Abstract
Sertraline, a selective serotonin reuptake inhibitor (SSRI), has been reported to lead to cardiac toxicity even at therapeutic doses including sudden cardiac death and ventricular arrhythmia. And in a SSRI-independent manner, sertraline has been known to inhibit various voltage-dependent channels, which play an important role in regulation of cardiovascular system. In the present study, we investigated the action of sertraline on Kv1.5, which is one of cardiac ion channels. The eff ect of sertraline on the cloned neuronal rat Kv1.5 channels stably expressed in Chinese hamster ovary cells was investigated using the whole-cell patch-clamp technique. Sertraline reduced Kv1.5 whole-cell currents in a reversible concentration-dependent manner, with an IC50 value and a Hill coefficient of 0.71 µM and 1.29, respectively. Sertraline accelerated the decay rate of inactivation of Kv1.5 currents without modifying the kinetics of current activation. The inhibition increased steeply between –20 and 0 mV, which corresponded with the voltage range for channel opening. In the voltage range positive to +10 mV, inhibition displayed a weak voltage dependence, consistent with an electrical distance δ of 0.16. Sertraline slowed the deactivation time course, resulting in a tail crossover phenomenon when the tail currents, recorded in the presence and absence of sertraline, were superimposed. Inhibition of Kv1.5 by sertraline was use-dependent. The present results suggest that sertraline acts on Kv1.5 currents as an open-channel blocker.
Sertraline, one of selective serotonin reuptake inhibitors (SSRI), exerts its antidepressant activity by inhibiting the reuptake of 5-hydroxytryptamine (5-HT, serotonin) in the central nervous system, without critical effects on other neurotransmitter reuptake system [1]. However, several evidences suggest that sertraline possesses various biological activities that appear to be unrelated to its inhibition of serotonin reuptake and that sertraline may lead to cardiac toxicity even at therapeutic doses. It was reported that sertraline may cause sudden cardiac death and ventricular arrhythmia [23]. Furthermore, sertraline inhibits various voltage-dependent channels including Ca2+ channels, Na+ channels and K+ channels [456789]. These results suggest that sertraline may have an effect on cardiovascular system, which is highly regulated by various ion channels. And pharmacological blockade of cardiac voltage-dependent K+ channels (Kv channels) is related with various cardiac arrhythmias. Therefore, sertraline may interact with cardiac Kv channels. However, the exact actions of sertraline on cardiac Kv channels remain to be elucidated.
Kv1.5, one of the cardiovascular-specific Kv channel isoforms, Kv1.5 channels play an important role in determining the length of cardiac action potentials and, therefore, have been the targets of antiarrhythmic drugs [1011]. Kv1.5 underlie the ultrarapid delayed rectifier outward K+ currents that are observed naturally in atrium of heart, whereas they appear to be absent from ventricle [1213]. Because of its rapid activation and little inactivation, Kv1.5 can contribute to repolarization of atrial action potentials. Dysfunction of Kv1.5 results in a prolongation of cardiac action potentials, which eventually leads to cardiac arrhythmias with serious morbidity [111214].
In this study, with whole-cell patch-clamp technique, we investigated whether sertraline interacts with Kv1.5 cloned from the rat brain, and determined the mechanisms of actions of sertraline on the Kv1.5.
Chinese hamster ovary (CHO) cells expressing Kv1.5 derived from rat brain were used for electrophysiological recordings [15]. Kv1.5 cDNA [13] was transferred into the plasmid expression vector pCR3.1 (Invitrogen Corporation, San Diego, CA, USA). CHO cells were transfected with Kv1.5 cDNA using FuGENE™6 reagent (Boehringer Mannheim, Indianapolis, IN, USA). The transfected cells were cultured in Iscove's modified Dulbecco's medium (Invitrogen Corporation) supplemented with 10% fetal bovine serum, 2 mM glutamine, 0.1 mM hypoxanthine, 0.01 mM thymidine, and 300 µg/ml G418 (A.G. Scientific, San Diego, CA, USA), in 95% humidified air-5% CO2 at 37℃. The cultures were passaged every 4~5 days with a brief trypsin-EDTA treatment followed by seeding onto glass coverslips (diameter: 12 mm, Fisher Scientific, Pittsburgh, PA, USA) in a Petri dish. After 12~24 h, the cell-attached coverslips were used for electrophysiological recordings.
Kv1.5 currents were recorded from CHO cells, with a whole-cell patch-clamp technique [16] at room temperature (22~23℃). The micropipettes fabricated from glass capillary tubing (PG10165-4; World Precision Instruments, Sarasota, FL, USA) with a double-stage vertical puller (PC-10; Narishige, Tokyo) had a tip resistance of 2~3 MΩ when filled with the pipette solution. Whole-cell currents were amplified with Axopatch 200B amplifier (Molecular Devices, Sunnyvale, CA, USA), digitized with Digidata 1440A (Molecular Devices) at 5 kHz and low-pass filtered with four-pole Bessel filter at 2 kHz. Capacitive currents were canceled and series resistance was compensated at 80% with the amplifier, while leak subtraction was not used. The generation of voltage commands and acquisition of data were controlled with pClamp 10.1 software (Molecular Devices) running on an IBM-compatible Pentium computer. Recording chamber (RC-13, Warner Instrument Corporation, Hamden, CT, USA) was continuously perfused with bath solution (see below for composition) at a rate of 1 ml/min.
The intracellular pipette solution for whole-cell recordings contained 140 mM KCl, 1 mM CaCl2, 1 mM MgCl2, 10 mM HEPES, and 10 mM EGTA, and was adjusted to pH 7.3 with KOH. The bath solution for whole-cell recordings contained 140 mM NaCl, 5 mM KCl, 1.3 mM CaCl2, 1 mM MgCl2, 20 mM HEPES and 10 mM glucose, and was adjusted to pH 7.3 with NaOH. Sertraline (Sigma Chemical Co., St. Louis, MO, USA) was dissolved in dimethyl sulfoxide (DMSO) at 30 mM and further diluted into the bath solution. Final concentration of DMSO in the bath solution, which was less than 0.1%, had no effect on Kv1.5 currents (Data not shown).
Data were analyzed with Origin 7.0 (OriginLab Corp., Northampton, MA, USA) and Clampfit 10.1 software (Molecular Devices). A model of interaction kinetics between drug and channel was based on a first-order blocking scheme as previously described [17]. This scheme allowed us to obtain IC50 and Hill coefficient (n) by fitting concentration dependence data to the following equation:
in which I (%) is the percent inhibition of current (I (%) = [1-Idrug/Icontrol]×100) at test potential and [D] represents various drug concentrations. The steady-state activation curve was fitted with the Boltzmann equation:
