cells.
KTH-13-t-Bu was supplied by Prof Lee, Yunmi (Kwangwoon University, Seoul). This compound was more than 98% pure according to HPLC analysis. (3-4,5-Dimethylthiazol-2-yl)-2,5-diphenyltetrazolium bromide, tetrazole (MTT) and bisbenzimide were purchased from Sigma Chemical Co. (St. Louis, MO, USA). Fetal bovine serum (FBS), Dulbecco's modified Eagle's medium (DMEM) and penicillin/streptomycin were obtained from Thermo Fisher Scientific Inc. (Waltham, MA, USA). C6 glioma, MDA-MB-231, LoVo, and HCT-15 cells were purchased from ATCC (Rockville, MD, USA). The FITC annexin V Apoptosis Detection Kit I was purchased from BD biosciences (San Diego, CA, USA). Antibodies against total caspases (3, 8, and 9) and phospho- and total forms of Bax, Bcl-2, STAT3, PI3K/p85, Src, and β-actin were obtained from Cell Signaling (Beverly, MA, USA).
C6 glioma, MDA-MB-231, LoVo, and HCT-15 cells were cultured in DMEM with 5% heat-inactivated FBS and 1% penicillin/streptomycin at 37℃ in 5% CO2, as reported previously [8]. For each experiment, cells were detached with trypsin/EDTA solution. The cell density used in our experiments is 5×105 cells/ml.
After pre-incubating C6 glioma, MDA-MB-231, LoVo, and HCT-15 cells (5×105 cells/ml) for 18 h, KTH-13-t-Bu was added to the cells and incubated for 6 or 24 h with 5% FBS. The effect of this compound on cell proliferation was then evaluated by using a conventional MTT assay [910]. Ten microliters of MTT solution (10 mg/ml in phosphate-buffered saline (PBS), pH 7.4) was added to the cultures, and the cells were cultured for 3 h. The incubation was stopped by adding 15% sodium dodecyl sulphate to each well to solubilize the formazan [11]. The absorbance at 570 nm (OD570~630) was assessed by using a Spectramax 250 microplate reader.
C6 glioma cells were incubated with KTH-13-t-Bu (0 to 50 µM) for the indicated times. Images of the cells in culture at each time point were obtained by using an inverted phase contrast microscope, attached to a video camera with NIH image software [12].
Apoptosis was determined with a FITC annexin V Apoptosis Detection Kit I that detects phosphatidylserine-based changes in cell membranes [1314]. Cells were plated in 12-well culture plate at a seeding density of 4×105 cells/dish (5×105 cells/ml), and KTH-13-t-Bu was added to the culture medium to the specified concentration. Vehicle alone was used as the untreated control. The subsequent procedures were conducted according to the instructions provided by the manufacturer. Briefly, at each time point, cells were harvested, washed twice with PBS and resuspended in 1X binding buffer. Annexin-V FITC and PI were added and incubated for 15 min at room temperature (25℃) in the dark. Fluorescence from 1×105 cells were detected with a BD FACScan flow cytometer (Becton Dickenson, Mountain View, CA, USA) and CellQuest Pro (IVD) software (Becton Dickenson, Mountain View, CA, USA). The assays were done in duplicate.
To assess the nuclear morphology, KTH-13-t-Bu-treated C6 glioma cells in a 12 well-plate were stained with bisbenzimide solution (Hoechst 33258; Sigma) as reported previously [1516]. Bisbenzimide (0.1 mg/ml) was dissolved in PBS/glycerol (1:1) solution. After rinsing with PBS, the cells were fixed by 4% paraformaldehyde in 0.1 M phosphate buffer and two drops of bisbenzimide solution were added. The cells were observed under a fluorescence microscope with an excitation wavelength of 365 nm.
KTH-13-t-Bu-treated C6 glioma cells (5×105 cells/ml) were washed three times in cold PBS and then lysed in lysis buffer (20 mM Tris-HCl, pH 7.4, 2 mM EDTA, 2 mM ethyleneglycotetraacetic acid, 50 mM β-glycerophosphate, 0.1 mM sodium vanadate, 1 mM dithiothreitol, 2% Triton X-100, 10% glycerol, 2 µg/ml aprotinin, 1 µg/ml pepstatin, 2 µg/ml leupeptin, 1 mM benzimide, 1.6 mM pervanadate, 20 mM NaF, and 50 µM PMSF) for 2 h on ice. The lysates were clarified by centrifugation at 12,000 × rpm for 10 min at 4℃ and then stored at -20℃ until used.
