Abstract
Fucoidan, a fucose-rich sulfated polysaccharide derived from brown seaweed in the class Phaeophyceae, has been widely studied for its possible health benefits. However, the potential of fucoidan as a possible treatment for hyperpigmentation is not fully understood. This study investigated the effects of fucoidan on melanogenesis and related signaling pathways using Mel-Ab cells. Fucoidan significantly decreased melanin content. While fucoidan treatment decreased tyrosinase activity, it did not do so directly. Western blot analysis indicated that fucoidan downregulated microphthalmia-associated transcription factor and reduced tyrosinase protein expression. Further investigation showed that fucoidan activated the extracellular signal-regulated kinase (ERK) pathway, suggesting a possible mechanism for the inhibition of melanin synthesis. Treatment with PD98059, a specific ERK inhibitor, resulted in the recovery of melanin production. Taken together, these findings suggest that fucoidan inhibits melanogenesis via ERK phosphorylation.
Fucoidans are a group of sulfated polysaccharides rich in fucose that are found in brown seaweeds of the class Phaeophyceae [1,2]. Fucoidans have a number of potential health benefits to humans such as immunomodulatory, anti-inflammatory, antiviral, and antioxidant effects [2]. Several potential applications of fucoidan have been studied, but its mechanisms of action in different biological activities are not completely clear. In addition, there are no officially approved dermatological applications of fucoidan [2]. We recently reported that fucoidan promotes the proliferation of human fibroblasts [3]. Furthermore, fucoidan increases expression of proliferating cell nuclear antigen (PCNA) and p63 in the epidermis of a reconstructed skin equivalent [3]. Therefore, it is feasible that fucoidan may have an influence on epidermal melanocytes. Thus, we investigated the effects of fucoidan on melanin synthesis and the related regulatory mechanisms.
Melanin is a pigmented biopolymer synthesized by melanocytes through the process of melanogenesis [4,5], which is responsible for human skin coloration. Overproduction of melanin leads to hyperpigmentary problems that affect quality of life [6,7]. Melanin overproduction continues to be a concern because safe and effective treatments are limited.
Microphthalmia-associated transcription factor (MITF) is a key regulator of the development, proliferation, and survival of melanocytes as well as the expression of enzymes and structural proteins involved in melanin synthesis [8]. MITF upregulation activates the expression of downstream melanogenic proteins including tyrosinase, tyrosinase-related protein 1 (TRP-1) and TRP-2 [4,8]. Tyrosinase is a major regulator of melanogenesis; it is, in fact, the rate-limiting enzyme of melanin synthesis [9,10]. Thus, melanin synthesis is primarily regulated by the expression and activity of tyrosinase, and agents that inhibit the activity or expression of tyrosinase or MITF may be useful in treating hyperpigmentation disorders and reduce the melanin content of skin.
The cAMP pathway is a major regulatory mechanism of MITF expression [11]. Phosphorylation of the cAMP response-element binding protein (CREB) increases MITF expression, resulting in melanin production [12,13]. In addition, several other signaling pathways have been implicated in the control of melanogenesis. The extracellular signal-regulated kinase (ERK) pathway is a key signaling pathway deeply involved in controlling cell growth. Indeed, it is generally accepted that ERK activation increases cell proliferation in a variety of cell types [14]. In addition, it has been reported that ERK is related to the regulation of cell differentiation depending on cell type [15,16]. For example, the ERK pathway is reported to be involved in MITF regulation in melanocytes [17,18]. Thus, ERK activation phosphorylates MITF, leading to its degradation and resulting in a decrease in melanin synthesis by melanocytes [19,20].
The Akt pathway is another key melanogenic regulatory mechanism. Specifically, Akt phosphorylates MITF, inhibiting its activation and resulting in inhibition of melanogenesis [21,22]. As mentioned above, fucoidan increases the proliferation of human fibroblasts [3]. In addition, fucoidan also stimulates ERK and Akt phosphorylation in human umbilical vein endothelial cells [23]. In the present study, we hypothesized that fucoidan may regulate the ERK and/or Akt pathways. In an extension of this possibility, we investigated whether fucoidan influences ERK and Akt signaling in the context of melanogenesis.
Fucoidan (from Fucus vesiculosus), 3,4-dihydroxyl-L-phenylalanine (L-DOPA), cholera toxin (CT), 12-O-tetradecanoylphorbol-13-acetate (TPA), and mushroom tyrosinase were from Sigma-Aldrich Co. (St. Louis, MO, USA). Antibodies specific for phospho-ERK1/2 (Thr202/Tyr204, #9101S), total (phosphorylated and non-phosphorylated) ERK1/2 (#9102), phospho-specific Akt (Ser473, #9271), total Akt (#9916), phospho-CREB (ser133, #9198), and total-CREB (#9197) were purchased from Cell Signaling Technology (Beverly, MA, USA). Antibodies for tyrosinase (C-19) and actin (I-19) were purchased from Santa Cruz Biotechnology, Inc. (Santa Cruz, CA, USA). Microphthalmia Ab-1 (C5, MS-771-P0) was from NeoMarkers (Fremont, CA, USA). PD98059 was from Cell Signaling Technology. Secondary antibodies specific for anti-goat IgG (PI-9500), anti-mouse IgG (PI-2000), and anti-rabbit IgG (PI-1000) were purchased from Vector Laboratories (Burlingame, CA, USA).
Mel-Ab is a spontaneously immortalized melanocyte cell line that synthesizes large quantities of melanin [24]. Mel-Ab cells were maintained in Dulbecco's modified Eagle's medium (DMEM) supplemented with 10% fetal bovine serum (FBS), 100 nM TPA, 1 nM CT, 50 µg/mL streptomycin, and 50 µg/mL penicillin (Hyclone Logan, UT, USA) at 37℃ in 5% CO2.
