Abstract
Significant evidence supports the role of the vestibular system in the regulation of blood pressure during postural movements. In the present study, the role of the vestibulo-spino-adrenal (VSA) axis in the modulation of blood pressure via the vestibulosympathetic reflex was clarified by immunohistochemical and enzyme immunoassay methods in conscious rats with sinoaortic denervation. Expression of c-Fos protein in the intermediolateral cell column of the middle thoracic spinal regions and blood epinephrine levels were investigated, following microinjection of glutamate receptor agonists or antagonists into the medial vestibular nucleus (MVN) and/or sodium nitroprusside (SNP)-induced hypotension. Both microinjection of glutamate receptor agonists (NMDA and AMPA) into the MVN or rostral ventrolateral medullary nucleus (RVLM) and SNP-induced hypotension led to increased number of c-Fos positive neurons in the intermediolateral cell column of the middle thoracic spinal regions and increased blood epinephrine levels. Pretreatment with microinjection of glutamate receptor antagonists (MK-801 and CNQX) into the MVN or RVLM prevented the increased number of c-Fos positive neurons resulting from SNP-induced hypotension, and reversed the increased blood epinephrine levels. These results indicate that the VSA axis may be a key component of the pathway used by the vestibulosympathetic reflex to maintain blood pressure during postural movements.
The homeostatic control of blood pressure is mediated through the baroreceptor reflex. This feedback control system maintains arterial blood pressure during hemorrhage, postural changes, and exercise. Signals from the baroreceptors are conveyed by branches of the glossopharyngeal and vagus nerves toward the nucleus tractus solitarius (NTS). They are then sent to the caudal ventrolateral medullary nucleus (CVLM) and the nucleus ambiguus. The CVLM projects inhibitory neurons to the rostral ventrolateral medullary nucleus (RVLM), an area containing presympathetic neurons that innervate the intermediolateral cell column in the spinal cord [12]. The vasomotor RVLM neurons receive GABAergic input from the CVLM that tonically inhibits bulbospinal neurons [3]. However, it should be noted that the RVLM integrates both the baroreceptor and vestibular inputs that constitute the sympathetic nerve circuitry [4567].
In all mammalian species, the vestibular system plays an important role in controlling blood pressure and respiration during postural changes through the vestibulosympathetic reflex [8]. Excitation of the peripheral vestibular system induces functional modulation of the cardiovascular system, including changes in blood pressure, pulse rate, and blood flow to the extremities [9]. Electrical or selective natural stimulation of peripheral vestibular receptors induces an increase in sympathetic nerve activity [810]. In humans, caloric vestibular stimulation also increases sympathetic nerve activity [11], and stimulation of the otolith organs by linear acceleration causes transient changes in systolic blood pressure. These changes are attenuated in patients with bilateral vestibular lesions [1213]. Galvanic vestibular stimulation also significantly increases muscle sympathetic nerve activity [1415]. Thus, data from both experimental animals and humans indicate that the vestibular system contributes to the sympathetic control of blood pressure.
The caudal portion of the medial vestibular nucleus (MVN) and the inferior vestibular nucleus receive afferent signals from the otolith organs as well as the semicircular canals. Both nuclei project to the caudal brainstem sites that are involved in the regulation of sympathetic outflow, including the NTS and RVLM [1617]. Vestibulosympathetic neural pathways have been identified electrophysiologically in the muscle and renal sympathetic nerves. Central vestibular neurons mediating the vestibulosympathetic reflex have also been demonstrated [18].
Humoral factors as well as neurogenic factors play an important role in the regulatory control of blood pressure. For example, it is well known that epinephrine released from the adrenal gland strongly influences the control of blood pressure [19]. It has been previously postulated that the modulation of blood pressure by the vestibular system can be mediated by the adrenal gland as well as by the sympathetically-mediated release of vasoconstrictive substances. Nevertheless, a relationship between activation of the vestibular system and secretion of epinephrine has not yet been demonstrated.
Previous studies in our laboratory have shown that sodium nitroprusside (SNP)-induced hypotension increases neuronal activity, expression of c-Fos protein, and glutamate release in the MVN and the RVLM [2021222324]. This indicates that SNP-induced hypotension influences the activity of neurons in the MVN by increasing afferent signals from the peripheral vestibular receptors. And afferent signals from the peripheral vestibular receptors following hypotension are transmitted to the RVLM through the MVN. Therefore, it is postulated that SNP-induced hypotension may produce the vestibulosympathetic reflex by activation of the sympathetic nerves through the intermediolateral cell column in the spinal cord and secretion of epinephrine in the adrenal gland. However, systemic research on the vestibulo-spino-adrenal (VSA) projections has not been conducted.
It is likely that the VSA projections can be incorporated as part of the basic strategy in the mechanistic treatment of orthostatic hypotension. In the current study, we directly tested the functional connectivity of the VSA projections in the modulation of blood pressure via the vestibulosympathetic reflex with the use of immunohistochemical and enzyme immunoassay methods. c-Fos protein, which is expressed in response to a variety of stimuli in neurons of the central nervous system and is considered a useful marker for detecting changes in neuronal activity [25], was measured in the spinal cord following SNP-induced hypotension. Immunohistochemical data were used to delineate functional connections in the vestibulo-bulbo-spinal pathway between the vestibular nuclei and the spinal cord. Blood epinephrine levels were also measured following SNP-induced hypotension to investigate the extent of functional connectivity involved in the vestibulosympathetic reflex between the vestibular nuclei and the adrenal gland. Further, functional connections between the RVLM and the spinal cord were measured to clarify the sympathetic projections. Since the expression of c-Fos protein in the spinal cord is known to be strongly affected by anesthesia [26], conscious animals were used during all experiments. Sinoaortic denervation (SAD) was performed to eliminate the influence of the baroreceptor reflex following hypotension.