where k represents the slope factor, V the test potential, and V1/2 the potential at which the conductance was half-maximal. An activation time constant was calculated by fitting the latter 50% of activation (i.e., rise from 50% to 100% of peak amplitude) with a single exponential function. The drug-induced time constant and deactivation time constant were determined by fitting with a single exponential function.
To investigate the voltage dependence of Kv1.5 inhibition by the drug, the relative current was plotted as a function of the membrane potential. The resultant percent inhibition data between 0 and +50 mV were fitted with a Woodhull equation [18]:
where KD(0) represents the apparent affinity at 0 mV, z the charge valence of the drug, δ the fractional electrical distance, F Faraday's constant, R the gas constant, and T the absolute temperature. A value of 25.4 mV was used for RT/F at 22℃ in the present study. Equation 6 can be modified to get a linear transformation as follows:
Results were expressed as mean±S.E.M. Student's t-test and analysis of variance (ANOVA) were used for statistical analysis with a confidence level of p<0.05.
Fig. 1 illustrates the effects of sertraline on Kv1.5 expressed in CHO cells. The superimposed-original Kv1.5 current traces were obtained by a 250-ms depolarizing pulse from –80 to +50 mV under control conditions and in the presence of various concentrations of sertraline (Fig. 1A). Under control conditions, Kv1.5 currents were activated with a fast rising to a peak and then slowly inactivated, as reported previously [15]. The dominant time constant of activation under control conditions was 1.63±0.19 ms (n=5) with a 250-ms depolarizing pulse from –80 to +50 mV. When exposed to different concentrations of sertraline (0.3, 1, 3 and 10 µM), the Kv1.5 currents were inhibited in a concentration-dependent manner. The sertraline-induced inhibition of Kv1.5 currents was characterized by an acceleration in the apparent rate of current decay in a concentration-dependent manner. In the presence of 1 µM sertraline, the time constant of activation was 1.57±0.31 ms (n=5), which indicates that the activation kinetics were not significantly modified by sertraline. Fig. 1B shows the concentration-dependent inhibition of Kv1.5 current by sertraline. Inhibition of the current was measured at the end of a 250-ms pulse of +50 mV and analyzed quantitatively. A nonlinear least-squares fit of the Hill equation to the individual data points yielded an IC50 value and a Hill coefficient of 0.71±0.01 µM and 1.29±0.04 (n=5), respectively. Sertraline-induced inhibition of Kv1.5 currents is reversible upon wash-out of the drug (Fig. 1C). The inhibition of Kv1.5 reached a steady state within 2 min and the wash-out by perfusion of drug-free solution was also reached within 2 min, and the inhibited current by 1 µM sertraline measured at the end of a depolarizing pulse of +50 mV recovered to 65.6±5.7% of control value (n=5).
Fig. 2 shows the effect of 1 µM sertraline on current-voltage (I~V) relations. Under control conditions, the Kv1.5 current activated at pulses greater than –30 mV, and the steady-state I~V relationship showed a sigmoidal shape at potentials between –30 and 0 mV (Fig. 2A and C). The inhibition of Kv1.5 currents by 1 µM sertraline was observed in the whole voltage range over which Kv1.5 was activated (Fig. 2B and C). By plotting percent inhibition (see METHODS) versus potential, a high degree of inhibition with a strong voltage dependence was observed between –20 and 0 mV, which involved the voltage range of channel opening (Fig. 2D). Between +10 and +50 mV, despite Kv1.5 being fully activated at this voltage range, inhibition continued to increase with a shallow voltage dependence: 60.9±1.3% inhibition at +10 mV, and 71.7±1.3% at +50 mV (n=4, p<0.05). Given the assumption that sertraline interacts intracellularly with Kv1.5, we investigated this effect by a linear curve fitting of the data at potentials positive to +10 mV, using a linear transformation of Woodhull equation (see METHODS). The solid lines in Fig. 2D represents a fitted curve which yielded δ=0.16±0.03 (n=4).
As shown in Fig. 3A, sertraline accelerated the decay of Kv1.5 current in a concentration-dependent manner. With a single exponential fitting to the traces of current decay at each concentration of sertraline, time constants were obtained. To avoid contamination by the time constant of the intrinsic slow and partial inactivation current of Kv1.5 under control conditions, a time constant value obtained at low concentrations (0.3 µM) of sertraline was omitted. Fig. 3B shows a summary of the time constants at various concentrations of sertraline. The values of time constant decreased as the concentration of sertraline increased, suggesting that the concentration-dependent sertraline-induced inhibition of Kv1.5 is associated with time-dependent development of block.
To further investigate the time-dependent inhibition of Kv1.5 by sertraline, deactivation kinetics of Kv1.5 current was studied. Fig. 4A shows the representative superimposed tail currents recorded with a 250-ms repolarizing pulse at –40 mV after a 250-ms depolarizing pulse of +50 mV from a holding potential of –80 mV, under control conditions and in the presence of 1 µM sertraline. These currents were well fitted to a single exponential function. Under control conditions, the tail current declined quickly with a time constant of 25.3±2.8 ms (n=4) and was nearly completely deactivated during a 250-ms repolarizing pulse of –40 mV. In the presence of 1 µM sertraline, the initial peak amplitude of the tail current was reduced and the subsequent decline of the current was slower (43.5±5.7 ms, n=4), which resulted in the tail crossover phenomenon. Fig. 4B shows a summary of the time constants obtained at repolarizing pulses of –40 mV. Sertraline significantly increased the deactivation time constants of Kv1.5 at repolarizing pulses of –40 mV (n=4, p<0.05).
Fig. 5A shows the original current traces in the absence or presence of a 2-min exposure to 1 µM sertraline, obtained after applying 20 repetitive 125-ms depolarizing pulses of +50 mV from a holding potential of –80 mV at 1 and 2 Hz. Fig. 5B shows the normalized current amplitudes at 1 and 2 Hz in the absence and presence of 1 µM sertraline during application of the pulse trains. Under control conditions, the peak amplitude of the Kv1.5 current decreased slightly by 14.6±2.3% (n=4) at 1 Hz and by 21.2±4.1% (n=4) at 2 Hz. In the presence of 1 µM sertraline, the peak amplitude of Kv1.5 decreased progressively in an use-dependent manner by 45.3±4.5% (n=4) at 1 Hz and by 64.2±7.4% (n=4) at 2 Hz. For an analysis of a frequency-dependent inhibition of Kv1.5 by sertraline without contamination of the decline of the current under control conditions, the relative currents (ISertraline/IControl) at 1 and 2 Hz were plotted (Fig. 5C). The frequency-dependent inhibition of Kv1.5 currents were detected at two frequencies, 1 and 2 Hz. These results suggest that sertraline-induced inhibition of Kv1.5 current is highly use-dependent.
