Abstract
Orthostatic hypotension is most common in elderly people, and its prevalence increases with age. Attenuation of the vestibulo-sympathetic reflex (VSR) is commonly associated with orthostatic hypotension. In this study, we investigated the role of glutamate on the vestibulo-solitary projection of the VSR pathway to clarify the pathophysiology of orthostatic hypotension. Blood pressure and expression of both pERK and c-Fos protein were evaluated in the nucleus tractus solitarius (NTS) after microinjection of glutamate into the medial vestibular nucleus (MVN) in conscious rats with sodium nitroprusside (SNP)-induced hypotension that received baroreceptor unloading via sinoaortic denervation (SAD). SNP-induced hypotension increased the expression of both pERK and c-Fos protein in the NTS, which was abolished by pretreatment with glutamate receptor antagonists (MK801 or CNQX) in the MVN. Microinjection of glutamate receptor agonists (NMDA or AMPA) into the MVN increased the expression of both pERK and c-Fos protein in the NTS without causing changes in blood pressure. These results indicate that both NMDA and AMPA receptors play a significant role in the vestibulo-solitary projection of the VSR pathway for maintaining blood pressure, and that glutamatergic transmission in this projection might play a key role in the pathophysiology of orthostatic hypotension.
Orthostatic hypotension is defined as a reduction in systolic blood pressure of at least 20 mmHg or a reduction in diastolic blood pressure of at least 10 mmHg during the first 3 min of standing or a head-up tilt on a tilt table [1]. Characteristic symptoms of orthostatic hypotension include headache, dizziness, presyncope, and syncope in response to sudden postural change. It is common in 50% of elderly people living in care facilities and its prevalence increases with age [2]. Standing from a lying or sitting position results in the pooling of 500 to 1000 ml of blood in the lower extremities, which causes a decrease in venous return to the heart, and results in decreased cardiac output and blood pressure. These hemodynamic changes provoke the baroreceptor reflex and postural changes provoke the vestibulo-sympathetic reflex (VSR). These reflexes increase peripheral resistance, venous return to the heart, and cardiac output, thereby limiting the drop in blood pressure. However, reduced baroreceptor reflex responsiveness and attenuation of the VSR are commonly associated with orthostatic hypotension. Impairment of baroreceptor reflex associated with orthostatic hypotension has been well established, but the role of the vestibular system in the mechanisms of orthostatic hypotension is not well understood.
The vestibular system controls autonomic function for the maintenance of respiration and blood pressure, and controls body posture through the contraction of extraocular and skeletal muscles in response to head movement [3]. However, animals with loss of vestibular function experience orthostatic hypotension and impairment of reflex control of body posture during head-up tilt [4]. Thus, peripheral vestibular receptors are necessary to produce the VSR for controlling blood pressure responses to postural changes.
The baroreceptor reflex is a feedback control system for maintaining arterial blood pressure. Baroreceptors in the carotid sinus and aortic arch are sensitive to changes in the distension of the vascular wall. Signals from baroreceptors are conveyed by branches of the glossopharyngeal and vagus nerves to the nucleus tractus solitarius (NTS), and then to the caudal ventrolateral medulla (CVLM), which is composed of inhibitory neurons. Neurons from the CVLM project into the rostral ventrolateral medulla (RVLM), which contains sympathetic preganglionic neurons [5]. In the VSR, neurons from the medial and inferior vestibular nuclei project to the NTS and RVLM, which control autonomic responses including those related to cardiovascular functions during head movement [6,7]. Therefore, the NTS and RVLM are integration centers of the baroreceptor reflex and VSR for maintaining blood pressure. Previous studies in our laboratory have found that the removal of baroreceptors or vestibular receptors decreases the expression of c-Fos protein in the NTS and RVLM following acute hypotension, which supports the role of these receptors in the regulation of blood pressure [8,9]. The RVLM contains sympathetic preganglionic neurons and receives afferent input from various regions including the CVLM and vestibular system, but the NTS receives afferent inputs mainly from baroreceptors and vestibular receptors. Neurochemical study of the neural connections from the vestibular system to the NTS (vestibulo-solitary projection) is critical for understanding the pathophysiology of orthostatic hypotension because the NTS is the primary integration center for baroreceptor and vestibular receptor inputs.
The baroreceptor reflex pathway includes a glutamatergic connection between the NTS and the CVLM, which was reported in a phenylephrine-induced hypertensive animal model [10]. However, the role of glutamate release from vestibular afferents in the NTS in the VSR pathway for the maintenance of blood pressure is not understood. Previous studies in our laboratory reported that a reduction in blood pressure elicits excitation of peripheral vestibular receptors [9,11,12,13]. Hypotension induced by sodium nitroprusside (SNP) infusion or hemorrhage elicits excitatory signals in peripheral vestibular receptors, and these signals are conveyed to the vestibular nuclei, which then result in increased neural activity and c-Fos/pERK expression in the vestibular nuclei. Furthermore, glutamate release increases in the vestibular nuclei following hypotension [14,15]. Excitatory signals induced by hypotension in peripheral vestibular receptors are transmitted to the NTS and RVLM through the vestibular nuclei [8,9].