Cell viability was measured using a crystal violet assay [25]. After incubation with fucoidan for 24 h, culture medium was removed and cells were stained with 0.1% crystal violet in 10% ethanol for 5 min at room temperature. Cells were rinsed four times with distilled water and retained crystal violet was extracted with 95% ethanol. Absorbance was measured at 590 nm using an ELISA reader (VERSAMax; Molecular Devices, Sunnyvale, CA, USA).
Melanin content was measured as described previously with slight modifications [20]. Briefly, Mel-Ab cells were treated with fucoidan in DMEM containing 10% FBS. Cells were then dissolved in 550 µL 1 N NaOH at 100℃ for 30 min and centrifuged at 13,000 rpm for 5 min. Optical densities of supernatants containing the same amount of protein were measured at 400 nm using an ELISA reader. Before measuring melanin content, cells were observed under a phase contrast microscope (Olympus IX50, Tokyo, Japan) and photographed using the attached DCM300 digital camera (Scopetek, Inc., Hangzhou, China), supported by ScopePhoto software (Scopetek, Inc.).
Tyrosinase activity was measured using the DOPA oxidase activity assay [26]. Mel-Ab cells were seeded in 6-well plates and incubated with fucoidan for 72 h. Cells were then washed with ice-cold PBS and lysed with phosphate buffer (pH 6.8) containing 1% Triton X-100. Cells were disrupted by freezing and thawing, and the resulting lysates were clarified by centrifugation at 15,000 rpm for 10 min. After quantifying lysate protein levels and adjusting protein concentrations with lysis buffer, 90 µL of lysate containing equal amounts of protein were plated on 96-well plates, to which 10 µL of 10 mM L-DOPA was added. Control wells contained 90 µL of lysis buffer and 10 µL of 10 mM L-DOPA. After incubation at 37℃, dopachrome formation was monitored by measuring the absorbance at 475 nm every 10 min for at least 1 h using an ELISA reader.
A cell-free assay system was used to examine the direct effects of fucoidan on tyrosinase activity. For this assay, 70 µL phosphate buffer containing fucoidan was mixed with 20 µL of 53.7 units/mL mushroom tyrosinase, to which 10 µL of 10 mM L-DOPA was added. Following incubation at 37℃, absorbance was measured at 475 nm.
Cells were lysed in cell lysis buffer containing 62.5 mM/L Tris-HCl, pH 6.8, 2% sodium dodecyl sulfate (SDS), 5% β-mercaptoethanol, 2 mM phenylmethylsulfonyl fluoride, protease inhibitors (Complete™; Roche, Mannheim, Germany), 1 mM Na3VO4, 50 nM NAF, and 10 mM EDTA. Proteins were separated by SDS-polyacrylamide gel electrophoresis and blotted onto polyvinylidene fluoride (PVDF) membranes. Membranes were blocked with 5% skim milk in Tris-buffered saline containing 0.5% Tween 20. Blots were then incubated with appropriate primary antibodies diluted 1:1000, washed, and incubated with horseradish peroxidase-conjugated secondary antibodies. Bound antibodies were detected using enhanced chemiluminescence plus kits (Amersham International, Little Chalfont, UK). Images of the blotted membranes were obtained using an LAS-4000 lumino-image analyzer (Fuji Film, Tokyo, Japan).
Mel-Ab cells were treated with 10~200 µg/mL fucoidan for 24 h. Fucoidan did not exhibit any cytotoxic effects at these concentrations (Fig. 1).
To determine the effects of fucoidan on melanin synthesis in Mel-Ab cells, cellular melanin and tyrosinase activity were measured in Mel-Ab cells with or without fucoidan. Cells were treated with 0~200 µg/mL fucoidan for 72 h and photographed with a phase-contrast microscope. The level of pigmentation was lower in fucoidan-treated cells than the control (Fig. 2A), and fucoidan treatment significantly decreased melanin content (Fig. 2B). Because tyrosinase is the primary regulator of melanin synthesis [27], the effects of fucoidan on cellular tyrosinase activity were examined by evaluating L-DOPA oxidation activity. Consistent with the reduction in melanin content, tyrosinase activity was decreased in fucoidan-treated cells (Fig. 2C). To investigate if fucoidan directly inhibited tyrosinase, we measured the effects of fucoidan on mushroom tyrosinase in a cell-free system. Fucoidan did not directly inhibit mushroom tyrosinase activity (Fig. 2D). Taken together, these results suggested that fucoidan-induced inhibition of melanin synthesis occurred via downregulation of tyrosinase expression.
Changes in protein levels of MITF and tyrosinase were investigated in a time-course experiment. Mel-Ab cells were treated with 50 µg/mL fucoidan for 24, 48, or 72 h, and Western blotting was performed to analyze fucoidantreated cells. Treatment with fucoidan decreased levels of MITF and tyrosinase up to 72 h (Fig. 3). These results suggested that fucoidan inhibited melanin synthesis by downregulating MITF and tyrosinase protein expression.
ERK and Akt activation have been reported to be involved in inhibiting melanogenesis [12,19,28]. Thus, we next investigated if the hypopigmentary effects of fucoidan were related to activation of either ERK or Akt. Fucoidan treatment (50 µg/mL) induced phosphorylation of ERK, but not Akt (Fig. 4). Involvement of the PKA pathway was also examined. No significant changes in CREB levels were observed (Fig. 4).