The present study shows the effects of sertraline on the Kv1.5 expressed in CHO cells, using the patch-clamp technique. In this study, the characteristics of the sertraline-induced inhibition of Kv1.5 suggest that sertraline preferentially interacts with the open state of Kv1.5 based on the following lines of evidence. 1) Sertraline accelerated the rate of Kv1.5 current decay during a depolarizing pulse. This time-dependent development of block indicates that the drug preferentially interacts with the open state of Kv1.5. 2) Sertraline had no effect on the activation kinetics of Kv1.5, suggesting that sertraline does not bind to the closed or resting state of Kv1.5. 3) The inhibition of Kv1.5 induced by sertraline was highly voltage-dependent and increased steeply in the voltage range of channel activation. The voltage-dependent inhibition of Kv1.5 by sertraline implies that the inhibition of Kv1.5 by sertraline occurs preferentially after the channels are open [15192021222324]. 4) Sertraline slowed the deactivation time course, resulting in a tail crossover phenomenon. This tail crossover phenomenon suggests an interaction between sertraline and the open state of Kv1.5 [1519212425]. 5) The actions of sertraline in inhibiting Kv1.5 were use-dependent, with effects enhanced at higher rates of channel activation. One of the features of open channel blockers is a use-dependent inhibition because the blockers would have a higher chance to bind to channel pores as the channels open more frequently[151920212426]. This is consistent with the actions of sertraline on the open state of Kv1.5.
The result of voltage-dependent inhibition of Kv1.5 by sertraline shows an additional low degree of inhibition detected in the voltage range positive to +10 mV despite Kv1.5 being fully activated at this voltage range (Fig. 2). This weak but significant voltage-dependent inhibition was considered the consequence of the effects of the transmembrane electrical field on the interaction between sertraline and Kv1.5. If a positively charged drug moves into the transmembrane electric field from the inside, then inhibition should increase upon depolarization due to electrostatic repulsion between a positively charged drug and membrane depolarizing potential. This will occur in the voltage range where channels are in the opening state and should also occur over the voltage range where channels are fully activated. At an intracellular pH of 7.3 in the present study, sertraline is mainly positively charged because the drug is a weak base with a pKa=9.47 [27]. Given the assumption that sertraline interacts intracellularly with Kv1.5, the positively charged drug appears to move into the transmembrane electric field from the inside. Inhibition should then increase at more depolarizing potentials because of electrostatic repulsion between the positively charged drug and the potentials, over the voltage range where the channels are fully activated. The δ value of 0.16 for the weak but significant voltage-dependent inhibition indicates that the positively charged sertraline senses 16% of the applied transmembrane electrical field as referenced from the intracellular side. This value is similar to the δ values of 0.16~0.19 obtained in previous experiments with open channel blockers of Kv1.5 [15212223].
Although sertraline is thought to be safer than tricyclic antidepressant in the risk of cardiovascular causes, several evidences suggest that sertraline possesses various biological activities that appear to be unrelated to its inhibition of serotonin reuptake and that sertraline may lead to cardiac toxicity even at therapeutic doses. It was reported that sertraline may cause sudden cardiac death and ventricular arrhythmia [23]. In human study, 21 patients of both sexes were treated with 25~150 mg of sertraline once a day for 30 days, and the plasma levels ranged 8 nM~0.32 µM [28]. In the present study, the IC50 value of sertraline for blocking Kv1.5 is 0.71 µM. Although IC50 is higher than therapeutic plasma concentrations, significant inhibition of Kv1.5 current by 0.3 µM sertraline was detected. In this study, the actions of sertraline on Kv1.5 were examined in a CHO cell line. The phospholipid composition of the cell may be different from human native cardiac myocytes and the differences of membrane composition may affect sertraline-induced Kv1.5 inhibition. Therefore, in situations where sertraline is administered at higher doses, sertraline-induced Kv1.5 blockade might have clinical significance. In cardiomyocytes, it is most likely that sertraline may lead to cardiac disorder through block of Kv1.5 channel resulting in a significant prolongation of the action potential or affecting cardiac excitability. However, we cannot completely rule out the possibility that the effect of sertraline on Kv1.5 expressed in cell line may be different from human native cardiac myocytes. And also, open channel blocker of Kv1.5 channel can be developed as a potential antiarrhythmic drug (class III) [172930]. Thus, sertraline may be beneficially used to treat depressed patients who have arrhythmic disease. In the further study, it is highly required whether sertraline can induce the prolongation of the action potential and arrhythmic or antiarrhythmic effects in the native cardiac myocytes or animal heart.
In conclusion, the present study has described the effects of sertraline on the Kv1.5 channel. The action kinetics between sertraline and Kv1.5 suggests that sertraline is an open-channel blocker for Kv1.5 in a concentration-, voltage-, time-, and use-dependent manner. Thus, much caution about arrhythmogenic risk is required when using sertraline in the treatment with depressed patients who are suffering from a cardiovascular disease.
ACKNOWLEDGMENTS
We thank Dr. Leonard Kaczmarek (Yale University School of Medicine, USA) for the Kv1.5 cDNA. This paper was supported by research funds of Chonbuk National University in 2012.
References
1. Grimsley SR, Jann MW. Paroxetine, sertraline, and fluvoxamine: new selective serotonin reuptake inhibitors. Clin Pharm. 1992; 11:930–957. PMID: 1464219.
2. Leonard CE, Bilker WB, Newcomb C, Kimmel SE, Hennessy S. Antidepressants and the risk of sudden cardiac death and ventricular arrhythmia. Pharmacoepidemiol Drug Saf. 2011; 20:903–913. PMID: 21796718.