The immediate early gene c-fos is transiently expressed after a variety of stimuli and is considered a useful marker of neuronal activity in the central nervous system [16]. c-Fos protein, encoded by the proto-oncogene c-fos, is expressed within 30 min after stimulation and is expressed exclusively in the nucleus. On the other hand, extracellular signal-regulated kinase (ERK) is activated by phosphorylation, and pERK expression is much more rapid and dynamic. pERK is expressed in various cellular and subcellular compartments including the nucleus, cytoplasm, axons, and dendrites [17]. Several studies have used c-Fos or pERK expression to identify the brain regions that respond to changes in blood pressure [10,18]. Therefore, measurement of c-Fos and pERK in the NTS following hypotension would be very useful for understanding the effect of glutamate on the vestibulo-solitary projection of the VSR pathway.
In this study, we investigated the role of glutamatergic transmission in the vestibulo-solitary projection of the VSR pathway to clarify the pathophysiology of orthostatic hypotension. To this end, changes in blood pressure and expression of both pERK and c-Fos protein in the NTS, caused by the microinjection of glutamate into the medial vestibular nucleus (MVN), were investigated using immunohistochemical methods in conscious SNP-induced hypotensive rats that received baroreceptor unloading via sinoaortic denervation (SAD).
Adult male Sprague-Dawley rats (Changchun, China) weighing 220~250 g were used in this study. Experimental animals were divided into two groups: a glutamate receptor antagonist group with SNP-induced hypotension and glutamate receptor agonist group with normal blood pressure. The glutamate receptor antagonist group was subdivided into four groups: microinjection of artificial cerebrospinal fluid into the MVN (ACSF+SNP), microinjection of lidocaine into the MVN (Lido+SNP), microinjection of MK801 into the MVN (MK801+SNP), and microinjection of CNQX into the MVN (CNQX+MVN). The glutamate receptor agonist group was subdivided into three groups: microinjection of artificial cerebrospinal fluid into the MVN (ACSF), microinjection of NMDA into the MVN (NMDA), microinjection of AMPA into the MVN (AMPA). The number of animals in each subgroup was six. Efforts were made to minimize the number of animals used and their suffering. All animal protocols and procedures were performed in accordance to the rules and regulations by the Animal Research Committee of the Yanbian University.
After anesthesia with isoflurane (Ilsung Co., Seoul, Korea), the carotid sinus nerve was sectioned bilaterally following a midventral incision in the neck. The internal, external, and common carotid arteries were stripped of connective tissue at the level of bifurcation and painted with 10% phenylethanol to denervate the carotid sinus. For aortic arch denervation, the aortic arch nerve was severed bilaterally proximal to its junction with the vagus nerve [19]. After surgery, the animals were breathing spontaneously without significant changes in respiratory rhythm. SAD was performed 48 hours prior to experimentation.
Two heparinized polyethylene tubes were inserted, one into the femoral artery to record blood pressure and one into the femoral vein for sodium nitroprusside (SNP) infusion, under isoflurane anesthesia. The tubes were guided toward the skull percutaneously, fixed into the skull, and connected to the tubes of a cybernation metabolism cage to allow free movement in a conscious state during the experiment. Blood pressure was recorded unilaterally from the femoral artery using a pressure transducer and physiography (Grass model 7400; USA). SNP was infused for 1 min at a dose of 15 µg·kg-1·min-1, decreasing blood pressure by 30~40 mmHg during this period.
The rats were anesthetized with isoflurane and placed in a stereotaxic frame (Narishige, Japan). The skull was exposed and a trocar sheath for microinjection (Terumo, Japan) was stereotaxically implanted into the left MVN [20]. The two polyethylene tubes inserted into the femoral vessels and a syringe for microinjection were connected to the tubes of the cybernation metabolism cage to allow free movement in a conscious state during the experiment. The glutamate receptor antagonist group received an intranuclear microinjection of either MK-801 or CNQX (1 mM, 10 µl) and an antagonist control group received an intranuclear microinjection of ACSF or lidocaine at the same dose. The glutamate receptor agonist group received an intranuclear microinjection of either N-methyl D-asparate (NMDA) or 2-amino-3-(5-methyl-3-oxo-1,2-oxazol-4-yl)propanoic acid (AMPA) (1 mM, 10 µl), and an agonist control group received an intranuclear microinjection of ACSF at the same dose. Microinjections were performed by a micromanipulator and all rats were conscious at the time of injection. Ten minutes after intranuclear microinjection, acute hypotension was induced by SNP.
An immunohistochemical analysis of pERK and c-Fos protein expression was performed as described previously [14,21]. After deep anesthesia with an intraperitoneal injection of sodium pentobarbital (100 mg·kg-1), animals were sacrificed for immunohistochemical analysis of ERK or c-Fos at 10 or 90 min, respectively, following administration of SNP. The rats were perfused transcardially with 500 mL of 0.9% NaCl, fixed with 4% paraformaldehyde, and the sucrose-embedded brain stem was sectioned on a cryostat. After nonspecific binding sites were blocked with normal goat serum, primary anti-rabbit polyclonal anti-pERK 1/2 antibody (1:1000) or anti-c-Fos antibody (1:400) (Cell Signaling Technology; MA, USA) was applied to tissue sections overnight at 4℃. Thereafter, tissue sections were incubated with a biotinylated goat anti-rabbit secondary antibody (1:200; Vector Lab., Burlingame, CA, USA) and then an avidin-biotin complex. The bound complex was visualized by incubating the tissue with diaminobenzidine plus H2O2. Sections were then dehydrated, cleared in xylene, and cover-slipped with Permount (Fisher Scientific; Pittsburgh, PA, USA). For quantification, pERK 1/2-immunopositive neurons or c-Fos positive neurons in the left NTS were counted using a digital image analysis system (Image-Pro; Media Cybernetics; MD, USA) at five different levels in a rostral-to-caudal continuum [20].