Whole cells were analyzed by immunoblotting [17]. Proteins were separated on 10% SDS-polyacrylamide gels and transferred by electroblotting onto polyvinylidenedifluoride (PVDF) membrane. Membranes were blocked for 60 min in Tris-buffered saline containing 3% FBS, 20 mM NaF, 2 mM EDTA, and 0.2% Tween-20 at room temperature. The membranes were incubated for 60 min with specific primary antibodies at 4℃, washed three times with the same buffer, and then incubated for an additional 60 min with HRP-conjugated secondary antibodies. The total and active caspases (3, 8, and 9) and total and phosphorylated Bax, Bcl-2, STAT3, PI3K/p85, Src, and β-actin were visualized with an ECL system (Amersham, Little Chalfont, Buckinghamshire, UK).
To confirm the effect of KTH-13-t-Bu as an anti-cancer compound, we tested its ability to suppress the proliferation of C6 glioma, MDA-MB-231, LoVo, and HCT-15 cells. As we expected, C6 glioma cell proliferation was dose-dependently decreased by KTH-13-t-Bu (Fig. 2A). Proliferation of other cells was also decreased by KTH-13-t-Bu in a dose-dependent manner (Fig. 2B, 2C, and 2D). Inhibitory levels (IC50 values) of this compound against the proliferation of the cancer cells were summarized in Table 1.
We next examined whether the anti-proliferative activity of KTH-13-t-Bu is derived from its apoptosis-inducing effect in C6 glioma cells. Since strong inhibitory activity of tested cells was displayed in the cells (Table 1) and we used the cell line in our previous experiments with KTH-13 derivatives [18], C6 glioma cells were selected. As shown in Fig. 3A, actin cytoskeleton-dependent morphological changes occurred after incubation with 25 µM KTH-13-t-Bu for 6 h, while 50 µM KTH-13-t-Bu induced morphological changes in 5 h. Annexin V-FITC staining, which indicates early apoptosis, dose-dependently increased from 0.86 to 12.32% (Fig. 3B). Additionally, annexin V-FITC staining increased over time from 0 to 3 h at 40 µM KTH-13-t-Bu (data not shown). As Fig. 3C shows, blebbing, cell shrinkage, and nuclear fragmentation appeared after treatment with KTH-13-t-Bu (40 µM). To identify dose-dependent effects of KTH-13-t-Bu on expression of apoptosis-related proteins, we analyzed the levels of the cleaved and pro-forms of caspase-3 and caspase-9, well-known apoptosis-inducing factors [19], as well as others (Bcl-2, which is apoptosis-preventing protein [20], and β-actin). Interestingly, it was revealed that the pro-forms of caspase-3, caspase-9, and Bcl-2 were decreased by this compound in a dose-dependent manner (Fig. 3D). In parallel, the cleaved forms of caspase-3 and -9 were increased by KTH-13-t-Bu (Fig. 3D), strongly supporting its apoptosis-inducing activity.
To confirm whether KTH-13-t-Bu affects cell survival signaling, we checked the phosphorylation levels of STAT-3, Src, and AKT, which regulate cell survival. The enzymes were chosen based on our previous finding in which KTH-13 were found to diminish the phosphorylation of these proteins without altering total proteins [18]. As expected, the levels of these phosphoproteins were clearly suppressed by 40 µM of KTH-13-t-Bu. A standard pro-apoptotic compound, staurosporin, also reduced phospho-protein levels of Src, AKT, and STAT-3 (Fig. 4A and 4B), implying that our experimental conditions were well established.
Cordyceps species were reported to exhibit anti-cancer activity [2122]. Cordyceps militaris (AECM) induced MDA-MB-231 to undergo apoptosis [23]. Cordyceps pruinosa butanol fraction (Cp-BF) inhibited proliferation and promoted apoptosis in HeLa cells [24]. Previously, we determined that KTH-13 from Cb-BF can also suppress cell growth and viability and induce apoptosis in glioma C6 and MDA-MB-231 cells [7]. We hypothesized that a KTH-13 derivative might have anti-proliferative and pro-apoptotic effects. To confirm this hypothesis, we synthesized KTH-13-t-Bu and tested whether this derivative can reduce the viability of several cancer cell lines including C6 glioma, MDA-MB-231, LoVo, and HCT-15 cells. As we expected, the viability of C6, MDA-MB-231, LoVo, and HCT-15 cells was suppressed by KTH-13-t-Bu in a dose-dependent manner similar to treatment with other natural compounds such as ginsenoside Rg3 and cordycepin [2526]. The IC50 of KTH-13-t-Bu (20 to 40 µM) is presented in Table 1. These results led us to further research its anti-cancer activity.