To determine whether fucoidan-mediated ERK phosphorylation was responsible for the reduction in melanin synthesis, Mel-Ab cells were treated with PD98059, a specific ERK pathway inhibitor. Cells were incubated with 50 µg/mL fucoidan for 48 h after pretreatment with 10 µM PD98059 for 1 h. Cells were photographed using phase-contrast microscopy (Fig. 5A). Fucoidan-treated cells showed decreased pigmentation whereas cells treated with PD98059 showed evidence of melanin synthesis recovery. Melanin content was also measured after pretreatment with PD98059. We found that PD98059 rescued fucoidan-inhibited melanin synthesis (Fig. 5B). We also investigated if fucoidan-induced phosphorylation of ERK was abolished by PD98059. Western blot data was consistent with melanin content results (Fig. 6A). Specifically, addition of PD98059 blocked downregulation of MITF and tyrosinase by fucoidan, and that MITF and tyrosinase protein levels were restored by PD98059 (Fig. 6B). Taken together, these results indicated that reduction of melanin synthesis by fucoidan was caused by ERK phosphorylation.
Seaweed is widely used as a folk medicine treatment for diseases such as eczema, scabies, psoriasis, heart disease, atherosclerosis and renal problems [29]. Brown seaweed has been studied as a possible treatment for hyperpigmentation. For example, in an investigation of the antimelanogenic effect of Sargassum polycystum, it was reported to inhibit cellular tyrosinase activity in B16F10 cells [30]. Wang et al. also suggested that fucoidan from Fucus vesiculosus may be a potential melanin-reducing agent based on their study of fucoidan inhibition of tyrosinase using a combination of inhibition kinetics and computational simulations [31]. However, the mechanism of action of fucoidan has not yet been fully elucidated. Therefore, we investigated the underlying antimelanogenic molecular mechanism of fucoidan in Mel-Ab cells.
Tyrosinase is the key enzyme in melanin biosynthesis [10], and thus regulating tyrosinase activity is a way to control melanogenesis. For this reason, direct inhibitors of tyrosinase activity have been sought as a way to treat hyperpigmentation [9]. We first tested the effects of fucoidan on tyrosinase activity, and found that fucoidan decreased tyrosinase activity in Mel-Ab cells, but did not directly alter mushroom tyrosinase activity in a cell-free system. These results indicated that the fucoidan-mediated inhibition of melanogenesis might not be due to direct inhibition of tyrosinase, but rather inhibition of tyrosinase expression.
MITF is a central regulating factor of tyrosinase expression [32,33], and we found that fucoidan decreased MITF and tyrosinase protein levels. These results suggested that the melanogenic signaling pathways regulating MITF expression and tyrosinase were involved in fucoidan-induced reduction of melanin synthesis.
CREB is a key factor in melanogenesis [34]. Phosphorylation of CREB promotes melanin synthesis through increased expression of MITF and tyrosinase [34]. Therefore, we examined the effect of fucoidan on CREB phosphorylation. No inactivation of phosphorylation was observed, suggesting that CREB was not involved in fucoidan-induced melanin reduction.
To determine the specific signaling mechanism involved in the fucoidan-regulated reduction of melanin synthesis, we investigated the effect of fucoidan on other melanogenic pathways. The ERK and Akt pathways have both been reported to be involved in the regulation of melanogenesis [19,21]. Akt is broadly implicated in cell survival and mitogenic signaling [35,36]. In addition, activation of the Akt pathway also reduces transcription of tyrosinase [37]. Therefore, we studied the effect of fucoidan on the Akt pathway, but observed no significant effects. On the other hand, fucoidan has been reported to stimulate ERK phosphorylation and cell proliferation in human umbilical vein endothelial cells [23], and thus we considered the possibility that fucoidan may activate ERK in Mel-Ab cells. Although ERK activation was mainly related to stimulation of cell proliferation, it also regulates cell differentiation [14]. Activation of the ERK pathway is known to phosphorylate MITF, leading to its subsequent degradation and hypopigmentation in melanocytes [38].
We previously reported several agents that inhibit melanin synthesis via activating ERK [12,18]. On the other hand, there is a discrepancy about the relationship between ERK activation and melanin inhibition. It was reported that ERK inhibition is accompanied by a reduction of melanin synthesis in B16F10 cells [39]. However, in the present study we found that fucoidan-induced activation of the ERK pathway led to a reduction in melanin synthesis in Mel-Ab cells. To verify these results, Mel-Ab cells were treated with PD98059, an ERK inhibitor. Treatment with PD98059 abrogated fucoidan-induced melanin reduction and prevented downregulation of MITF and tyrosinase. In normal human melanocytes, sphingosylphosphorylcholine also downregulates MITF and tyrosinase via ERK activation [19]. Moreover, the inhibition of the ERK pathway by PD98059 promotes MITF and tyrosinase expression [19,20]. These reports indicate that ERK activation is deeply involved in the inhibition of melanin synthesis. Therefore, our results suggest that fucoidan downregulates MITF and tyrosinase and subsequently reduces melanin synthesis via ERK activation.
In conclusion, this study demonstrated that ERK activation is involved in the anti-melanogenic mechanism of fucoidan. The ERK pathway led to MITF and tyrosinase downregulation, resulting in a reduction in melanin synthesis.
ACKNOWLEDGEMENTS
This research was supported by the Department of Convergence Medicine and Pharmaceutical Biosciences Research Scholarship Grants, Chung-Ang University in 2013.
References
1. Ale MT, Maruyama H, Tamauchi H, Mikkelsen JD, Meyer AS. Fucoidan from Sargassum sp. and Fucus vesiculosus reduces cell viability of lung carcinoma and melanoma cells in vitro and activates natural killer cells in mice in vivo. Int J Biol Macromol. 2011; 49:331–336. PMID: 21624396.