Stat View version 11.5 software for SPSS (SPSS Inc., USA) was used for all statistical procedures. All data are expressed as the mean±standard deviation (SD). The statistical significance of differences was assessed using multivariate analysis of variance (MANOVA). Values of p<0.05 were considered statistically significant.
Blood pressure was measured after microinjection of glutamate receptor agonists or antagonists into the vestibular nuclei to investigate the effect of glutamate on the regulation of blood pressure through the vestibulo-solitary projection in SAD rats. The basal range of systolic blood pressure was 110~120 mmHg and diastolic blood pressure was 70~85 mmHg. After microinjection of ACSF into the MVN as a control, intravenous infusion of SNP decreased blood pressure within 1 min of the infusion start, and hypotension was maintained for 2 min after the infusion. After microinjection of lidocaine into the MVN as a pretreatment, intravenous infusion of SNP induced hypotension, which was similar to the control. After pretreatment with the glutamate receptor antagonist MK801 or CNQX, hypotension was induced by intravenous infusion of SNP, which was not different from the control. NMDA or AMPA was injected into the MVN to investigate the effect of glutamate receptor agonists in the vestibular nuclei on systemic blood pressure. Microinjection of ACSF into the MVN as a control had no effect on changes in blood pressure. In addition, blood pressure was not changed by microinjection of the glutamate agonists NMDA or AMPA into the MVN (Fig. 1).
In our previous study, expression of pERK in the NTS peaked at 10 min after SNP-induced hypotension [22], so pERK expression was measured 10 min after SNP infusion or microinjection of glutamate receptor agonist into the MVN. The NTS showed a few pERK-immunoreactive neurons under resting conditions in SAD rats, but SNP-induced hypotension significantly increased the number of pERK-positive NTS neurons to 83.8±9.2. Expression of pERK was detected throughout the rostral-caudal axis of the NTS, but was concentrated in the caudal regions. The pERK-positive neurons were round, triangle, or rod-shaped. After microinjection of lidocaine into the MVN, the number of pERK-positive NTS neurons was significantly decreased to 25.9±4.0 after SNP-induced hypotension (p<0.01, compared with the ACSF+SNP group). In addition, after microinjection of the glutamate receptor antagonist MK801 or CNQX into the MVN, the number of pERK-positive NTS neurons significantly decreased to 36.1±3.4 or 27.1±2.8, respectively, after SNP-induced hypotension (p<0.01, compared with the ACSF+SNP group). In normotensive rats, the number of pERK-positive NTS neurons was 23.8±2.6 after microinjection of ACSF into the MVN. However, microinjection of the glutamate receptor agonist NMDA or AMPA into the MVN significantly increased the number of pERK-positive NTS neurons to 112.5±6.6 or 129.5±7.6, respectively (p<0.01, compared with microinjection of ACSF into the MVN) (Figs. 2 and 3).
Expression of c-Fos protein in the NTS peaked at 90 min after SNP-induced hypotension in our previous study [9], so c-Fos protein expression was measured 90 min after SNP infusion or microinjection of glutamate receptor agonists into the MVN. A few c-Fos-positive neurons were visible in the NTS under resting conditions in SAD rats. SNP-induced hypotension significantly increased the number of c-Fos-positive NTS neurons to 53.6±7.0. Expression of c-Fos protein was detected throughout the rostral-caudal axis of the NTS, but was mostly concentrated in the caudal regions. The c-Fos-positive neurons were round with a dark brown color. After microinjection of lidocaine into the MVN, the number of c-Fos-positive NTS neurons significantly decreased to 22.2±2.8 after SNP-induced hypotension (p<0.01, compared with the ACSF+SNP group). After microinjection of the glutamate receptor antagonist MK801 or CNQX, the number of c-Fos-positive NTS neurons significantly decreased to 32.9±2.8 or 33.5±3.4, respectively, after SNP-induced hypotension (p<0.01, compared with the ACSF+ SNP group). In normotensive rats, the number of c-Fos-positive NTS neurons was 27.9±2.2 after microinjection of ACSF into the MVN. However, microinjection of the glutamate receptor agonist NMDA or AMPA into the MVN in normotensive rats significantly increased the number of c-Fos-positive NTS neurons to 79.9±8.0 or 90.1±7.4, respectively (p<0.01, compared with microinjection of ACSF into the MVN) (Figs. 4 and 5).
The NTS receives primary sensory inputs from cardiovascular, gastrointestinal, and pulmonary afferents, and plays a key role in reflex control of blood pressure [23] via integration of afferent inputs from baroreceptors in the baroreceptor reflex and from the medial vestibular nuclei and inferior vestibular nuclei in the VSR [6]. Our previous study reported that two-thirds of c-Fos protein expressed in the NTS following acute hypotension originates from baroreceptor inputs and one-third originates from vestibular receptor inputs [9]. The SAD model, which involves removal of baroreceptor inputs in this study, is reliable for investigating the role of the vestibular inputs to the NTS in the VSR pathway since the NTS is the primary integrator of baroreceptor and vestibular receptor inputs.