To investigate the anti-cancer mechanism of KTH-13-t-Bu, we determined the morphological changes in C6 glioma cells treated with KTH-13-t-Bu. Previous studies reported that actin, a cytoskeletal protein, is cleaved by caspases during cell apoptosis [27]. As shown in Fig. 3A, 25 µM KTH-13-t-Bu stimulated morphological changes in C6 glioma cells at 6 h and 50 µM KTH-13-t-Bu at 5 h. These results imply that KTH-13-t-Bu can induce apoptosis in cancer cells. To confirm this effect, we performed annexin V-FITC staining [282930]. Fig. 3B depicts the increase in annexin V-FITC staining level from 0.86 to 12.32% during KTH-13-t-Bu treatment, indicating that KTH-13-t-Bu can promote apoptosis in cancer cells. To confirm the apoptosis-inducing activity of KTH-13-t-Bu, we also assessed nuclear fragmentation by confocal microscopy with DAPI as reported previously [153132]. Fig. 3C shows that KTH-13-t-Bu induced nuclear fragmentation in C6 glioma cells.
The molecular mechanism of the pro-apoptotic effect of KTH-13-t-Bu was examined. Since active caspases have been identified as key enzymes regulating apoptosis [33], the levels of cleaved caspase proteins were detected after treatment with KTH-13-t-Bu. We chose caspase-3 and -9, since they represent mitochondrialdependent intrinsic apoptosis [34]. As shown in Fig. 3D, procaspase-3 and -9 levels decreased dose-dependently while cleaved caspase-3 and -9 increased. Bcl-2, an apoptosis inhibitor [35], also decreased in KTH-13-t-Bu-treated cells. These results indicate that KTH-13-t-Bu induces apoptosis through mitochondrialdependent intrinsic pathways.
Since apoptosis is closely linked to the down-regulation of cell survival signaling, we examined the effects of KTH-13-t-Bu on cell survival signaling. Src, PI3K, and AKT have been reported to have anti-apoptotic effects via various downstream targets, including STAT-3 [363738]. Fig. 4A shows that STAT-3 phosphorylation decreased dose-dependently in response to KTH-13-t-Bu. KTH-13-t-Bu also decreased phosphorylation of Src and AKT (Fig. 4B), which are upstream enzymes for STAT-3 activation [39]. Src phosphorylation affects Bcl-2 activity and cell apoptosis by regulating STAT-3 [40]. These results imply that KTH-13-t-Bu blocks cell survival signaling by inhibiting the Src/AKT-STAT3 cascade.
Our results strongly show that structural change of KTH-13 can give its derivatives more active in view of their anti-cancer activities. Thus, interestingly, simple change in 4-isopropyl group (IC50=64.9 µM) of ring B [18] to tert-butyl group (IC50=21.1 µM) led the compound to the enhancement of cytotoxicity up to 3-fold in C6 glioma cells (Fig. 1 and Table 1), implying that the hydrophobicity of side chain of C4 in ring B might critically contribute to the interaction of KTH-13 analogs with target protein(s). Furthermore, removal of ring C [4-isopropyl-2-(1-phenylethyl) aniline] in KTH-13 backbone has negatively affected to its anti-cancer activity [41], implying that the polarity of ring C seems to be also important to maintain its anti-cancer activity. Therefore, to further improve anti-proliferative activity of KTH-13 derivatives, additional modification of C4 side chain in ring B will be continued by introducing more hydrophobic functional groups. In addition, identification of exact target molecule toward this compound should be also continued to understand its full interpretation of drug's action. Therefore, relevant works will be preceded in the following studies.
In summary, we demonstrated that KTH-13-t-Bu can suppress cancer cell viability by inducing apoptosis, as summarized in Fig. 5. KTH-13-t-Bu induces pro-apoptotic signaling mediated by caspase and Bcl proteins. Furthermore, KTH-13-t-Bu suppressed Src/AKT-STAT3 signaling, a cell survival cascade. Because KTH-13-t-Bu shows a clear anti-cancer effect, it has the potential to be used for cancer treatment. This study implies that derivatizing compounds from C. bassiana has the potential to increase their anti-cancer activity.
ACKNOWLEDGMENTS
This work was carried out with the support of the Cooperative Research Program for Agriculture Science & Technology Development (Project no. PJ009241), Rural Development Administration, Korea.
Notes
References
1. Ng TB, Wang H. Pharmacological actions of cordyceps, a prized folk medicine. J Pharm Pharmacol. 2005; 57:1509–1519. PMID: 16354395.