Adult male Sprague-Dawley rats (Changchun, China) weighing 220~250 g were used. All animal protocols and procedures described were performed in accordance with the rules and regulations of the Animal Research Committee of the Yanbien University. The animals had free access to food and water. All possible efforts were made to minimize the number of animals used and suffering. The rats were divided into the MVN group and the RVLM group, and each group was subdivided into six groups for immunohistochemical analysis and epinephrine detection (n=7 per group): ACSF, NMDA, AMPA, ACSF+SNP, MK-801+SNP, and CNQX+SNP groups. All rats had previously undergone SAD surgery, and all experimental observations were conducted in conscious animals.
Under isoflurane anesthesia (Ilsung Co., Seoul, Korea), the carotid sinus nerve was sectioned bilaterally following a midventral incision in the neck. The internal, external, and common carotid arteries were stripped of connective tissue at the level of the bifurcation and painted with 10% phenolethanol in order to denervate the carotid sinus. For aortic arch denervation, the aortic arch nerve was severed bilaterally proximal to its junction with the vagus nerve [27]. After the surgery, the animals breathed spontaneously without significant changes in their respiratory rhythm. SAD was performed 24 h prior to experimentation.
The rats were anesthetized with isoflurane and placed in a stereotaxic frame (Narishige, Tokyo, Japan). In the MVN group, the skull was exposed and a trocar sheath for microinjection (Terumo, Tokyo, Japan) was stereotaxically implanted into the left MVN according to a rat atlas [28]: antero-posterior, -12.3 mm from bregma; latero-medial, 0.6 mm from midline; and dorso-ventral, 5.6 mm from skull. In the RVLM group, a trocar sheath for microinjection was implanted into the left RVLM: antero-posterior, -12.7 mm from bregma; latero-medial, 2.4 mm from midline; and dorso-ventral, 7.3 mm from skull. ACSF, NMDA, AMPA, MK-801, or CNQX was injected into the MVN or RVLM at a concentration of 1 mmol/L and a volume of 10 µL. Microinjections were administered by a micro-amount thruster (Harvard Apparatus, Holliston, MA, USA), and all rats were conscious at the time of injection. Ten minutes after microinjection of drugs, hypotension was induced by infusion of SNP. After experimentation, the microinjection site was confirmed in the MVN or RVLM by histological examinations according to a rat atlas [28].
Under isoflurane anesthesia, two heparinized polyethylene tubes were inserted into the femoral artery for the recording of blood pressure and into the femoral vein for SNP infusion. The tubes were guided toward the skull percutaneously, fixed to the skull, and connected to the tubes of a cybernation metabolism cage to allow free movement during the experiment. Blood pressure was recorded from the femoral artery using a pressure transducer and physiological recorder (Grass model 7400, Grass Technologies, Warwick, RI, USA). SNP was infused over 3 min at a dose of 15 µg/(kg·min). This resulted in a blood pressure decrease of 30~40 mmHg during this period.
Immunohistochemical analysis of c-Fos protein expression was performed as described previously [2930]. Deep anesthesia was induced with an overdose of sodium pentobarbital 90 min after MVN or RVLM microinjection of glutamate agonists or infusion of SNP, and the animals were perfused transcardially with 500 mL of 0.9% NaCl at 4℃ followed by 500 mL of 4% paraformaldehyde dissolved in 0.1 M phosphate buffer (pH 7.4) at 4℃. The spinal cord was dissected and post-fixed with paraformaldehyde fixative solution for 4 h at room temperature. The fixed spinal cord was then cryoprotected by incubation in 30% sucrose in phosphate-buffered saline (PBS) for 2 d at 4℃. Frozen tissue sections of 20 µm thickness were obtained using a microtome (Leica; Nubloch, Germany). These were then incubated for 30 min in 0.3% H2O2, rinsed three times for 5 min with 0.01 M PBS, and incubated in 1% Triton X-100 for 30 min. After a brief wash, the tissues were incubated for 30 min with PBS containing 5% bovine serum albumin (PBS+BSA) followed by an overnight incubation at 4℃ with rabbit polyclonal anti-c-Fos antibody (1 : 400; Cell Signaling Technology, Danvers, MA, USA) in PBS + BSA. The following day, tissue sections were incubated with a biotinylated goat anti-rabbit secondary antibody (1 : 200; Vector Labs; Burlingame, CA, USA) and then with the Elite ABC Kit (Vector Labs) for 2 h at 37℃. The bound complex was visualized by incubating the tissue with diaminobenzidine. Finally, sections were dehydrated, dipped in xylene, and mounted with a coverslip using Permount (Fisher Scientific; Pittsburgh, PA, USA). The c-Fos-positive neurons in the T4-T7 spinal cord region were counted using a digital image analysis system (Image-Pro; Media Cybernetics, Silver Spring, MD, USA) at four different levels in a rostral-to-caudal continuum.
Blood samples were obtained from the femoral artery immediately after MVN or RVLM microinjection of glutamate agonists or SNP infusion, and collected in polyethylene tubes containing ethylenediaminetetraacetic acid as an anti-coagulant. Samples were centrifuged at 3000 rpm for 10 min at 4℃. The supernatant was then aspirated and frozen immediately at -25℃. The analysis of epinephrine secretion was performed by enzyme-linked immunosorbent assay (ELISA) according to the manufacturer's instructions (TSZ Scientific LLC, Lexington, MA, USA). Both the intraand inter-assay variabilities were lower than 10%.
Stat View version 11.5 software for SPSS (SPSS Inc, USA) was used for all statistical procedures. All data are expressed as the mean±standard deviation of the mean (SD). Differences were identified using multivariate analyses of variance. A significance level of p<0.05 was used in all experiments.