Peripheral vestibular receptors in the VSR pathway for the regulation of blood pressure convey afferent signals to the vestibular nuclei by detection of head movement, and produce sympathetic responses directly through the RVLM or indirectly through the vestibulo-solitary projection [24]. Hypotension induced by SNP or hemorrhage increases glutamate release in the vestibular nuclei, which elicits the sympathetic responses through NMDA and AMPA receptors [13,14,15]. Therefore, the role of glutamate in the vestibular nuclei of the VSR pathway could be a neurochemical basis of pathophysiology in orthostatic hypotension.
Intravenous infusion of SNP reduced blood pressure in this study, which is explained by its release of nitric oxide [25]. SNP-induced hypotension was not blocked by pretreatment with lidocaine or glutamate receptor antagonists in the MVN. Moreover, basal levels of blood pressure were not affected by microinjection of glutamate receptor agonists into the MVN. These results indicate that systemic blood pressure is not affected by microinjection of glutamate into the MVN. Regarding the increases in blood pressure elicited by electrical stimulation of the vestibular nuclei [26], stimulation of the MVN by direct microinjection of glutamate in this study would be too weak to cause blood pressure changes.
SNP-induced hypotension increased the expression of pERK and c-Fos protein in the NTS, which likely resulted from excitation of peripheral vestibular receptors by SNP-induced hypotension; this result is consistent with those of our previous studies [9,22]. pERK and c-Fos protein expression in the NTS following hypotension results from afferent vestibular receptor signals only, not from baroreceptors. Expression of pERK and c-Fos protein in the NTS after pretreatment with lidocaine was not increased following hypotension since excitation of the vestibular nuclei did not occur after hypotension, presumably because lidocaine blocked Na+ channels in the neurons of the vestibular nucleus. This indicates that the NTS is a component of the VSR pathway for the regulation of blood pressure. Therefore, orthostatic hypotension can occur by interruption of the VSR pathway in case of bilateral loss of peripheral vestibular receptors [4] or suppression of bilateral vestibular nucleus function. Pretreatment with glutamate receptor antagonists abolished the expression of pERK and c-Fos protein in the NTS following hypotension, which indicates that both NMDA and AMPA receptors are involved in the vestibulo-solitary projection. pERK and c-Fos protein were mainly expressed in the caudal portion of the NTS, which is consistent with the fact that the caudal portion of the NTS receives afferent inputs from the MVN and inferior vestibular nuclei [7].
On the contrary, microinjection of glutamate receptor agonists into the MVN increased the expression of pERK and c-Fos protein in the NTS. It is postulated that glutamate receptor agonists elicit direct excitation of the vestibular nuclei, and then excitatory signals from the vestibular nuclei are transmitted to the NTS, although microinjection of glutamate receptor agonists into the MVN did not affect changes in blood pressure. pERK and c-Fos protein expression was localized in the caudal portion of the NTS, and microinjection of NMDA or AMPA into the MVN increased their expression in the NTS, which also indicates the significance of both NMDA and AMPA receptors in the vestibulo-solitary projection.
pERK and c-Fos protein expression is affected by various stimuli, including anesthetics [27]. In this study, conscious animals were used to prevent potential effects of anesthetics on the expression of these proteins. Control animals without drug treatment showed only a few pERK- or c-Fos-positive neurons in the NTS, which strongly suggests that any kind of external stimuli, including painful or surgical stimulation, did not significantly affect the expression of these proteins during the experiment. However, microinjection of ACSF into the MVN in normotensive rats caused a lot of pERK and c-Fos protein expression in the NTS, which could be due to stimulation of the neurons by the microinjection itself. The number of c-Fos-positive neurons in the NTS was lesser than that of pERK-positive neurons in this study. This could be because c-Fos protein is expressed in the nucleus only, but pERK is expressed in various cellular and subcellular compartments including the nucleus, cytoplasm, axons, and dendrites [17]. On the basis of previous studies in our laboratory, expression of pERK and c-Fos protein peaked at 10 and 90 min, respectively, after hypotension [9,22]. Thus, expression of pERK and c-Fos protein was measured at 10 and 90 min, respectively, after SNP infusion in this study. Simultaneous measurement of both pERK and c-Fos protein could reveal spatiotemporal information regarding the response of NTS neurons to glutamatergic vestibular inputs.
In summary, SNP-induced hypotension increased expression of both pERK and c-Fos protein in the caudal portion of the NTS, which was abolished by pretreatment with glutamate receptor antagonists in the MVN. Microinjection of glutamate receptor agonists into the MVN increased expression of both pERK and c-Fos protein in the NTS without changes in blood pressure. These results indicate that both NMDA and AMPA receptors play a significant role in vestibulo-solitary projection of the VSR pathway for the maintenance of blood pressure, and that glutamatergic transmission in this pathway might play a key role in the pathophysiology of orthostatic hypotension.
ACKNOWLEDGEMENT
This work was supported by grant 31160212 from the National Natural Science Foundation of China.
References
1. Kaufmann H. Consensus statement on the definition of orthostatic hypotension, pure autonomic failure and multiple system atrophy. Clin Auton Res. 1996; 6:125–126. PMID: 8726100.
2. Freeman R. Clinical practice. Neurogenic orthostatic hypotension. N Engl J Med. 2008; 358:615–624. PMID: 18256396.
3. Yates BJ. Vestibular influences on the sympathetic nervous system. Brain Res Brain Res Rev. 1992; 17:51–59. PMID: 1638275.