2. Ale MT, Mikkelsen JD, Meyer AS. Important determinants for fucoidan bioactivity: a critical review of structure-function relations and extraction methods for fucose-containing sulfated polysaccharides from brown seaweeds. Mar Drugs. 2011; 9:2106–2130. PMID: 22073012.


3. Song YS, Li H, Balcos MC, Yun HY, Baek KJ, Kwon NS, Choi HR, Park KC, Kim DS. Fucoidan promotes the reconstruction of skin equivalents. Korean J Physiol Pharmacol. 2014; 18:327–331. PMID: 25177165.


4. Kondo T, Hearing VJ. Update on the regulation of mammalian melanocyte function and skin pigmentation. Expert Rev Dermatol. 2011; 6:97–108. PMID: 21572549.


5. Schiaffino MV. Signaling pathways in melanosome biogenesis and pathology. Int J Biochem Cell Biol. 2010; 42:1094–1104. PMID: 20381640.


6. Speeckaert R, Van Gele M, Speeckaert MM, Lambert J, van Geel N. The biology of hyperpigmentation syndromes. Pigment Cell Melanoma Res. 2014; 27:512–524. PMID: 24612852.


7. Gilchrest BA, Park HY, Eller MS, Yaar M. Mechanisms of ultraviolet light-induced pigmentation. Photochem Photobiol. 1996; 63:1–10. PMID: 8577860.