3. Lusetti M, Licata M, Silingardi E, Reggiani Bonetti L, Palmiere C. Cardiac toxicity in selective serotonin reuptake inhibitor users. Am J Forensic Med Pathol. 2015; 36:293–297. PMID: 26448056.


4. Aldana BI, Sitges M. Sertraline inhibits pre-synaptic Na+ channel-mediated responses in hippocampus-isolated nerve endings. J Neurochem. 2012; 121:197–205. PMID: 22288826.
5. Becker B, Morel N, Vanbellinghen AM, Lebrun P. Blockade of calcium entry in smooth muscle cells by the antidepressant imipramine. Biochem Pharmacol. 2004; 68:833–842. PMID: 15294446.


6. Lee HA, Kim KS, Hyun SA, Park SG, Kim SJ. Wide spectrum of inhibitory effects of sertraline on cardiac ion channels. Korean J Physiol Pharmacol. 2012; 16:327–332. PMID: 23118556.


7. Ohno Y, Hibino H, Lossin C, Inanobe A, Kurachi Y. Inhibition of astroglial Kir4.1 channels by selective serotonin reuptake inhibitors. Brain Res. 2007; 1178:44–51. PMID: 17920044.


8. Wang GK, Mitchell J, Wang SY. Block of persistent late Na+ currents by antidepressant sertraline and paroxetine. J Membr Biol. 2008; 222:79–90. PMID: 18418539.
9. Fonseca-Magalhães PA, Sousa DF, de Siqueira RJ, Jorge RJ, Meneses GC, Alves RS, Monteiro HS, Magalhães PJ, Martins AM. Inhibitory effects of sertraline in rat isolated perfused kidneys and in isolated ring preparations of rat arteries. J Pharm Pharmacol. 2011; 63:1186–1194. PMID: 21827491.