4. Doba N, Reis DJ. Role of the cerebellum and the vestibular apparatus in regulation of orthostatic reflexes in the cat. Circ Res. 1974; 40:9–18.


5. Dampney RA, Polson JW, Potts PD, Hirooka Y, Horiuchi J. Functional organization of brain pathways subserving the baroreceptor reflex: studies in conscious animals using immediate early gene expression. Cell Mol Neurobiol. 2003; 23:597–616. PMID: 14514018.
6. Balaban CD, Beryozkin G. Vestibular nucleus projections to nucleus tractus solitarius and the dorsal motor nucleus of the vagus nerve: potential substrates for vestibulo-autonomic interactions. Exp Brain Res. 1994; 98:200–212. PMID: 8050507.


7. Yates BJ, Grélot L, Kerman IA, Balaban CD, Jakus J, Miller AD. Organization of vestibular inputs to nucleus tractus solitarius and adjacent structures in cat brain stem. Am J Physiol. 1994; 267:R974–R983. PMID: 7524372.


8. Lan Y, Yang YZ, Jiang X, Li LW, Jin GS, Kim MS, Park BR, Jin YZ. Additive role of the vestibular end organ and baroreceptors on the regulation of blood pressure in rats. Korean J Physiol Pharmacol. 2013; 17:367–373. PMID: 23946697.


9. Jiang X, Li LW, Lan Y, Yang YZ, Jin GS, Kim MS, Park BR, Jin YZ. Comparative analysis of vestibular receptor and baroreceptor inputs to the nucleus tractus solitarius following acute hypotension in conscious rats. Neurosci Lett. 2014; 563:70–74. PMID: 24486893.


10. Weston M, Wang H, Stornetta RL, Sevigny CP, Guyenet PG. Fos expression by glutamatergic neurons of the solitary tract nucleus after phenylephrine-induced hypertension in rats. J Comp Neurol. 2003; 460:525–541. PMID: 12717712.


11. Park BR, Kim MS, Kim JH, Jin YZ. Effects of acute hypotension on neuronal activity in the medial vestibular nuclei of rats. Neuroreport. 2001; 12:3821–3824. PMID: 11726802.