2. Zhou X, Gong Z, Su Y, Lin J, Tang K. Cordyceps fungi: natural products, pharmacological functions and developmental products. J Pharm Pharmacol. 2009; 61:279–291. PMID: 19222900.


3. Holliday JC, Cleaver MP. Medicinal value of the caterpillar fungi species of the genus cordyceps (Fr.) link (Ascomycetes). A review. Int J Med Mushrooms. 2008; 10:219–234.


4. Jayakumar T, Chiu CC, Wang SH, Chou DS, Huang YK, Sheu JR. Anti-cancer effects of CME-1, a novel polysaccharide, purified from the mycelia of cordyceps sinensis against B16-F10 melanoma cells. J Cancer Res Ther. 2014; 10:43–49. PMID: 24762485.
5. Byeon SE, Lee SY, Kim AR, Lee J, Sung GH, Jang HJ, Kim TW, Park HJ, Lee SJ, Hong S, Cho JY. Inhibition of cytokine expression by a butanol extract from cordyceps bassiana. Pharmazie. 2011; 66:58–62. PMID: 21391436.
6. Wu G, Li L, Sung GH, Kim TW, Byeon SE, Cho JY, Park CW, Park HJ. Inhibition of 2, 4-dinitrofluorobenzene-induced atopic dermatitis by topical application of the butanol extract of cordyceps bassiana in NC/Nga mice. J Ethnopharmacol. 2011; 134:504–509. PMID: 21184821.
7. Antiproliferative and apoptosis-inducing activities of 4-Isopropyl-2, 6-bis (1-phenylethyl) phenol isolated from butanol fraction of cordyceps bassiana. Evid Based Complement Alternat Med. 2015; DOI: 10.1155/2015/739874.
8. Kim MY, Yoo BC, Cho JY. Ginsenoside-Rp1-induced apolipoprotein A-1 expression in the LoVo human colon cancer cell line. J Ginseng Res. 2014; 38:251–255. PMID: 25379004.


9. Twentyman PR, Luscombe M. A study of some variables in a tetrazolium dye (MTT) based assay for cell growth and chemosensitivity. Br J Cancer. 1987; 56:279–285. PMID: 3663476.


10. Kim MY, Cho JY. 20S-dihydroprotopanaxadiol, a ginsenoside derivative, boosts innate immune responses of monocytes and macrophages. J Ginseng Res. 2013; 37:293–299. PMID: 24198654.


11. Kim MY, Cho JY. 20S-dihydroprotopanaxatriol modulates functional activation of monocytes and macrophages. J Ginseng Res. 2013; 37:300–307. PMID: 24198655.


12. Kothakota S, Azuma T, Reinhard C, Klippel A, Tang J, Chu K, McGarry TJ, Kirschner MW, Koths K, Kwiatkowski DJ. Caspase-3-generated fragment of gelsolin: effector of morphological change in apoptosis. Science. 1997; 278:294–298. PMID: 9323209.