The intermediolateral cell column in the T4-T7 spinal cord region did not show any expression of c-Fos protein under resting conditions in rats with intact baroreceptors and vestibular receptors. In addition, rats with SAD did not show any expression of c-Fos protein in the spinal cord under resting conditions. Expression of c-Fos protein in the intermediolateral cell column of the T4-T7 spinal cord was measured following drug modulation of MVN activity, in order to measure the functional connectivity between the MVN and the spinal cord. The number of c-Fos positive neurons in the intermediolateral cell column of the spinal cord was 2.2±1.0 in rats after microinjection of artificial cerebrospinal fluid (ACSF) into the MVN. However, microinjection of glutamate receptor agonists significantly increased the number of c-Fos positive neurons in the spinal cord as compared to ACSF microinjection. N-methyl-D-aspartic acid (NMDA) increased the number of c-Fos positive neurons to 20.5±2.2, and 2-amino-3-(5-methyl-3-oxo-1,2-oxazol-4-yl) propanoic acid (AMPA) increased the number of c-Fos positive neurons to 21.8±1.9. The effect of glutamate receptor antagonists on the expression of c-Fos protein in the spinal cord was also investigated in rats with SNP-induced hypotension. SNP-induced hypotension increased the number of c-Fos positive neurons in the spinal cord to 27.0±3.9 as compared to the normotensive rat levels of 2.2±1.0, with both groups receiving microinjection of ACSF into the MVN. The effects of two glutamate receptor antagonists, (+)-5-methyl-10,11-dihydro-5H-dibenzo[a,d] cyclohepten-5,10-imine maleate (MK801) and 6-cyano-7-nitroquinoxaline-2,3-dione (CNQX), were then tested. Pretreatment with microinjection of either MK801 or CNQX into the MVN significantly decreased the number of c-Fos positive neurons in the spinal cord to 8.7±6.0 or 3.7±2.4, respectively, after SNP-induced hypotension as compared to the ACSF+SNP group (Fig. 1 and 2).
The functional connectivity between the RVLM and the spinal cord was measured following drug modulation of RVLM activity. The number of c-Fos positive neurons in the intermediolateral cell column of spinal cord was 6.3±0.2 after microinjection of ACSF into the RVLM. However, microinjection of a glutamate receptor agonist, NMDA or AMPA, into the RVLM significantly increased the number of c-Fos positive neurons to 35.8±4.4 or 39.5±2.4, respectively, as compared to the ACSF microinjection. After microinjection of ACSF into the RVLM, SNP-induced hypotension increased the number of c-Fos positive neurons in the spinal cord to 37.2±4.7 as compared to the normotensive rat levels of 6.3±0.2. Pretreatment with microinjection of a glutamate receptor antagonist, MK801 or CNQX, into the RVLM significantly decreased the number of c-Fos positive neurons to 8.3±6.6 or 7.2±1.6, respectively, after SNP-induced hypotension as compared to the ACSF + SNP group (Fig. 3 and 4).
Secretion of epinephrine was measured following drug modulation of MVN activity in order to measure the functional connectivity between the MVN and the adrenal gland in the control of blood pressure by the vestibular system. Microinjection of glutamate receptor agonists into the MVN was used to induce epinephrine secretion. In rats microinjected with ACSF into the MVN, the level of epinephrine secretion was 77.8±3.0 pg/mL. However, microinjection of glutamate receptor agonists into the MVN significantly increased the epinephrine secretion as compared to ACSF microinjection, with NMDA increasing the levels of epinephrine to 88.2±5.0 pg/mL and AMPA increasing the levels to 97.5±8.3 pg/mL. The effect of glutamate receptor antagonists on epinephrine secretion was also investigated following SNP-induced hypotension. SNP-induced hypotension resulted in increased epinephrine secretion in rats receiving microinjection of ACSF into the MVN when compared to the normotensive rats receiving microinjection of ACSF. Pretreatment with microinjection of ACSF into the MVN raised epinephrine secretion to 91.6±11.2 pg/mL following SNP-induced hypotension, which was higher than the secretion in normotensive rats (77.8±3.0 pg/mL). However, pretreatment with microinjection of glutamate receptor antagonists significantly decreased epinephrine secretion following acute hypotension compared to microinjection of ACSF. Pretreatment with microinjection of MK-801 decreased epinephrine secretion following SNP-induced hypotension to 79.4±7.6 pg/mL, and CNQX decreased it to 72.6±1.2 pg/mL (Fig. 5A).
The basal range of systolic and diastolic blood pressure was 103~116 mmHg and 72~84 mmHg, respectively, before drug treatment. However, microinjection of glutamate receptor agonists into the MVN increased blood pressure as compared to control levels (Fig. 6).
Microinjection of ACSF into the RVLM induced epinephrine secretion at a level of 75.1±2.9 pg/mL. However, microinjection of glutamate receptor agonists into the RVLM significantly increased the epinephrine secretion as compared to ACSF microinjection, with NMDA increasing the levels of epinephrine to 81.9±1.9 pg/mL and AMPA increasing the levels to 92.2±1.3 pg/mL. SNP-induced hypotension resulted in increased epinephrine secretion in rats receiving microinjection of ACSF into the MVN when compared to the normotensive rats receiving microinjection of ACSF. SNP-induced hypotension after pretreatment with ACSF into the RVLM raised epinephrine secretion to 92.5±3.0 pg/mL, which was higher than the secretion in normotensive rats (75.1±2.9 pg/mL). However, pretreatment with microinjection of glutamate receptor antagonists into the RVLM significantly decreased epinephrine secretion following SNP-induced hypotension compared to pretreatment with ACSF. Pretreatment with microinjection of MK-801 decreased epinephrine secretion to 82.1±1.8 pg/mL, and CNQX decreased it to 78.7±4.2 pg/mL (Fig. 5B).
The major source of descending drive to the sympathetic preganglionic neurons of the spinal cord is the spinally-projecting neurons in the RVLM [31]. Excitation of the RVLM increases blood pressure and sympathetic vasomotor tone, whereas inhibition of the RVLM decreases blood pressure. The activity in the bulbospinal sympathoexcitatory pathways [32] is modulated by afferent signals from both the baroreceptors and vestibular receptors. Since glutamate is a major excitatory neurotransmitter in the vestibular nuclei, microinjection of glutamate receptor agonists into the MVN causes excitation of the vestibular nuclear neurons [33]. The MVN sends direct projections to the RVLM, which is involved in the regulation of sympathetic outflow [1734]. In turn, the RVLM sends excitatory projections to the sympathetic pre-ganglionic neurons in the intermediolateral cell column of the spinal cord [1]. Therefore, stimulation of the MVN may produce excitation of the sympathetic preganglionic neurons in the spinal cord through the bulbospinal sympathoexcitatory pathways (Fig. 7).