12. Kim MS, Hyo Kim J, Kry D, Ae Choi M, Ok Choi D, Gon Cho B, Jin YZ, Ho Lee S, Park BR. Effects of acute hypotension on expression of cFos-like protein in the vestibular nuclei of rats. Brain Res. 2003; 962:111–121. PMID: 12543461.


13. Choi MA, Lee JH, Hwang JH, Choi SJ, Kim MS, Park BR. Signaling pathway of glutamate in the vestibular nuclei following acute hypotension in rats. Brain Res. 2008; 1229:111–117. PMID: 18639534.


14. Li XL, Nian B, Jin Y, Li LW, Jin GS, Kim MS, Park BR, Jin YZ. Mechanism of glutamate receptor for excitation of medial vestibular nucleus induced by acute hypotension. Brain Res. 2012; 1443:27–33. PMID: 22305141.


15. Li XL, An Y, Jin QH, Kim MS, Park BR, Jin YZ. Changes of some amino acid concentrations in the medial vestibular nucleus of conscious rats following acute hypotension. Neurosci Lett. 2010; 477:11–14. PMID: 20399837.


16. Morgan JI, Curran T. Stimulus-transcription coupling in the nervous system: involvement of the inducible proto-oncogenes fos and jun. Annu Rev Neurosci. 1991; 14:421–451. PMID: 1903243.


17. Sgambato V, Pagès C, Rogard M, Besson MJ, Caboche J. Extracellular signal-regulated kinase (ERK) controls immediate early gene induction on corticostriatal stimulation. J Neurosci. 1998; 18:8814–8825. PMID: 9786988.


18. Chan RK, Peto CA, Sawchenko PE. Fine structure and plasticity of barosensitive neurons in the nucleus of solitary tract. J Comp Neurol. 2000; 422:338–351. PMID: 10861511.


19. Wei S, Lei M, Tong M, Ding J, Han Q, Xiao M. Acute baroreceptor unloading evokes Fos expression in anesthetized rat brain. Brain Res Bull. 2008; 76:63–69. PMID: 18395612.


20. Paxinos G, Watson C. The Rat Brain in Stereotaxic Coordinates. Orlando, FL, USA: Academic Press;2007.
21. Kim MS, Choi DO, Choi MA, Kim JH, Kim KY, Lee MY, Rhee JK, Chun SW, Park BR. Immunohistochemical detection of phosphorylated form of extracellular signal-regulated kinase 1/2 in rat vestibular nuclei following hemorrhagic hypotension. Neurosci Lett. 2004; 360:49–52. PMID: 15082176.


22. Jiang X, Lan Y, Jin YZ, Park JY, Park BG, Ameer AN, Park BR. Effect of vestibulosympathetic reflex and baroreflex on expression of pERK in the nucleus tractus solitarius following acute hypotension in conscious rats. Korean J Physiol Pharmacol. 2014; 18:353–358. PMID: 25177169.


23. Kalia M, Mesulam MM. Brain stem projections of sensory and motor components of the vagus complex in the cat: II. Laryngeal, tracheobronchial, pulmonary, cardiac, and gastrointestinal branches. J Comp Neurol. 1980; 193:467–508. PMID: 7440778.


24. Holstein GR, Friedrich VL Jr, Martinelli GP. Projection neurons of the vestibulo-sympathetic reflex pathway. J Comp Neurol. 2014; 522:2053–2074. PMID: 24323841.


25. Murad F. Cyclic guanosine monophosphate as a mediator of vasodilation. J Clin Invest. 1986; 78:1–5. PMID: 2873150.


26. Kerman IA, Yates BJ. Regional and functional differences in the distribution of vestibulosympathetic reflexes. Am J Physiol. 1998; 275:R824–R835. PMID: 9728081.
27. Takayama K, Suzuki T, Miura M. The comparison of effects of various anesthetics on expression of Fos protein in the rat brain. Neurosci Lett. 1994; 176:59–62. PMID: 7970238.