8. Vachtenheim J, Borovanský J. "Transcription physiology" of pigment formation in melanocytes: central role of MITF. Exp Dermatol. 2010; 19:617–627. PMID: 20201954.


9. Ando H, Kondoh H, Ichihashi M, Hearing VJ. Approaches to identify inhibitors of melanin biosynthesis via the quality control of tyrosinase. J Invest Dermatol. 2007; 127:751–761. PMID: 17218941.


10. Hearing VJ, Tsukamoto K. Enzymatic control of pigmentation in mammals. FASEB J. 1991; 5:2902–2909. PMID: 1752358.


11. Bertolotto C, Bille K, Ortonne JP, Ballotti R. In B16 melanoma cells, the inhibition of melanogenesis by TPA results from PKC activation and diminution of microphthalmia binding to the M-box of the tyrosinase promoter. Oncogene. 1998; 16:1665–1670. PMID: 9582014.


12. Kim EH, Kim MK, Yun HY, Baek KJ, Kwon NS, Park KC, Kim DS. Menadione (Vitamin K3) decreases melanin synthesis through ERK activation in Mel-Ab cells. Eur J Pharmacol. 2013; 718:299–304. PMID: 24012927.


13. Ahn MJ, Hur SJ, Kim EH, Lee SH, Shin JS, Kim MK, Uchizono JA, Whang WK, Kim DS. Scopoletin from Cirsium setidens Increases Melanin Synthesis via CREB Phosphorylation in B16F10 Cells. Korean J Physiol Pharmacol. 2014; 18:307–311. PMID: 25177162.
14. Marshall CJ. Specificity of receptor tyrosine kinase signaling: transient versus sustained extracellular signal-regulated kinase activation. Cell. 1995; 80:179–185. PMID: 7834738.


15. Cowley S, Paterson H, Kemp P, Marshall CJ. Activation of MAP kinase kinase is necessary and sufficient for PC12 differentiation and for transformation of NIH 3T3 cells. Cell. 1994; 77:841–852. PMID: 7911739.


16. Sale EM, Atkinson PG, Sale GJ. Requirement of MAP kinase for differentiation of fibroblasts to adipocytes, for insulin activation of p90 S6 kinase and for insulin or serum stimulation of DNA synthesis. EMBO J. 1995; 14:674–684. PMID: 7882971.


17. Alesiani D, Cicconi R, Mattei M, Bei R, Canini A. Inhibition of Mek 1/2 kinase activity and stimulation of melanogenesis by 5,7-dimethoxycoumarin treatment of melanoma cells. Int J Oncol. 2009; 34:1727–1735. PMID: 19424591.


18. Li H, Kim J, Hahn HG, Yun J, Jeong HS, Yun HY, Baek KJ, Kwon NS, Min YS, Park KC, Kim DS. KHG26792 Inhibits Melanin Synthesis in Mel-Ab Cells and a Skin Equivalent Model. Korean J Physiol Pharmacol. 2014; 18:249–254. PMID: 24976765.


19. Kim DS, Park SH, Kwon SB, Park ES, Huh CH, Youn SW, Park KC. Sphingosylphosphorylcholine-induced ERK activation inhibits melanin synthesis in human melanocytes. Pigment Cell Res. 2006; 19:146–153. PMID: 16524430.


20. Kim DS, Hwang ES, Lee JE, Kim SY, Kwon SB, Park KC. Sphingosine-1-phosphate decreases melanin synthesis via sustained ERK activation and subsequent MITF degradation. J Cell Sci. 2003; 116:1699–1706. PMID: 12665551.


21. Oka M, Nagai H, Ando H, Fukunaga M, Matsumura M, Araki K, Ogawa W, Miki T, Sakaue M, Tsukamoto K, Konishi H, Kikkawa U, Ichihashi M. Regulation of melanogenesis through phosphatidylinositol 3-kinase-Akt pathway in human G361 melanoma cells. J Invest Dermatol. 2000; 115:699–703. PMID: 10998146.


22. Khaled M, Larribere L, Bille K, Ortonne JP, Ballotti R, Bertolotto C. Microphthalmia associated transcription factor is a target of the phosphatidylinositol-3-kinase pathway. J Invest Dermatol. 2003; 121:831–836. PMID: 14632202.


23. Kim BS, Park JY, Kang HJ, Kim HJ, Lee J. Fucoidan/FGF-2 induces angiogenesis through JNK- and p38-mediated activation of AKT/MMP-2 signalling. Biochem Biophys Res Commun. 2014; 450:1333–1338. PMID: 25003321.