In our previous studies, SNP-induced hypotension increased neuronal activity and glutamate release in the MVN [21222324]. Moreover, connections between the vestibular system and the RVLM following SNP-induced hypotension in SAD animals were investigated [20]. In the current study, SNP-induced hypotension increased the expression of c-Fos protein in the spinal cord, which is consistent with the effects of glutamate receptor agonist microinjection into the MVN. That is, microinjection of glutamate receptor agonists, NMDA and AMPA, into the MVN increased expression of c-Fos protein in the intermediolateral cell column of the middle thoracic spinal regions. This region provides the major source of input to the adrenal gland [35]. Minson et al. [36] reported that SNP-induced hypotension increased the expression of c-Fos protein in the RVLM and spinal cord. They demonstrated the bulbospinal projections immunohistochemically, following unloading of the baroreceptors by SNP-induced hypotension, but they did not describe the role of the vestibular system in sympathetic modulation following hypotension. SNP-induced hypotension produces excitation of the MVN neurons, and then this excitatory signal is transmitted to the sympathetic preganglionic neurons in the spinal cord through the RVLM, constituting the vestibulo-bulbo-spinal projections. Interestingly, pretreatment with microinjection of glutamate receptor antagonists, MK-801 and CNQX, into the MVN prevented the hypotension-induced excitation of MVN neurons. This resulted in decreased expression of c-Fos protein in the spinal cord following SNP-induced hypotension.
c-Fos-positive neurons in the intermediolateral cell column of the middle thoracic spinal regions are mainly sympathoadrenal neurons [35]. Epinephrine is released from the chromaffin cells of the adrenal medulla. The increased c-Fos protein expression in the sympathoadrenal neurons induced by hypotension suggests that epinephrine is likely released in response to hypotension. This result is consistent with a previous study, in which elevated plasma epinephrine concentrations were associated with the activation of sympathoadrenal neurons as revealed by c-Fos immunoreactivity [37]. Microinjection of glutamate receptor agonists, NMDA and AMPA, into the MVN increased blood levels of epinephrine. SNP-induced hypotension also increased blood levels of epinephrine; however, pretreatment with microinjection of glutamate receptor antagonists, MK-801 and CNQX, into the MVN abolished this increased epinephrine secretion. Moreover, blood pressure was increased following microinjection of glutamate receptor agonists into the MVN, which was different from our previous study [38]. The difference is likely related to the dose of glutamate receptor agonists which was injected into the MVN. These results suggest that the functional connections between the vestibular nuclei and the adrenal gland maintain blood pressure following hypotension. The response pattern of epinephrine secretion by stimulation of the MVN was consistent with the expression of c-Fos protein in the spinal cord. Moreover, the response pattern of both c-Fos protein expression and epinephrine secretion in the MVN group was very similar to that in the RVLM group, which provides indirect evidence that the vestibulospinal sympathetic pathway is mediated via the RVLM. Taken together, our results and the literature propose that the VSA axis maintains blood pressure following hypotension. Furthermore, the neural connections involved are mediated by the glutamatergic NMDA and AMPA receptors.
Although the involvement of the VSA axis in the vestibulosympathetic reflex represents an acute response pathway, secretion of epinephrine from the adrenal gland must involve a time delay in order to control blood pressure during postural movements. Adequate control of blood pressure by the vestibulosympathetic reflex would hence require involvement of both the neurogenic and humoral pathways. The neurogenic pathway of the vestibulosympathetic reflex on blood pressure control involves the direct stimulation of blood vessels via sympathetic nerves such as the renal nerve, muscle vasoconstrictor efferents, and nerves to the extremities [1439]. Electrical stimulation of the vestibular nerve elicits a patterning of sympathetic nerve activity, and can lead to distinct immediate changes in local blood flow. On the other hand, the humoral pathway is mediated by epinephrine secretion from the adrenal gland; it produces a delayed response on blood pressure as compared to the neurogenic pathway. Therefore, the vestibular system can modulate blood pressure through both the adrenal gland and the sympathetic nerves.
In summary, both microinjection of glutamate receptor agonists into the MVN or RVLM and SNP-induced hypotension increase the number of c-Fos positive neurons in the intermediolateral cell column of the middle thoracic regions. Pretreatment with microinjection of glutamate receptor antagonists into the MVN or RVLM can prevent the increased number of c-Fos positive neurons following SNP-induced hypotension. These results are consistent with the existence of functional connections between the vestibular nuclei and the sympathetic preganglionic nerves, known as the vestibulospinal sympathetic pathway. Both microinjection of glutamate receptor agonists into the MVN or RVLM and SNP-induced hypotension increase circulating epinephrine levels, and this is prevented by pretreatment with microinjection of glutamate receptor antagonists into the MVN or RVLM. This further indicates the presence of functional connections between the vestibular nuclei and the adrenal gland. Hence, the VSA axis is proposed to be a key component of the pathway used by the vestibulosympathetic reflex to maintain blood pressure during postural movements. Further research is required to determine whether other nuclei groups also participate in this neural pathway.
References
1. Guyenet PG. The sympathetic control of blood pressure. Nat Rev Neurosci. 2006; 7:335–346. PMID: 16760914.