Fig. 1
Changes in blood pressure after microinjection of glutamate receptor antagonists or agonists into the medial vestibular nuclei (MVN). (A) Effect of pretreatment with glutamate receptor antagonists on SNP-induced hypotension. Intravenous infusion of SNP (15 µg·kg-1·min-1) reduced blood pressure. Pretreatment with artificial cerebrospinal fluid (ACSF), lidocaine (Lido), or the glutamate receptor antagonist MK801 or CNQX in the MVN did not abolish SNP-induced hypotension. (B) Effect of glutamate receptor agonists on blood pressure. Microinjection of ACSF or the glutamate receptor agonist NMDA or AMPA into the MVN had no effect on changes in blood pressure.
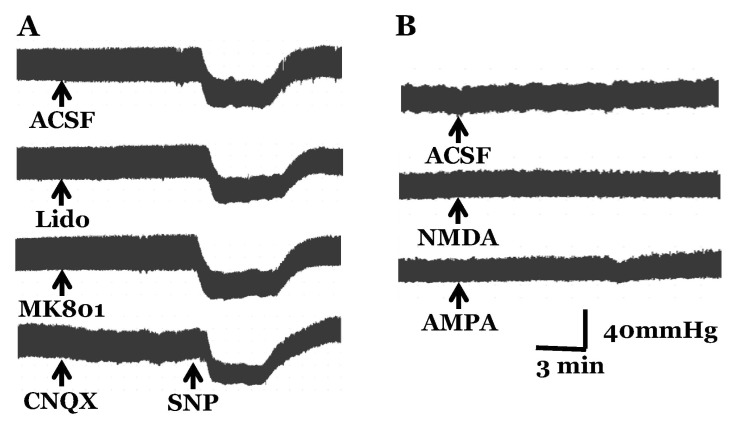
Fig. 2
Photomicrographs showing the effect of glutamate on pERK expression in the nucleus tractus solitarius (NTS). (A) Effect of pretreatment with glutamate receptor antagonists on pERK expression in the NTS. SNP-induced hypotension after pretreatment with artificial cerebrospinal fluid (ACSF) increased pERK expression in the NTS. Pretreatment with lidocaine or the glutamate receptor antagonist MK801 or CNQX in the MVN attenuated pERK expression in the NTS caused by SNP infusion. ACSF+SNP, SNP infusion after pretreatment with ACSF in the MVN; Lido+SNP, SNP infusion after pretreatment with lidocaine in the MVN; MK801+SNP, SNP infusion after pretreatment with MK801 in the MVN; CNQX+SNP, SNP infusion after pretreatment with CNQX in the MVN. Rectangle represents higher magnification of the NTS. (B) Effect of glutamate receptor agonists on pERK expression in the NTS. Microinjection of the glutamate receptor agonist NMDA or AMPA into the MVN increased pERK expression in the NTS. pERK expression was measured 10 min after SNP infusion or microinjection of glutamate agonist into the MVN. ACSF, microinjection of ACSF into the MVN; NMDA, microinjection of NMDA into the MVN; AMPA, microinjection of AMPA into the MVN. NTS, nucleus tractus solitarius (the coordinate: 0.2 mm rostral to the obex). Scale bar=200 µm. In right upper quadrant of B, a and b represent higher magnification of the NTS in NMDA and AMPA, respectively (Scale bar=50 µm).
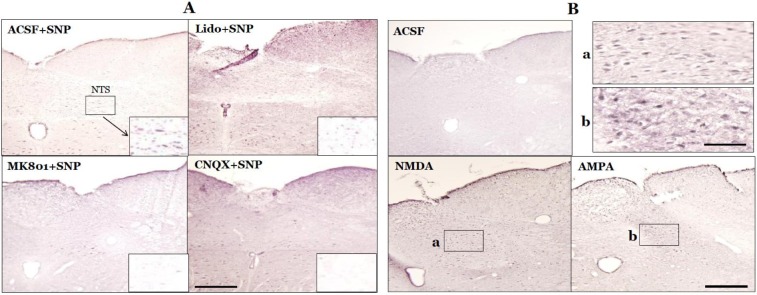
Fig. 3
Bar histograms showing the effect of glutamate on pERK expression in the nucleus tractus solitarius. ACSF+SNP, SNP infusion after pretreatment with ACSF in the MVN; Lido+SNP, SNP infusion after pretreatment with lidocaine in the MVN; MK801+SNP, SNP infusion after pretreatment with MK801 in the MVN; CNQX+SNP, SNP infusion after pretreatment with CNQX in the MVN; ACSF, microinjection of ACSF into the MVN; NMDA, microinjection of NMDA into the MVN; AMPA, microinjection of AMPA into the MVN. The number of animals in each group was six. Values are mean±SD. *Significant difference from ACSF+SNP group (**p<0.01). †Significant difference from ACSF group (‡p<0.01).