13. Satzger I, Mattern A, Kuettler U, Weinspach D, Voelker B, Kapp A, Gutzmer R. MicroRNA-15b represents an independent prognostic parameter and is correlated with tumor cell proliferation and apoptosis in malignant melanoma. Int J Caner. 2010; 126:2553–2562.


14. Chang Y, Yang ST, Liu JH, Dong E, Wang Y, Cao A, Liu Y, Wang H. In vitro toxicity evaluation of graphene oxide on A549 cells. Toxicol Lett. 2011; 200:201–210. PMID: 21130147.


15. Lee HS, Jung KK, Cho JY, Rhee MH, Hong S, Kwon M, Kim SH, Kang SY. Neuroprotective effect of curcumin is mainly mediated by blockade of microglial cell activation. Pharmazie. 2007; 62:937–942. PMID: 18214347.
16. Jang YJ, Won JH, Back MJ, Fu Z, Jang JM, Ha HC, Hong S, Chang M, Kim DK. Paraquat induces apoptosis through a mitochondria-dependent pathway in RAW264.7 cells. Biomol Ther (Seoul). 2015; 23:407–413. PMID: 26336579.


17. Kim JH, Lee YG, Yoo S, Oh J, Jeong D, Song WK, Yoo BC, Rhee MH, Park J, Cha SH, Hong S, Cho JY. Involvement of Src and the actin cytoskeleton in the antitumorigenic action of adenosine dialdehyde. Biochem Pharmacol. 2013; 85:1042–1056. PMID: 23353701.


18. Kim JH, Lee Y, Sung GH, Kim HG, Jeong D, Park JG, Baek KS, Sung NY, Yang S, Yoon DH, Lee SY, Kang H, Song C, Cho JH, Lee KH, Kim TW, Cho JY. Antiproliferative and apoptosis-inducing activities of 4-Isopropyl-2,6-bis(1-phenylethyl)phenol isolated from butanol fraction of cordyceps bassiana. Evid Based Complement Alternat Med. 2015; DOI: 10.1155/2015/739874.
19. Orrenius S. Reactive oxygen species in mitochondria-mediated cell death. Drug Metab Rev. 2007; 39:443–455. PMID: 17786631.


20. Igney FH, Krammer PH. Death and anti-death: tumour resistance to apoptosis. Nat Rev Cancer. 2002; 2:277–288. PMID: 12001989.


21. Tian T, Song L, Zheng Q, Hu X, Yu R. Induction of apoptosis by cordyceps militaris fraction in human chronic myeloid leukemia K562 cells involved with mitochondrial dysfunction. Pharmacogn Mag. 2014; 10:325–331. PMID: 25210321.


22. Ji J, Liu J, Liu H, Wang Y. Effects of fermented mushroom of cordyceps sinensis, Rich in selenium, on uterine cervix cancer. Evid Based Complement Alternat Med. 2014; DOI: 10.1155/2014/173180.
23. Shinohara K, Tomioka M, Nakano H, Tone S, Ito H, Kawashima S. Apoptosis induction resulting from proteasome inhibition. Biochem J. 1996; 317:385–388. PMID: 8713062.


24. Jin CY, Kim GY, Choi YH. Induction of apoptosis by aqueous extract of cordyceps militaris through activation of caspases and inactivation of Akt in human breast cancer MDA-MB-231 cells. J Microbiol Biotechnol. 2008; 18:1997–2003. PMID: 19131705.
25. Park EH, Kim YJ, Yamabe N, Park SH, Kim HK, Jang HJ, Kim JH, Cheon GJ, Ham J, Kang KS. Stereospecific anticancer effects of ginsenoside Rg3 epimers isolated from heat-processed American ginseng on human gastric cancer cell. J Ginseng Res. 2014; 38:22–27. PMID: 24558306.


26. Chen Y, Yang SH, Hueng DY, Syu JP, Liao CC, Wu YC. Cordycepin induces apoptosis of C6 glioma cells through the adenosine 2A receptor-p53-caspase-7-PARP pathway. Chem Biol Interact. 2014; 216:17–25. PMID: 24704558.