24. Dooley TP, Gadwood RC, Kilgore K, Thomasco LM. Development of an in vitro primary screen for skin depigmentation and antimelanoma agents. Skin Pharmacol. 1994; 7:188–200. PMID: 8024800.


25. Ishiyama M, Tominaga H, Shiga M, Sasamoto K, Ohkura Y, Ueno K. A combined assay of cell viability and in vitro cytotoxicity with a highly water-soluble tetrazolium salt, neutral red and crystal violet. Biol Pharm Bull. 1996; 19:1518–1520. PMID: 8951178.


26. Buscà R, Bertolotto C, Ortonne JP, Ballotti R. Inhibition of the phosphatidylinositol 3-kinase/p70(S6)-kinase pathway induces B16 melanoma cell differentiation. J Biol Chem. 1996; 271:31824–31830. PMID: 8943224.


27. Wang N, Hebert DN. Tyrosinase maturation through the mammalian secretory pathway: bringing color to life. Pigment Cell Res. 2006; 19:3–18. PMID: 16420243.


28. Kim EH, Jeong HS, Yun HY, Baek KJ, Kwon NS, Park KC, Kim DS. Geranylgeranylacetone inhibits melanin synthesis via ERK activation in Mel-Ab cells. Life Sci. 2013; 93:226–232. PMID: 23792203.


29. Raghavendran HB, Sathivel A, Devaki T. Defensive nature of Sargassum polycystum (Brown alga) against acetaminopheninduced toxic hepatitis in rats: role of drug metabolizing microsomal enzyme system, tumor necrosis factor-alpha and fate of liver cell structural integrity. World J Gastroenterol. 2006; 12:3829–3834. PMID: 16804966.
30. Chan YY, Kim KH, Cheah SH. Inhibitory effects of Sargassum polycystum on tyrosinase activity and melanin formation in B16F10 murine melanoma cells. J Ethnopharmacol. 2011; 137:1183–1188. PMID: 21810462.


31. Wang ZJ, Si YX, Oh S, Yang JM, Yin SJ, Park YD, Lee J, Qian GY. The effect of fucoidan on tyrosinase: computational molecular dynamics integrating inhibition kinetics. J Biomol Struct Dyn. 2012; 30:460–473. PMID: 22694253.


32. Bertolotto C, Abbe P, Hemesath TJ, Bille K, Fisher DE, Ortonne JP, Ballotti R. Microphthalmia gene product as a signal transducer in cAMP-induced differentiation of melanocytes. J Cell Biol. 1998; 142:827–835. PMID: 9700169.


33. Lee HE, Kim EH, Choi HR, Sohn UD, Yun HY, Baek KJ, Kwon NS, Park KC, Kim DS. Dipeptides Inhibit Melanin Synthesis in Mel-Ab Cells through Down-Regulation of Tyrosinase. Korean J Physiol Pharmacol. 2012; 16:287–291. PMID: 22915995.


34. Buscà R, Ballotti R. Cyclic AMP a key messenger in the regulation of skin pigmentation. Pigment Cell Res. 2000; 13:60–69. PMID: 10841026.
35. Dent P. Crosstalk between ERK, AKT, and cell survival. Cancer Biol Ther. 2014; 15:245–246. PMID: 24424114.


36. Ocana A, Vera-Badillo F, Al-Mubarak M, Templeton AJ, Corrales-Sanchez V, Diez-Gonzalez L, Cuenca-Lopez MD, Seruga B, Pandiella A, Amir E. Activation of the PI3K/mTOR/AKT pathway and survival in solid tumors: systematic review and meta-analysis. PLoS One. 2014; 9:e95219. PMID: 24777052.


37. Khaled M, Larribere L, Bille K, Aberdam E, Ortonne JP, Ballotti R, Bertolotto C. Glycogen synthase kinase 3beta is activated by cAMP and plays an active role in the regulation of melanogenesis. J Biol Chem. 2002; 277:33690–33697. PMID: 12093801.
38. Wu M, Hemesath TJ, Takemoto CM, Horstmann MA, Wells AG, Price ER, Fisher DZ, Fisher DE. c-Kit triggers dual phosphorylations, which couple activation and degradation of the essential melanocyte factor Mi. Genes Dev. 2000; 14:301–312. PMID: 10673502.


39. Yoon HS, Lee SR, Ko HC, Choi SY, Park JG, Kim JK, Kim SJ. Involvement of extracellular signal-regulated kinase in nobiletin-induced melanogenesis in murine B16/F10 melanoma cells. Biosci Biotechnol Biochem. 2007; 71:1781–1784. PMID: 17617702.