2. Pilowsky PM, Goodchild AK. Baroreceptor reflex pathways and neurotransmitters: 10 years on. J Hypertens. 2002; 20:1675–1688. PMID: 12195099.


3. Schreihofer AM, Ito S, Sved AF. Brain stem control of arterial pressure in chronic arterial baroreceptor-denervated rats. Am J Physiol Regul Integr Comp Physiol. 2005; 289:R1746–R1755. PMID: 16123230.


4. Holstein GR, Friedrich VL Jr, Kang T, Kukielka E, Martinelli GP. Direct projections from the caudal vestibular nuclei to the ventrolateral medulla in the rat. Neuroscience. 2011; 175:104–117. PMID: 21163335.


5. Lan Y, Yang YZ, Jiang X, Li LW, Jin GS, Kim MS, Park BR, Jin YZ. Additive role of the vestibular end organ and baroreceptors on the regulation of blood pressure in rats. Korean J Physiol Pharmacol. 2013; 17:367–373. PMID: 23946697.


6. Yates BJ, Yamagata Y, Bolton PS. The ventrolateral medulla of the cat mediates vestibulosympathetic reflexes. Brain Res. 1991; 552:265–272. PMID: 1913189.


7. Yates BJ, Miller AD. Properties of sympathetic reflexes elicited by natural vestibular stimulation: implications for cardiovascular control. J Neurophysiol. 1994; 71:2087–2092. PMID: 7931504.


8. Yates BJ. Vestibular influences on the sympathetic nervous system. Brain Res Brain Res Rev. 1992; 17:51–59. PMID: 1638275.


9. Normand H, Etard O, Denise P. Otolithic and tonic neck receptors control of limb blood flow in humans. J Appl Physiol (1985). 1997; 82:1734–1738. PMID: 9173934.
10. Kerman IA, Yates BJ, McAllen RM. Anatomic patterning in the expression of vestibulosympathetic reflexes. Am J Physiol Regul Integr Comp Physiol. 2000; 279:R109–R117. PMID: 10896871.


