Abstract
Extracellular nicotinamide adenine dinucleotide (NAD) cleaving activity of a particular cell type determines the rate of the degradation of extracellular NAD with formation of metabolites in the vicinity of the plasma membrane, which has important physiological consequences. It is yet to be elucidated whether intact human neutrophils have any extracellular NAD cleaving activity. In this study, with a simple fluorometric assay utilizing 1,N6-ethenoadenine dinucleotide (etheno-NAD) as the substrate, we have shown that intact peripheral human neutrophils have scant extracellular etheno-NAD cleaving activity, which is much less than that of mouse bone marrow neutrophils, mouse peripheral neutrophils, human monocytes and lymphocytes. With high performance liquid chromatography (HPLC), we have identified that ADP-ribose (ADPR) is the major extracellular metabolite of NAD degradation by intact human neutrophils. The scant extracellular etheno-NAD cleaving activity is decreased further by N-formyl-methionine-leucine-phenylalanine (fMLP), a chemoattractant for neutrophils. The fMLP-mediated decrease in the extracellular etheno-NAD cleaving activity is reversed by WRW4, a potent FPRL1 antagonist. These findings show that a much less extracellular etheno-NAD cleaving activity of intact human neutrophils compared to other immune cell types is down-regulated by fMLP via a low affinity fMLP receptor FPRL1.
In addition to its major role in the regulation of cellular redox-related metabolism, nicotinamide adenine dinucleotide (NAD) and its metabolites have been found to be important for various cellular signaling processes [1]. NAD can be metabolized extracellularly in a number of different ways by cell surface enzymes. Cell surface NAD glycohydrolases [2,3,4] and ADP-ribosyltransferases [5,6] cleave NAD at the N-glycosidic bond to produce ADP-ribose (ADPR) and nicotinamide. Cleavage by NAD glycohydrolases produces free ADPR, and ADP-ribosyltransferases transfer ADPR to an acceptor molecule. Another family of cell surface extracellular NAD cleaving enzymes is pyrophosphatase that can cleave NAD directly to adenosine monophosphate and nicotinamide mononucleotide [7].
Extracellular NAD cleaving activity of a particular cell type is physiologically important as it determines the rate of the degradation of extracellular NAD with formation of metabolites in the vicinity of the plasma membrane, indirectly determining the interaction of the cells with extracellular NAD or with its metabolites. Extracellular application of NAD or its metabolites, especially ADPR and cyclic ADPR (cADPR), reportedly affect intracellular signaling of several cell types: extracellular NAD increases intracellular free calcium concentration ([Ca2+]i) in human neutrophils [8], and human monocytes, where ADPR was also effective [9]. Further, extracellular cADPR increases [Ca2+]i and stimulates proliferation of human hemopoietic progenitors [10]. Thus, extracellular NAD cleaving activity depending on the cell types might have physiological meaning, and deserves substantial consideration to study.
However, it is yet to be clarified whether intact human neutrophils have extracellular NAD cleaving activity. Furthermore, previously no study showed the comparison of extracellular NAD cleaving activity of intact human neutrophils with other immune cell types. In this study, with a simple fluorometric assay utilizing 1,N6-ethenoadenine dinucleotide (etheno-NAD) as the substrate, we have shown that intact human peripheral neutrophils have scant extracellular etheno-NAD cleaving activity which is much less than that of mouse bone marrow neutrophils, mouse peripheral neutrophils, human monocytes and lymphocytes. With high performance liquid chromatography (HPLC), it was identified that ADPR is the major extracellular metabolite of NAD degradation by human neutrophils. Furthermore, the scant extracellular etheno-NAD cleaving activity of intact human neutrophils is down-regulated by fMLP via the low affinity fMLP receptor FPRL1.
Etheno-NAD was obtained from Sigma-Aldrich Chemical, and 20 mM stock solution was prepared in 10 mM potassium phosphate buffer (pH 7.4). fMLP and retinoic acid were also from Sigma-Aldrich Chemical. WRW4 was from Tocris Bioscience (Bristol, UK).
Neutrophils were purified from venous blood of healthy volunteer. In brief, venous blood was collected with peripheral venous puncture and immediately anti-coagulated with 10 U/ml sodium heparin. Then, neutrophils were isolated by density gradient centrifugation in Histopaque-1077, followed by dextran sedimentation. Residual erythrocytes were eliminated with hypotonic lysis. The purity of neutrophils counted by Diff Quik staining was >95% average. Eosinophils were found to be <5%. The viability of neutrophils stained with tryphan blue was >99%.