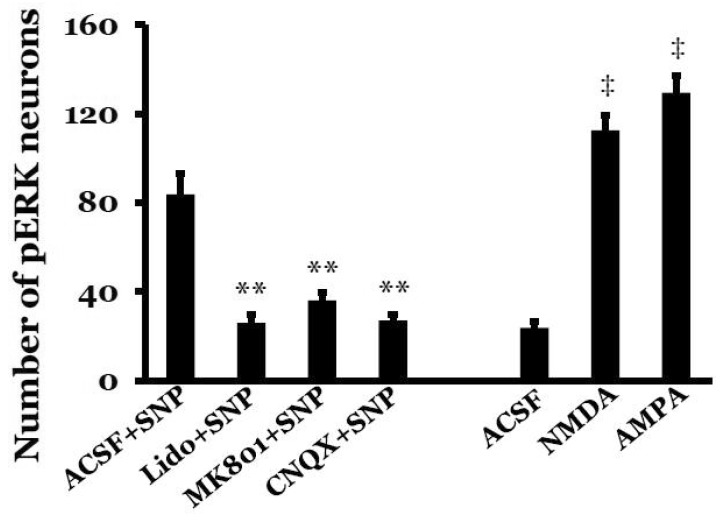
Fig. 4
Photomicrographs showing the effect of glutamate on c-Fos protein expression in the nucleus tractus solitarius (NTS). (A) Effect of pretreatment with glutamate receptor antagonists on c-Fos protein expression in the NTS. SNP-induced hypotension after pretreatment with artificial cerebrospinal fluid (ACSF) increased c-Fos protein expression in the NTS. Pretreatment with lidocaine or the glutamate antagonist MK801 or CNQX in the MVN attenuated c-Fos protein expression in the NTS caused by SNP infusion. ACSF+SNP, SNP infusion after pretreatment with ACSF in the MVN; Lido+SNP, SNP infusion after pretreatment with lidocaine in the MVN; MK801+SNP, SNP infusion after pretreatment with MK801 in the MVN; CNQX+SNP, SNP infusion after pretreatment with CNQX in the MVN. Rectangle represents higher magnification of the NTS. (B) Effect of glutamate receptor agonists on c-Fos protein expression in the NTS. Microinjection of the glutamate agonist NMDA or AMPA into the MVN increased c-Fos protein expression in the NTS. c-Fos protein expression was measured 90 min after SNP infusion or microinjection of glutamate agonist into the MVN. ACSF, microinjection of ACSF into the MVN; NMDA, microinjection of NMDA into the MVN; AMPA, microinjection of AMPA into the MVN. NTS, nucleus tractus solitarius (the coordinate: 0.2 mm rostral to the obex). Scale bar=200 µm. In right upper quadrant of B, a and b represent higher magnification of the NTS in NMDA and AMPA, respectively (Scale bar=50 µm).
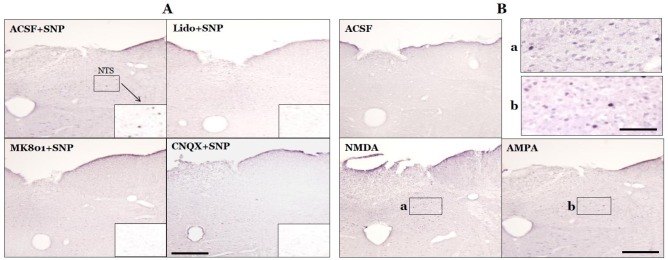
Fig. 5
Bar histograms showing the effect of glutamate on c-Fos protein expression in the nucleus tractus solitarius. ACSF+SNP, SNP infusion after pretreatment with ACSF in the MVN; Lido+SNP, SNP infusion after pretreatment with lidocaine in the MVN; MK801+SNP, SNP infusion after pretreatment with MK801 in the MVN; CNQX+SNP, SNP infusion after pretreatment with CNQX in the MVN; ACSF, microinjection of ACSF into the MVN; NMDA, microinjection of NMDA into the MVN; AMPA, microinjection of AMPA into the MVN. The number of animals in each group was six. Values are mean±SD. *Significant difference from ACSF+SNP group (**p<0.01). †Significant difference from ACSF group (‡p<0.01).
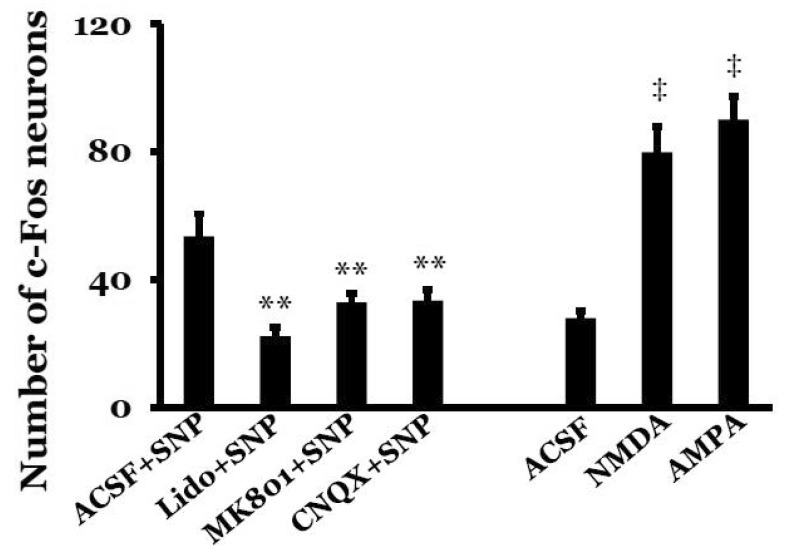