11. Cui J, Mukai C, Iwase S, Sawasaki N, Kitazawa H, Mano T, Sugiyama Y, Wada Y. Response to vestibular stimulation of sympathetic outflow to muscle in humans. J Auton Nerv Syst. 1997; 66:154–162. PMID: 9406120.


12. Cui J, Iwase S, Mano T, Kitazawa H. Responses of sympathetic outflow to skin during caloric stimulation in humans. Am J Physiol. 1999; 276:R738–R744. PMID: 10070134.
13. Yates BJ, Aoki M, Burchill P, Bronstein AM, Gresty MA. Cardiovascular responses elicited by linear acceleration in humans. Exp Brain Res. 1999; 125:476–484. PMID: 10323294.


14. Bent LR, Bolton PS, Macefield VG. Modulation of muscle sympathetic bursts by sinusoidal galvanic vestibular stimulation in human subjects. Exp Brain Res. 2006; 174:701–711. PMID: 16721608.


15. Voustianiouk A, Kaufmann H, Diedrich A, Raphan T, Biaggioni I, Macdougall H, Ogorodnikov D, Cohen B. Electrical activation of the human vestibulo-sympathetic reflex. Exp Brain Res. 2006; 171:251–261. PMID: 16308690.


16. Balaban CD, Beryozkin G. Vestibular nucleus projections to nucleus tractus solitarius and the dorsal motor nucleus of the vagus nerve: potential substrates for vestibulo-autonomic interactions. Exp Brain Res. 1994; 98:200–212. PMID: 8050507.


17. Porter JD, Balaban CD. Connections between the vestibular nuclei and brain stem regions that mediate autonomic function in the rat. J Vestib Res. 1997; 7:63–76. PMID: 9057160.


18. Holstein GR, Friedrich VL Jr, Martinelli GP. Projection neurons of the vestibulo-sympathetic reflex pathway. J Comp Neurol. 2014; 522:2053–2074. PMID: 24323841.


19. Barcroft H, Konzett H. On the actions of noradrenaline, adrenaline and isopropyl noradrenaline on the arterial blood pressure, heart rate and muscle blood flow in man. J Physiol. 1949; 110:194–204. PMID: 15406390.


20. Lan Y, Lu HJ, Jiang X, Li LW, Yang YZ, Jin GS, Park JY, Kim MS, Park BR, Jin YZ. Analysis of the baroreceptor and vestibular receptor inputs in the rostral ventrolateral medulla following hypotension in conscious rats. Korean J Physiol Pharmacol. 2015; 19:159–165. PMID: 25729278.


21. Kim MS, Kim JH, Kry D, Choi MA, Choi DO, Cho BG, Jin YZ, Lee SH, Park BR. Effects of acute hypotension on expression of cFos-like protein in the vestibular nuclei of rats. Brain Res. 2003; 962:111–121. PMID: 12543461.


22. Li XL, An Y, Jin QH, Kim MS, Park BR, Jin YZ. Changes of some amino acid concentrations in the medial vestibular nucleus of conscious rats following acute hypotension. Neurosci Lett. 2010; 477:11–14. PMID: 20399837.


23. Li XL, Nian B, Jin Y, Li LW, Jin GS, Kim MS, Park BR, Jin YZ. Mechanism of glutamate receptor for excitation of medial vestibular nucleus induced by acute hypotension. Brain Res. 2012; 1443:27–33. PMID: 22305141.


24. Park BR, Kim MS, Kim JH, Jin YZ. Effects of acute hypotension on neuronal activity in the medial vestibular nuclei of rats. Neuroreport. 2001; 12:3821–3824. PMID: 11726802.


25. Morgan JI, Curran T. Stimulus-transcription coupling in the nervous system: involvement of the inducible proto-oncogenes fos and jun. Annu Rev Neurosci. 1991; 14:421–451. PMID: 1903243.


26. Takayama K, Suzuki T, Miura M. The comparison of effects of various anesthetics on expression of Fos protein in the rat brain. Neurosci Lett. 1994; 176:59–62. PMID: 7970238.


27. Wei S, Lei M, Tong M, Ding J, Han Q, Xiao M. Acute baroreceptor unloading evokes Fos expression in anesthetized rat brain. Brain Res Bull. 2008; 76:63–69. PMID: 18395612.


28. Paxinos G, Watson C. The Rat Brain in Stereotaxic Coordinates. New York, NY, USA: Academic Press;2007.
29. Kim MS, Jin BK, Chun SW, Lee MY, Lee SH, Kim JH, Park BR. Effect of MK801 on cFos-like protein expression in the medial vestibular nucleus at early stage of vestibular compensation in uvulonodullectomized rats. Neurosci Lett. 1997; 231:147–150. PMID: 9300643.


30. Kim MS, Choi DO, Choi MA, Kim JH, Kim KY, Lee MY, Rhee JK, Chun SW, Park BR. Immunohistochemical detection of phosphorylated form of extracellular signal-regulated kinase 1/2 in rat vestibular nuclei following hemorrhagic hypotension. Neurosci Lett. 2004; 360:49–52. PMID: 15082176.