Procedures for animal experiments were approved by the Animal Experimentation Committee at Hallym University. C57BL/6J female mice were sacrificed by cervical dislocation, and their femurs and tibiae were carefully cleaned from adherent tissues. After bone ends were cut off, the marrow was collected. Residual erythrocytes were eliminated with hypotonic lysis. The bone marrow neutrophils were then isolated by density gradient centrifugation in Percoll and suspended at a density of 1×107 cells/ml in DMEM containing 10% FBS, 100 U/ml penicillin and 100 U/ml streptomycin. The purity of neutrophils counted by Giemsa staining was >90% average. Cultures were kept at 37℃ in a humidified atmosphere containing 95% air and 5% CO2.
Mouse peripheral neutrophils were isolated according to the manufacturer's protocol (mouse neutrophil isolation kit, MACS Miltenyl Biotec, Germany). Briefly, peripheral blood was collected from C57BL/6J female mice and immediately anti-coagulated with 10 U/ml sodium heparin. Erythrocytes were eliminated from the whole blood cells by lysis with BD Pharm Lyse™ followed by hypotonic lysis. Then, leukocytes were treated with neutrophil biotin-antibody cocktail, and neutrophils were selectively isolated by magnetic separation with LS column.
Monocytes and lymphocytes were isolated as described previously [11]. For monocyte isolation, peripheral blood mononuclear cells (PBMC) from healthy donors were isolated from buffy coats obtained by density sedimentation over Histopaque-1077. Residual erythrocytes were eliminated with hypotonic lysis, and then to separate monocytes from lymphocytes, PBMC suspension was carefully laid onto the hyper-osmotic Percoll solution in each tube and centrifuged with brake off. Monocytes layer at the interface was collected and suspended in RPMI-1640. To obtain lymphocytes, after collecting the monocyte fraction at the interface, the bottom layer was collected and after washing with PBS, the residual sediments were suspended in RPMI-1640 supplemented with 5% FBS.
HL-60 (a promyelocytic leukemic cell line), U937 (a promonocytic tumor cell line) and Jurkat (a T lymphocyte cell line) cells were cultured in RPMI-1640 supplemented with 10% FBS, 100 U/ml penicillin and 100 U/ml streptomycin and kept in a 5% CO2 humidified chamber. To differentiate HL-60 cells into the neutrophil lineage by dimethyl sulphoxide (DMSO), cells at a density of 0.3×106 cells/ml were grown in the presence of 1.3% DMSO for 3 days. Afterwards, the medium was replaced by fresh medium containing 0.65% DMSO and the culture continued for 3 more days. To differentiate HL-60 cells into the neutrophil lineage by retinoic acid, cells at a density of 0.2×106 cells/ml were grown in the presence of 100 nM retinoic acid for 4 days as described previously [12].
Etheno-NAD cleaving activity was measured by a fluorimetric assay with etheno-NAD as the substrate [2]. Neutrophils or other immune cell types were suspended in Hank's balanced salt solution (HBSS) (in mM) (KCl 5.4, Na2HPO4 0.338, KH2PO4 0.44, NaHCO3 4.2, CaCl2 1.3, MgCl2 0.5, MgSO4 0.6, NaCl 137, D-glucose 5.6) and seeded to 96 well-plate at a cell density of 1×107 cells/ml. Substrate etheno-NAD (final concentration 20 µM) was added following 5 min pre-read. Cleavage of etheno-NAD, which causes a 10-fold fluorescence enhancement, was continuously followed at 37℃ using Spectramax M2/e fluorescence microplate reader (Molecular Devices) at an excitation of 300 nm and an emission wave length of 410 nm.
Human peripheral neutrophils at a density of 1×107 cells/ml and mouse bone marrow neutrophils at a density of 3×106 cells/ml were incubated with or without NAD (1 µM) and incubated for 15 min or 1 hour at 37℃. Then the extracellular media were collected after centrifugation. Aliquots were analyzed by reverse-phase HPLC (Jasco Instruments) using a C18 column (4.6×250 mm, particle size 5 µm). Absorbance was measured at 254 nm using a UV detector (Jasco UV-2075 plus intelligent UV/VIS detector) and data were processed by the chromNAV data acquisition system from Jasco instruments. Peaks were identified by comparison to known standards.