10. Colatsky TJ, Follmer CH, Starmer CF. Channel specificity in antiarrhythmic drug action. Mechanism of potassium channel block and its role in suppressing and aggravating cardiac arrhythmias. Circulation. 1990; 82:2235–2242. PMID: 2242545.


11. Schumacher SM, McEwen DP, Zhang L, Arendt KL, Van Genderen KM, Martens JR. Antiarrhythmic drug-induced internalization of the atrial-specific k+ channel kv1.5. Circ Res. 2009; 104:1390–1398. PMID: 19443837.
12. Wang Z, Fermini B, Nattel S. Sustained depolarization-induced outward current in human atrial myocytes. Evidence for a novel delayed rectifier K+ current similar to Kv1.5 cloned channel currents. Circ Res. 1993; 73:1061–1076. PMID: 8222078.
13. Swanson R, Marshall J, Smith JS, Williams JB, Boyle MB, Folander K, Luneau CJ, Antanavage J, Oliva C, Buhrow SA, Bennet C, Stein RB, Kaczmarek LK. Cloning and expression of cDNA and genomic clones encoding three delayed rectifier potassium channels in rat brain. Neuron. 1990; 4:929–939. PMID: 2361015.


14. Li GR, Feng J, Yue L, Carrier M, Nattel S. Evidence for two components of delayed rectifier K+ current in human ventricular myocytes. Circ Res. 1996; 78:689–696. PMID: 8635226.
15. Choi BH, Choi JS, Jeong SW, Hahn SJ, Yoon SH, Jo YH, Kim MS. Direct block by bisindolylmaleimide of rat Kv1.5 expressed in Chinese hamster ovary cells. J Pharmacol Exp Ther. 2000; 293:634–640. PMID: 10773038.
16. Hamill OP, Marty A, Neher E, Sakmann B, Sigworth FJ. Improved patch-clamp techniques for high-resolution current recording from cells and cell-free membrane patches. Pflugers Arch. 1981; 391:85–100. PMID: 6270629.


17. Snyders DJ, Yeola SW. Determinants of antiarrhythmic drug action. Electrostatic and hydrophobic components of block of the human cardiac hKv1.5 channel. Circ Res. 1995; 77:575–583. PMID: 7641327.
18. Woodhull AM. Ionic blockage of sodium channels in nerve. J Gen Physiol. 1973; 61:687–708. PMID: 4541078.


19. Delpón E, Valenzuela C, Gay P, Franqueza L, Snyders DJ, Tamargo J. Block of human cardiac Kv1.5 channels by loratadine: voltage-, time- and use-dependent block at concentrations above therapeutic levels. Cardiovasc Res. 1997; 35:341–350. PMID: 9349397.


20. Sung MJ, Hahn SJ, Choi BH. Effect of psoralen on the cloned Kv3.1 currents. Arch Pharm Res. 2009; 32:407–412. PMID: 19387585.


21. Valenzuela C, Delpón E, Franqueza L, Gay P, Pérez O, Tamargo J, Snyders DJ. Class III antiarrhythmic effects of zatebradine. Time-, state-, use-, and voltage-dependent block of hKv1.5 channels. Circulation. 1996; 94:562–570. PMID: 8759103.
22. Franqueza L, Valenzuela C, Delpón E, Longobardo M, Caballero R, Tamargo J. Effects of propafenone and 5-hydroxy-propafenone on hKv1.5 channels. Br J Pharmacol. 1998; 125:969–978. PMID: 9846634.


23. Snyders J, Knoth KM, Roberds SL, Tamkun MM. Time-, voltage-, and state-dependent block by quinidine of a cloned human cardiac potassium channel. Mol Pharmacol. 1992; 41:322–330. PMID: 1538710.
24. Choi JS, Hahn SJ, Rhie DJ, Yoon SH, Jo YH, Kim MS. Mechanism of fluoxetine block of cloned voltage-activated potassium channel Kv1.3. J Pharmacol Exp Ther. 1999; 291:1–6. PMID: 10490879.
25. Lee HM, Hahn SJ, Choi BH. Open channel block of Kv1.5 currents by citalopram. Acta Pharmacol Sin. 2010; 31:429–435. PMID: 20228830.


26. Wu J, Ding WG, Matsuura H, Tsuji K, Zang WJ, Horie M. Inhibitory actions of the phosphatidylinositol 3-kinase inhibitor LY294002 on the human Kv1.5 channel. Br J Pharmacol. 2009; 156:377–387. PMID: 19154427.


27. Valenti TW Jr, Perez-Hurtado P, Chambliss CK, Brooks BW. Aquatic toxicity of sertraline to Pimephales promelas at environmentally relevant surface water pH. Environ Toxicol Chem. 2009; 28:2685–2694. PMID: 19663538.