27. Foerster F, Braig S, Moser C, Kubisch R, Busse J, Wagner E, Schmoeckel E, Mayr D, Schmitt S, Huettel S, Zischka H, Mueller R, Vollmar AM. Targeting the actin cytoskeleton: selective antitumor action via trapping PKCε. Cell Death Dis. 2014; 5:e1398. PMID: 25165884.


28. Vermes I, Haanen C, Steffens-Nakken H, Reutellingsperger C. A novel assay for apoptosis flow cytometric detection of phosphatidylserine expression on early apoptotic cells using fluorescein labelled annexin V. J immunol methods. 1995; 184:39–51. PMID: 7622868.


29. Zhang G, Gurtu V, Kain SR, Yan G. Early detection of apoptosis using a fluorescent conjugate of annexin V. Biotechniques. 1997; 23:525–531. PMID: 9298227.


30. Wi SM, Lee KY. 5-aminoimidazole-4-carboxamide Riboside induces apoptosis through AMP-activated protein kinase-independent and NADPH oxidase-dependent pathways. Immune Netw. 2014; 14:241–248. PMID: 25360075.


31. Kim YC, Song SB, Lee SK, Park SM, Kim YS. The nuclear orphan receptor NR4A1 is involved in the apoptotic pathway induced by LPS and Simvastatin in RAW 264.7 macrophages. Immune Netw. 2014; 14:116–122. PMID: 24851101.


32. Johnson V, Ko S, Holmstrom T, Eriksson J, Chow S. Effector caspases are dispensable for the early nuclear morphological changes during chemical-induced apoptosis. J Cell Sci. 2000; 113:2941–2953. PMID: 10934034.


33. Nuñez G, Benedict MA, Hu Y, Inohara N. Caspases: the proteases of the apoptotic pathway. Oncogene. 1998; 17:3237–3245. PMID: 9916986.


34. Kim B, Srivastava SK, Kim SH. Caspase-9 as a therapeutic target for treating cancer. Expert Opin Ther Targets. 2015; 19:113–127. PMID: 25256701.


35. Oltval ZN, Milliman CL, Korsmeyer SJ. Bcl-2 heterodimerizes in vivo with a conserved homolog, bax, that accelerates programed cell death. Cell. 1993; 74:609–619. PMID: 8358790.


36. Clark AR, Toker A. Signalling specificity in the Akt pathway in breast cancer. Biochem Soc Trans. 2014; 42:1349–1355. PMID: 25233414.


37. Zhang X, Shan P, Alam J, Fu XY, Lee PJ. Carbon monoxide differentially modulates STAT1 and STAT3 and inhibits apoptosis via a phosphatidylinositol 3-kinase/Akt and p38 kinase-dependent STAT3 pathway during anoxia-reoxygenation injury. J Biol Chem. 2005; 280:8714–8721. PMID: 15590660.


38. Li H, Marshall AJ. Phosphatidylinositol (3,4) bisphosphate-specific phosphatases and effector proteins: a distinct branch of PI3K signaling. Cell Signal. 2015; 27:1789–1798. PMID: 26022180.


39. Rho O, Kim DJ, Kiguchi K, Digiovanni J. Growth factor signaling pathways as targets for prevention of epithelial carcinogenesis. Mol Carcinog. 2011; 50:264–279. PMID: 20648549.


40. Kundu J, Choi BY, Jeong CH, Kundu JK, Chun KS. Thymoquinone induces apoptosis in human colon cancer HCT116 cells through inactivation of STAT3 by blocking JAK2-and Src mediated phosphorylation of EGF receptor tyrosine kinase. Oncol Rep. 2014; 32:821–828. PMID: 24890449.
41. Kim MS, Lee Y, Sung GH, Kim JH, Park JG, Kim HG, Baek KS, Cho JH, Han J, Lee KH, Hong S, Kim JH, Cho JY. Pro-apoptotic activity of 4-Isopropyl-2-(1-Phenylethyl) Aniline isolated from Cordyceps bassiana. Biomol Ther (Seoul). 2015; 23:367–373. PMID: 26157554.