31. Guyenet PG, Haselton JR, Sun MK. Sympathoexcitatory neurons of the rostroventrolateral medulla and the origin of the sympathetic vasomotor tone. Prog Brain Res. 1989; 81:105–116. PMID: 2616776.
32. Granata AR, Denavit-Saubie M. Bulbospinal sympathoexcitatory pathways in the rat. Brain Res Bull. 1994; 34:601–605. PMID: 7922604.


33. Choi MA, Lee JH, Hwang JH, Choi SJ, Kim MS, Park BR. Signaling pathway of glutamate in the vestibular nuclei following acute hypotension in rats. Brain Res. 2008; 1229:111–117. PMID: 18639534.


34. Yates BJ, Grélot L, Kerman IA, Balaban CD, Jakus J, Miller AD. Organization of vestibular inputs to nucleus tractus solitarius and adjacent structures in cat brain stem. Am J Physiol. 1994; 267:R974–R983. PMID: 7524372.


35. Kesse WK, Parker TL, Coupland RE. The innervation of the adrenal gland. I. The source of pre- and postganglionic nerve fibres to the rat adrenal gland. J Anat. 1988; 157:33–41. PMID: 3198482.
36. Minson J, Arnolda L, Llewellyn-Smith I, Pilowsky P, Chalmers J. Altered c-fos in rostral medulla and spinal cord of spontaneously hypertensive rats. Hypertension. 1996; 27:433–441. PMID: 8698450.
37. Minson J, Kapoor V, Llewellyn-Smith I, Pilowsky P, Chalmers J. Kainic acid injection in NTS evokes hypertension and c-fos expression in spinal cord. Neuroreport. 1992; 3:437–440. PMID: 1633282.


38. Li LW, Jin GS, Yang YZ, Ameer AN, Kim MS, Park BR, Jin YZ. Effect of glutamate on the vestibulo-solitary projection after sodium nitroprusside-induced hypotension in conscious rats. Korean J Physiol Pharmacol. 2015; 19:275–281. PMID: 25954134.


39. Kerman IA, McAllen RM, Yates BJ. Patterning of sympathetic nerve activity in response to vestibular stimulation. Brain Res Bull. 2000; 53:11–16. PMID: 11033203.


Fig. 1
Photomicrographs showing the effect of medial vestibular nucleus (MVN) glutamate on c-Fos protein expression in the spinal cord. (A) Effect of microinjection of glutamate receptor agonists into the left MVN on c-Fos protein expression in the intermediolateral cell column (IMC) of the T7 spinal cord. (B) Effect of pretreatment with microinjection of glutamate receptor antagonists into the left MVN on c-Fos protein expression in the IMC of the T7 spinal cord. Right lower quadrant in A and B represents higher magnification. Rectangles in right lower corner of each diagram represent higher magnification. ACSF, microinjection of artificial cerebrospinal fluid into the MVN; NMDA, microinjection of NMDA into the MVN; AMPA, microinjection of AMPA into the MVN; ACSF+SNP, SNP infusion after pretreatment with ACSF in the MVN; MK801+SNP, SNP infusion after pretreatment with MK801 in the MVN; CNQX+SNP, SNP infusion after pretreatment with CNQX in the MVN. ACSF, artificial cerebrospinal fluid; AMPA, 2-amino-3-(5-methyl-3-oxo-1,2-oxazol-4-yl) propanoic acid; CNQX, 6-cyano-7-nitroquinoxaline-2,3-dione; IMC, intermediolateral cell column; MK801, (+)-5-methyl-10,11-dihydro-5H-dibenzo[a,d] cyclohepten-5,10-imine maleate; NMDA, N-methyl-D-aspartic acid; SNP, sodium nitroprusside.

Fig. 2
Bar histogram showing the effect of medial vestibular nucleus (MVN) glutamate on c-Fos protein expression in the spinal cord. ACSF, microinjection of artificial cerebrospinal fluid into the MVN; NMDA, microinjection of NMDA into the MVN; AMPA, microinjection of AMPA into the MVN; ACSF+SNP, SNP infusion after pretreatment with ACSF in the MVN; MK801+SNP, SNP infusion after pretreatment with MK801 in the MVN; CNQX+SNP, SNP infusion after pretreatment with CNQX in the MVN. The number of animals in each group was 7. Values are mean±SE. *Significant difference from ACSF group (**p<0.01). †Significant difference from ACSF + SNP group (‡p<0.01). Other notations are as in Fig. 1.

Fig. 3
Photomicrographs showing the effect of rostral ventrolateral medullary nucleus (RVLM) glutamate on c-Fos protein expression in the spinal cord. (A) Effect of microinjection of glutamate receptor agonists into the right RVLM on c-Fos protein expression in the IMC of the T7 spinal cord. (B) Effect of pretreatment with microinjection of glutamate receptor antagonists into the right RVLM on c-Fos protein expression in the IMC of the T7 spinal cord. Right lower quadrant in A and B represents higher magnification. Rectangles in right lower corner of each diagram represent higher magnification. ACSF, microinjection of artificial cerebrospinal fluid into the RVLM; NMDA, microinjection of NMDA into the RVLM; AMPA, microinjection of AMPA into the RVLM; ACSF+SNP, SNP infusion after pretreatment with ACSF in the RVLM; MK801+SNP, SNP infusion after pretreatment with MK801 in the RVLM; CNQX+SNP, SNP infusion after pretreatment with CNQX in the RVLM. Other notations are as in Fig. 1.