28. Mauri MC, Laini V, Cerveri G, Scalvini ME, Volonteri LS, Regispani F, Malvini L, Manfré S, Boscati L, Panza G. Clinical outcome and tolerability of sertraline in major depression: a study with plasma levels. Prog Neuropsychopharmacol Biol Psychiatry. 2002; 26:597–601. PMID: 11999914.
29. Aréchiga IA, Barrio-Echavarria GF, Rodríguez-Menchaca AA, Moreno-Galindo EG, Decher N, Tristani-Firouzi M, Sánchez-Chapula JA, Navarro-Polanco RA. Kv1.5 open channel block by the antiarrhythmic drug disopyramide: molecular determinants of block. J Pharmacol Sci. 2008; 108:49–55. PMID: 18818480.


30. Fedida D. Gating charge and ionic currents associated with quinidine block of human Kv1.5 delayed rectifier channels. J Physiol. 1997; 499:661–675. PMID: 9130163.


Fig. 1
Concentration-dependent inhibition of Kv1.5 by sertraline.
(A) Superimposed current traces were produced by applying 250-ms depolarizing pulses from a holding potential of –80 to +50 mV followed by a 250-ms repolarizing pulse to –40 mV every 10 s under control conditions and in the presence of 0.3, 1, 3 and 10 µM sertraline, as indicated. The dotted line represents zero current. (B) Concentration-dependent curve of inhibition by sertraline. Current amplitudes of Kv1.5 measured at the end of the depolarizing pulses were used and the percentage inhibitions were plotted against various concentrations of sertraline. The solid line is fitted to the data points by the Hill equation. Data are expressed as mean±S.E.M. (C) Representative time course for inhibition in the presence of 1 µM sertraline. The current amplitudes were measured at the end of a 250-ms depolarizing pulses from a holding potential of –80 to +50 mV every 10 s in the presence of 1 µM sertraline and normalized to the first current amplitude and the normalized data were plotted as a function of time.