Fig. 1
Fucoidan has no influence on cell viability. Mel-Ab cells were treated with 0~200 µg/mL fucoidan for 24 h in serum-free media. Cell viability was measured by crystal violet assay. Determinations were made in triplicate. Data shown are the mean±SD.
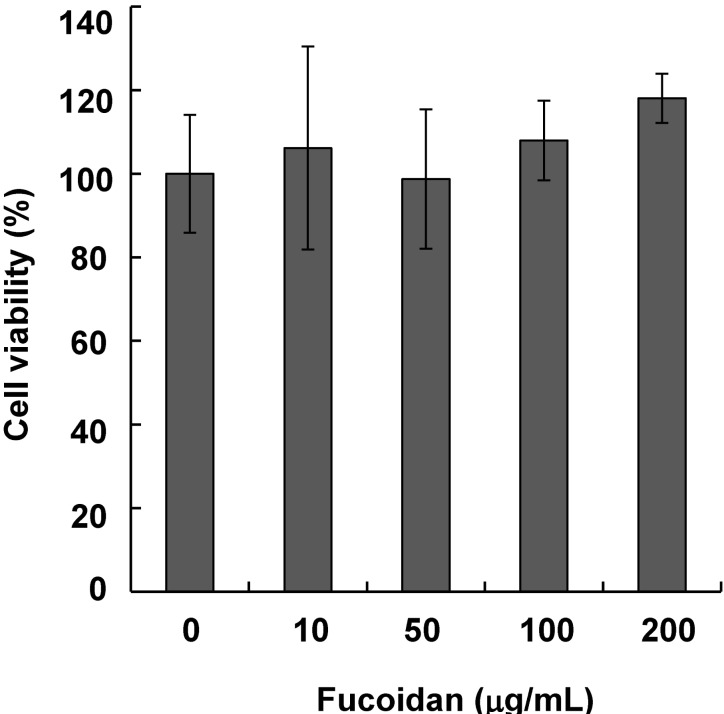
Fig. 2
Inhibitory effects of fucoidan on melanogenesis. (A) Mel-Ab cells were treated with 0~200 µg/mL fucoidan for 72 h and examined by phase contrast microscopy. (B) Melanin content and (C) tyrosinase activity were assessed as described in the Materials and Methods section. (D) To test the direct effect of fucoidan on tyrosinase, activity in a cell-free system was measured. Each measurement was performed in triplicate. Data shown are the mean±SD. **p<0.01 compared to the untreated control.
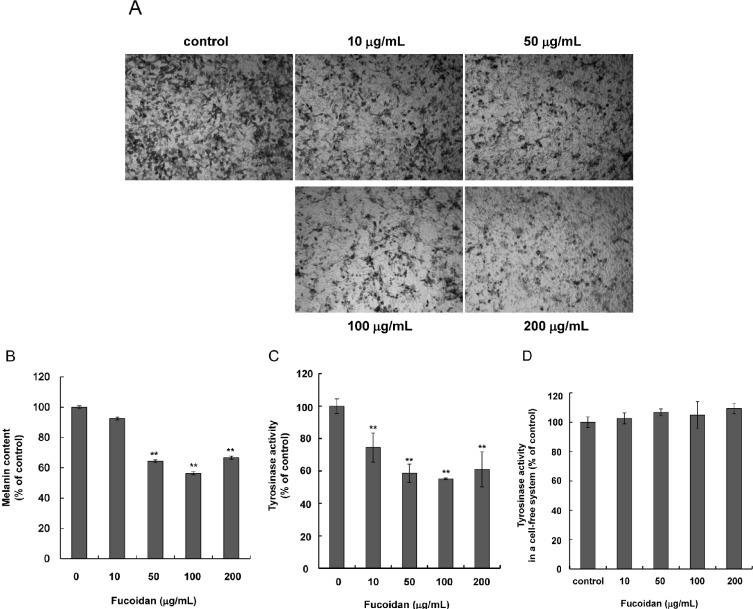
Fig. 3
Effects of fucoidan treatment on MITF and tyrosinase protein expression. Mel-Ab cells were cultured with 50 µg/mL fucoidan for 24, 48, or 72 h. Whole cell lysates were subjected to Western blotting using antibodies against MITF and tyrosinase. An actin antibody was used as a loading control.
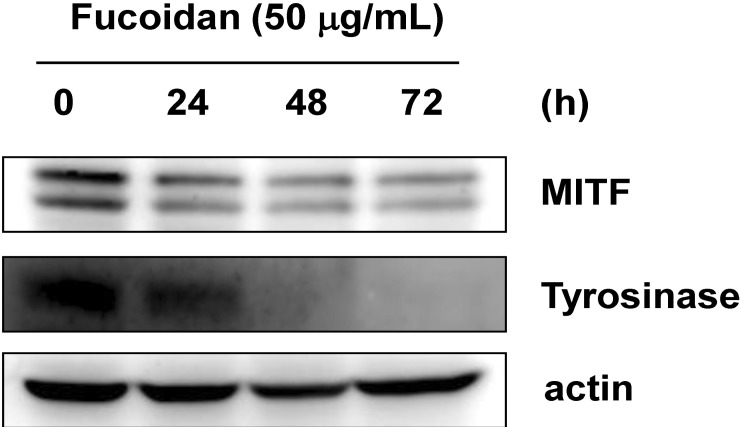
Fig. 4