Fig. 4
Bar histogram showing the effect of rostral ventrolateral medullary nucleus (RVLM) glutamate on c-Fos protein expression in the spinal cord. ACSF, microinjection of artificial cerebrospinal fluid into the RVLM; NMDA, microinjection of NMDA into the RVLM; AMPA, microinjection of AMPA into the RVLM; ACSF+SNP, SNP infusion after pretreatment with ACSF in the RVLM; MK801+SNP, SNP infusion after pretreatment with MK801 in the RVLM; CNQX+SNP, SNP infusion after pretreatment with CNQX in the RVLM. The number of animals in each group was 7. Values are mean±SE. *Significant difference from ACSF group (**p<0.01). †Significant difference from ACSF+SNP group (‡p<0.01). Other notations are as in Fig. 1.

Fig. 5
The effect of glutamate on levels of blood epinephrine. (A) Effect of microinjection of glutamate receptor agonists or antagonists into the right medial vestibular nucleus (MVN) on levels of blood epinephrine. (B) Effect of microinjection of glutamate receptor agonists or antagonists into the right rostral ventrolateral medullary nucleus (RVLM) on levels of blood epinephrine. The number of animals in each group was 7. Values are mean±SE. *Significant difference from ACSF group (**p<0.01). †Significant difference from ACSF+SNP group (‡p<0.01). Other notations are as in previous figures.
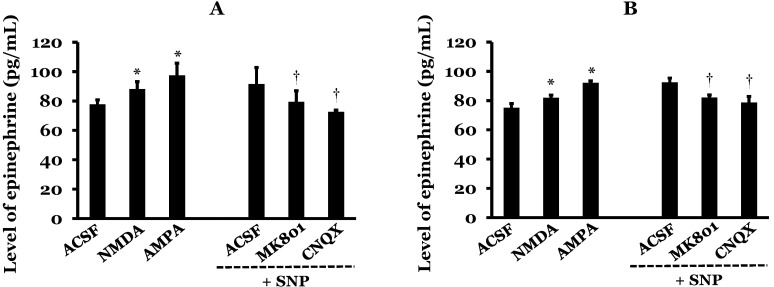
Fig. 6
The effect of microinjection of glutamate receptor agonists into the MVN on blood pressure. Microinjection of AMPA or NMDA into the MVN increased blood pressure, but blood pressure was not changed following ACSF microinjection. AMPA or NMDA was injected into the right MVN at a concentration of 1.5 mmol/L and a volume of 10 µL. Arrows indicate microinjection of ACSF and glutamate agonists.
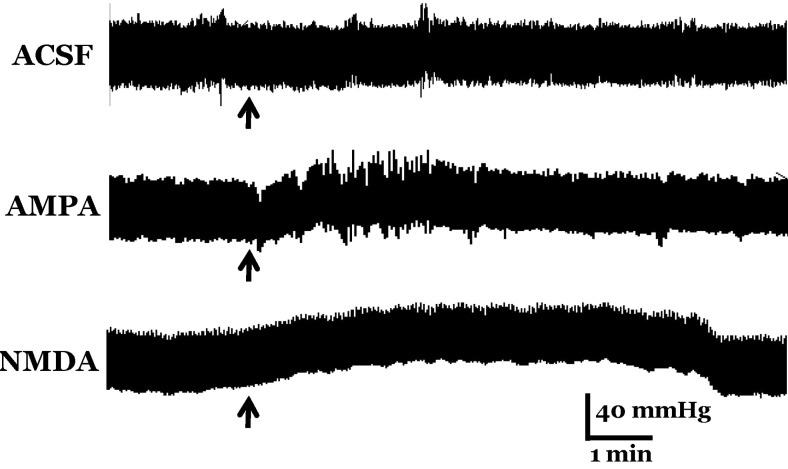
Fig. 7
Schematic diagram of the vestibulo-spino-adrenal (VSA) axis in the vestibulosympathetic reflex. Dotted lines represent pathway of the VSA axis. VNC, vestibular nuclear complex; NTS, nucleus tractus solitarius; CVLM, caudal ventrolateral medulla; RVLM, rostral ventrolateral medulla; IML, intermediolateral cell column of the spinal cord; AM, adrenal medulla.
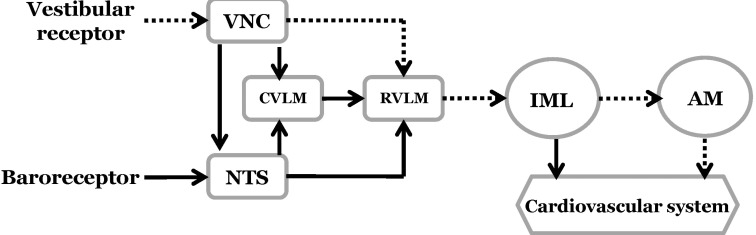