Fig. 2
Voltage dependent inhibition of Kv1.5 currents by sertraline.
The Kv1.5 currents were produced by applying 250-ms pulses between –60 and +50 mV in 10-mV increments followed by a 250-ms repolarizing pulse to –40 mV every 10 s, from a holding potential of –80 mV under control conditions (A), and after the addition of 1 µM sertraline (B). The dotted lines in (A) and (B) represent zero current. (C) Resultant I~V relationships taken at the end of the test pulses in the absence (open circle) and presence (closed circle) of 1 µM sertraline. (D) Percentage current inhibition (closed square) from data in (C) was plotted against the membrane potential. For potentials positive to +10 mV, the data of percentage current inhibition was recalculated by using ln{(IControl–ISertraline)/ISertraline} (closed triangle) and replotted against membrane potential. The voltage dependence was linear fitted with equation 4 (see METHODS), shown by the solid line with the indicated values for the equivalent electrical distance (δ=0.16±0.03, n=4). The dotted line represents the activation curve of Kv1.5 under control conditions, which was obtained from a deactivating tail current amplitude at –40 mV after 250-ms depolarizing pulses to potentials between –60 to +50 mV in steps of 10 mV from a holding potentials of –80 mV and thereafter normalization using equation 2 (see METHODS).
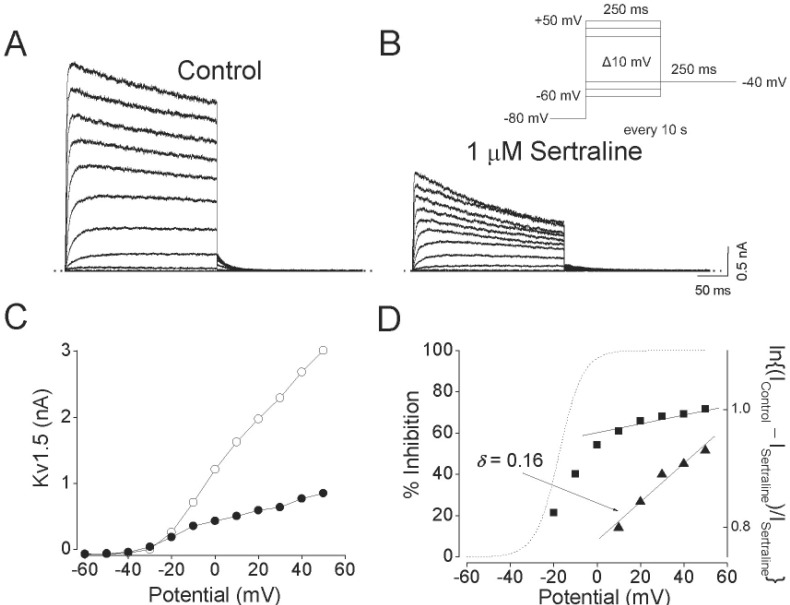
Fig. 3
Concentration-dependent kinetics of Kv1.5 inhibition by sertraline.
(A) Superimposed Kv1.5 current traces were elicited by applying 250-ms depolarizing pulses from a holding potential of –80 to +50 mV every 10 s in the presence of sertraline (1, 3, and 10 µM). The drug-induced time constants were obtained by a single exponential fitting to the decaying traces of Kv1.5 currents. The dotted line represents zero current. (B) Summary data obtained from (A). The time constants (τ) were plotted versus various concentrations of sertraline (n =4; *p<0.05 versus control data). Data are expressed as mean±S.E.M.