Fig. 2
Effect of KTH-13-t-Bu on proliferation of (A) C6 glioma, (B) MDA-MB-231, (C) LoVo, and (D) HCT-15 cells after treatment for 24 or 6 h.
Cell viability was determined by the MTT assay. *p<0.05 and **p<0.01 compared with the normal group.
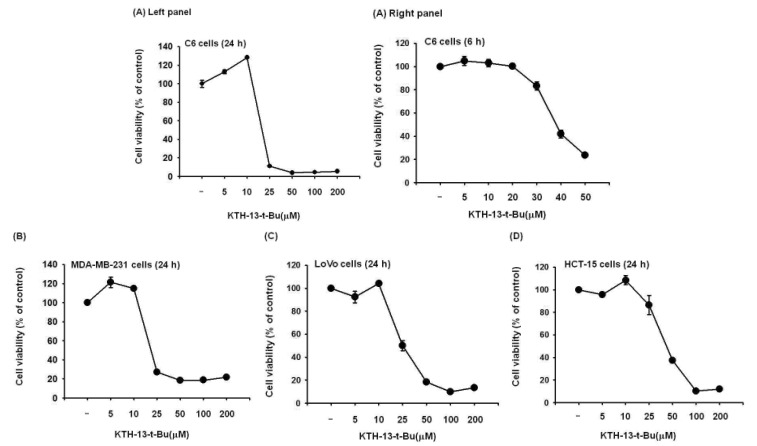
Fig. 3
Effect of KTH-13-t-Bu on inducing apoptosis in C6 glioma cells.
(A) C6 glioma cells (5×105 cells/ml) were incubated with KTH-13-t-Bu for 0, 5, and 6 h. Morphological chan ges were detected at each time point by microscopic analysis. (B) Dose-dependent pro-apoptotic effect of KTH-13-t-Bu was measured by FITC annexin V-PI staining assay. Cells were treated with annexin V, PI, and KTH-13-t-Bu (0 to 40 µM) for 6 h. Stained cells were detected by flow cytometry. (C) Changes the nuclear morphology of C6 glioma cells induced by KTH-13-t-Bu were analyzed by confocal microscopy after DAPI staining. (D) Dose-dependent upregulation of apoptosis-related proteins induced by KTH-13-t-Bu. C6 glioma cells (5×105 cells/ml) were incubated with KTH-13-t-Bu for 6 h. The levels of total and cleaved caspase 3, caspase 9, and Bcl-2 were detected by immunoblotting analysis.
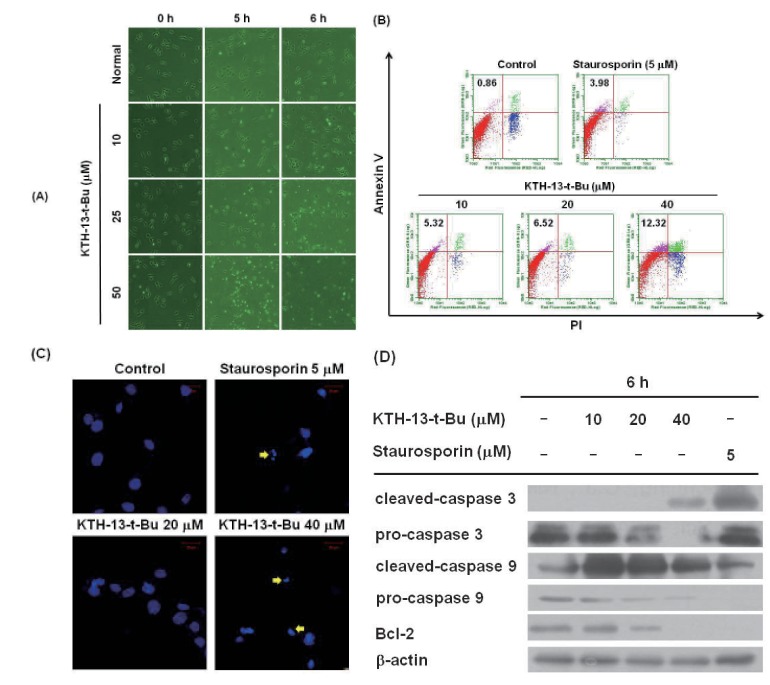