Effects of fucoidan treatment on signal transduction pathways. After serum starvation for 24 h, Mel-Ab cells were treated 50 µg/mL fucoidan for the indicated periods. Whole cell lysates were subjected to Western blots using phospho-specific antibodies for ERK, Akt, and CREB. Phosphorylation-independent ERK, Akt and CREB antibodies were used as loading controls, respectively.
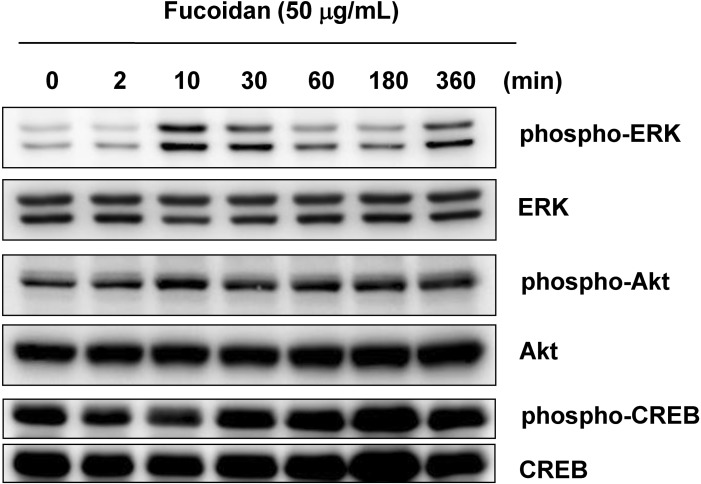
Fig. 5
Effects of fucoidan on melanin synthesis in the presence of PD98059. Mel-Ab cells were treated with 10 µM PD98059 for 1 h and incubated with 50 µg/mL fucoidan for 3 days. (A) Phase contrast microscopy. (B) Melanin content measurements performed in triplicate. Data are the mean±SD. **p<0.01 compared to the fucoidan-treated control.
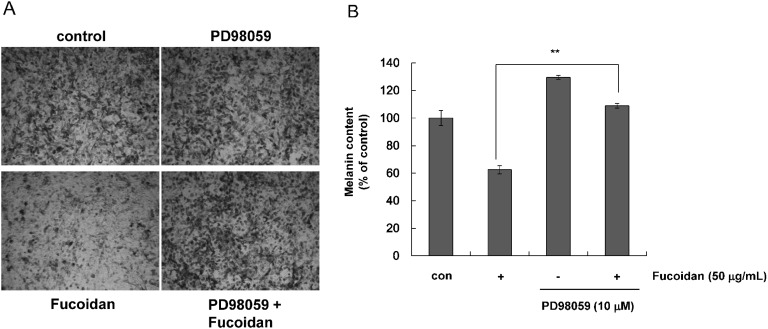
Fig. 6
Effects of fucoidan on ERK phosphorylation and expression of MITF and tyrosinase in the presence of PD98059. Mel-Ab cells were treated with 10 µM PD98059 for 1 h, then incubated with 50 µg/mL of fucoidan for 30 min (A) or 48 h (B). Whole cell extracts were subjected to Western blotting with antibodies against phospho-ERK (A), or MITF and tyrosinase (B). Actin and phosphorylation-independent ERK antibodies were used as loading controls.
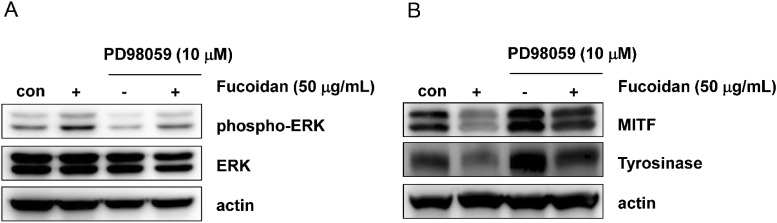