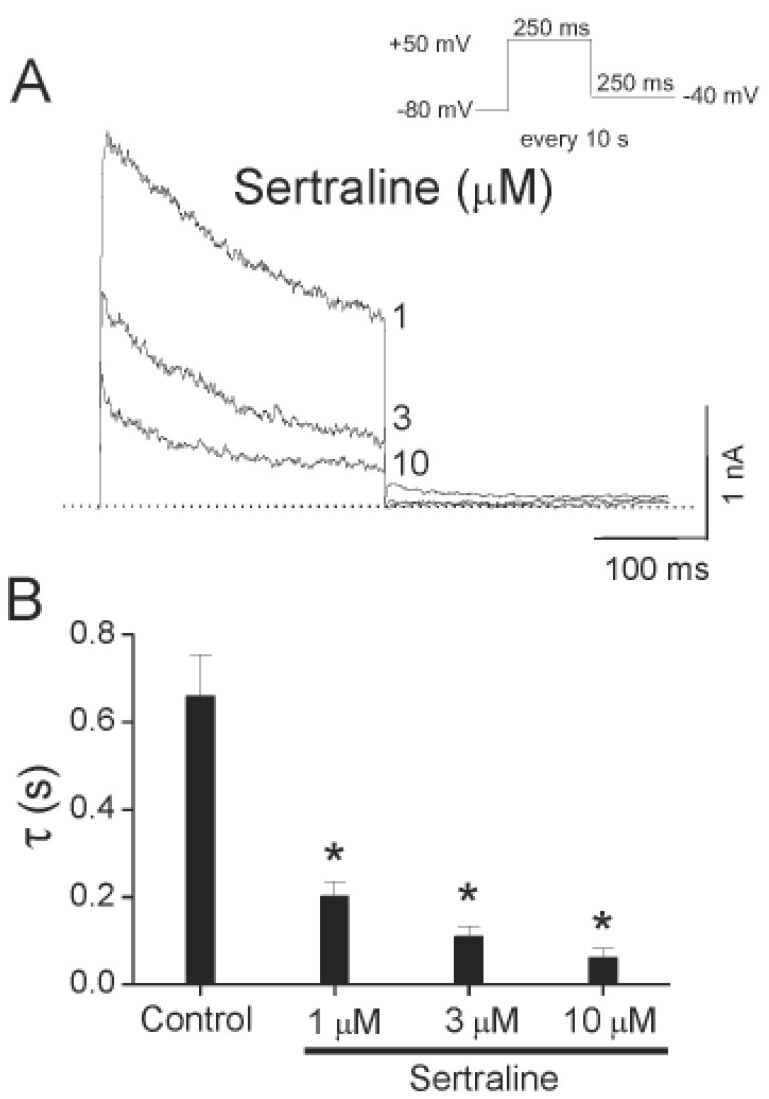
Fig. 4
Effects of sertraline on deactivation kinetics of Kv1.5.
(A) Tail currents were induced at the repolarizing pulses of –40 mV after a 250-ms depolarizing pulse of +50 mV from a holding potential of –80 mV in the absence and presence of 1 µM sertraline. The dotted lines represent a zero current. Tail crossover phenomenon (indicated by the arrow) observed by superimposing the two tail currents. The solid lines over the current traces represent the single exponential fitting to the data. (B) Deactivation time constants (π) obtained from (A). The symbol *indicates a statistically significant difference (n=4, p<0.05 versus control data). Data are expressed as mean±S.E.M.
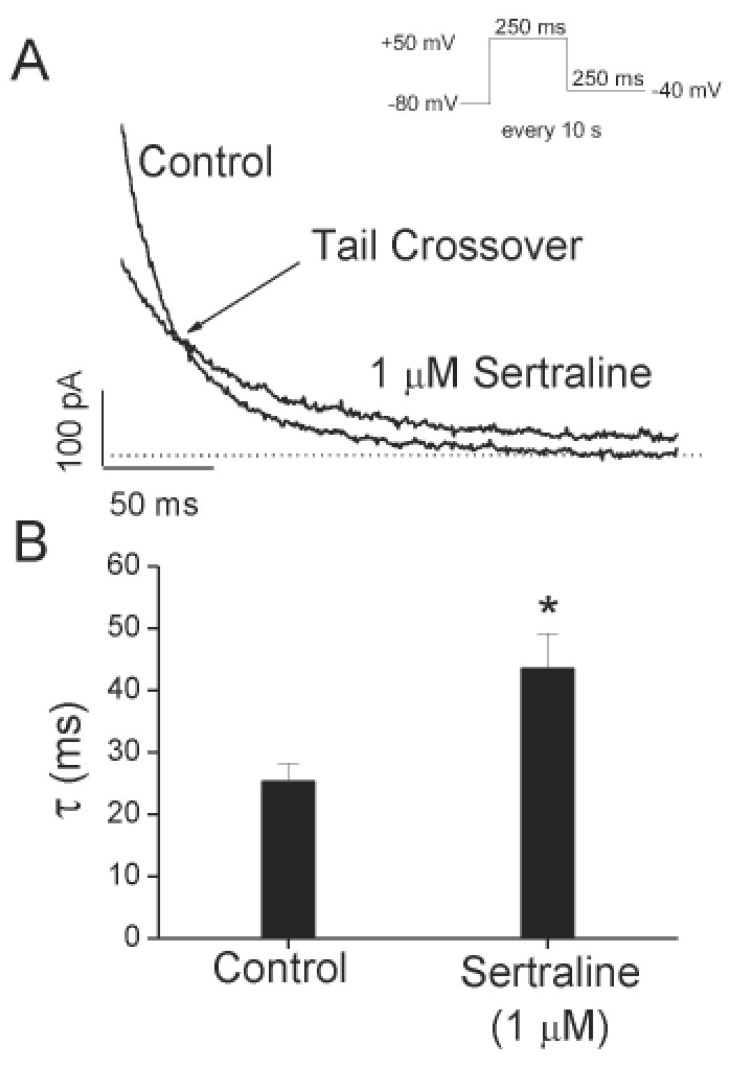
Fig. 5
Effects of repetitive depolarization on sertraline-induced inhibition of Kv1.5 currents.
(A) Original current traces under control conditions and in the presence of 1 µM sertraline obtained from applying 20 repetitive 125-ms depolarizing pulses of +50 mV from a holding potential of –80 mV at 1 or 2 Hz. The dotted lines represent a zero current. (B) Plot of normalized current at two different frequencies, 1 and 2 Hz under control conditions (open circle and open triangle, n=4) and in the presence of 1 µM sertraline (closed circle and closed triangle, n=4) as a function of the number of pulses. The peak amplitudes of the current at every pulse were normalized to the peak amplitudes of current obtained at the first pulse. (C) Relative current (ISertraline/IControl) plotted at 1 (open square) and 2 Hz (closed square) from (B) as a function of the number of pulses. Data are expressed as mean±S.E.M.
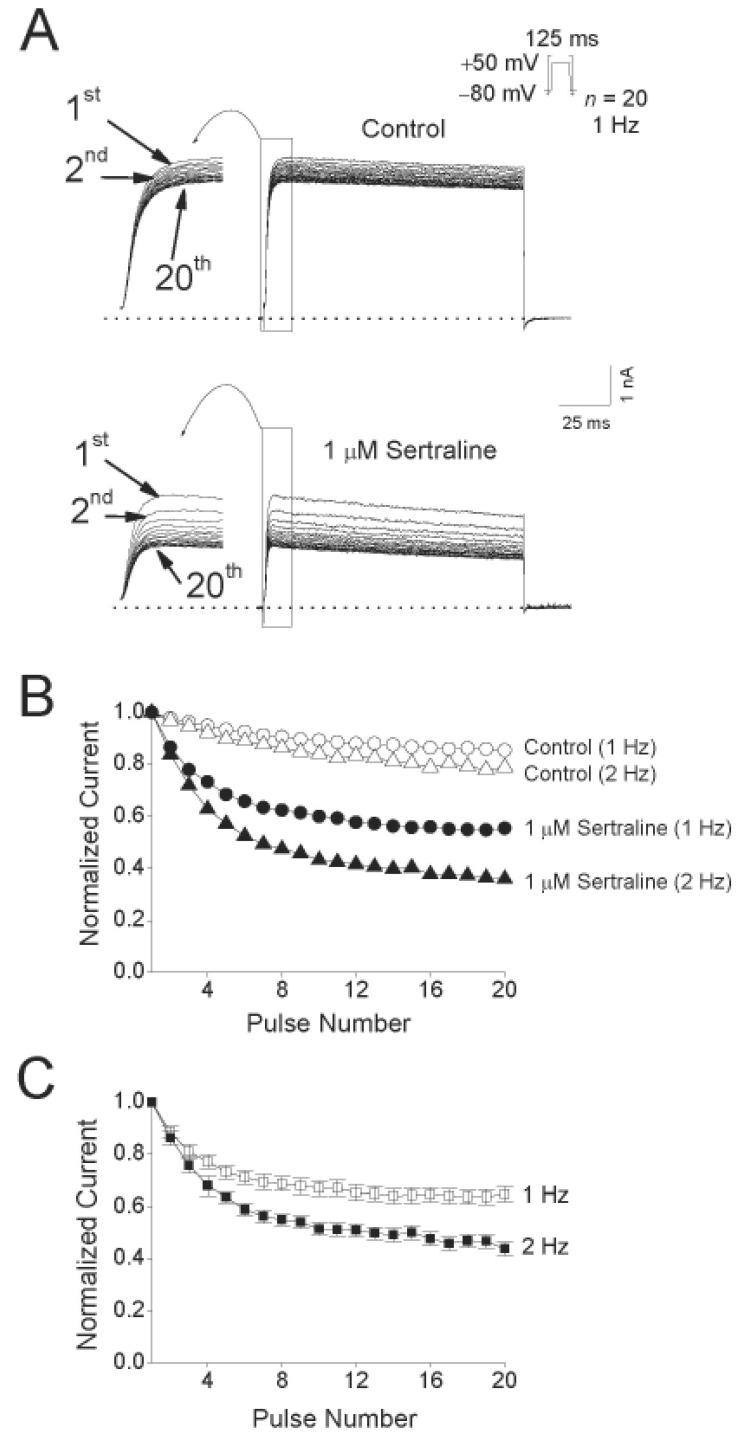