Funaro et al. reported that human neutrophils are inactive in terms of extracellular ADP-ribosylcyclase activity [13]. However, there is no clear indication whether intact human neutrophils have any other extracellular NAD cleaving activities. To address this uncertainty, we undertook a simple fluorometric assay using etheno-NAD. As shown in Fig. 1A, intact human peripheral neutrophils showed scant extracellular etheno-NAD cleaving activity, which is much less than that of mouse (either bone marrow or peripheral) neurophils. It is to be noted that mature mouse peripheral neutrophils have lower extracellular etheno-NAD cleaving activity than immature mouse bone marrow neutrophils (Fig. 1A). Also, there is no data until now comparing the extracellular NAD cleaving activity of intact human peripheral neutrophils with other immune cell types. Thus next, we compared extracellular etheno-NAD cleaving activity of human neutrophils with different human primary immune cells like monocytes and lymphocytes. As shown in Fig. 1B, human peripheral neutrophils showed a much less extracellular etheno-NAD cleaving activity compared to monocytes and lymphocytes. We also compared extracellular etheno-NAD cleaving activity of DMSO- and retinoic acid-differentiated neutrophillike HL-60 cells with U937 and Jurkat T cells to check whether the cell lines show the similar observation. As expected, DMSO-differentiated neutrophil-like HL-60 cells were devoid of extracellular etheno-NAD metabolizing activity, whilst U937 and Jurkat T cells, like primary human monocytes and lymphocytes, respectively, showed marked extracellular etheno-NAD metabolizing activity (Fig. 1B). However, retinoic acid-treated HL-60 cells showed a marked increase in extracellular etheno-NAD cleaving activity (Fig. 1B) as reported previously [14].
Next, we attempted to identify the extracellular metabolites generated from extracellular NAD degradation by human peripheral neutrophils and mouse bone marrow neutrophils with HPLC. As shown in Fig. 2, the major extracellular metabolite generated from extracellular NAD degradation by both human peripheral neutrophils and mouse bone marrow neutrophils was ADPR. Extracellular NAD degradation by human neutrophils, as expected, was scant following incubation of NAD with human neutrophils for 15 minutes or 1 hour (Fig. 2A). Though about three times less mouse bone marrow neutrophils (3×106 cells/ml) than human neutrophils (1×107 cells/ml) were used, degradation of extracellular NAD by mouse bone marrow neutrophils was remarkable (Fig. 2B).
Next, we investigated whether the scant extracellular etheno-NAD cleaving activity of human neutrophils is regulated following activation with fMLP, a chemoattractant for neutrophils. Since fMLP-like molecules could be abundant at bacterial infection sites, we investigated whether the extracellular etheno-NAD cleaving activity of intact human neutrophils are affected following fMLP stimulation. As shown in Fig. 3A, stimulation with fMLP induced a concentration-dependent decrease in the extracellular etheno-NAD cleaving activity of intact human neutrophils, which became significant at 100 nM, and was more marked at 1 µM concentration. In the next experiment, it was of interest that whether fMLP can affect extracellular etheno-NAD cleaving activity in other cells such as monocytes. Monocytes, however, was found to be unaffected by fMLP (Fig. 3B).
Human neutrophils express two types of fMLP receptors. The prototype receptor formyl peptide receptor (FPR) is activated by low concentration (in the picomolar to low nanomolar range) of fMLP and is considered a high affinity fMLP receptor. An FPR variant, FPR-like 1 (FPRL1), interacts with high concentrations (in the micromolar range) of fMLP and is defined as a low affinity fMLP receptor [15].
Since fMLP, at micromolar concentration showed the maximal inhibition in the extracellular etheno-NAD cleaving activity of intact human neutrophils (Fig. 3A), we hypothesized that fMLP-induced decrease in the extracellular etheno-NAD cleaving activity might occur through FPRL1. To test this hypothesis, the effect of WRW4, a potent antagonist for FPRL1, on the fMLP-induced effect was examined. WRW4 at 10 µM but not at 1 µM reversed the fMLP-induced decrease in the extracellular etheno-NAD cleaving activity of intact human neutorphils (Fig. 4), suggesting that fMLP-induced decrease in the extracellular etheno-NAD cleaving activity of intact human neutrophils occur via FPRL1.
Neutrophils and monocytes are the major immune cells to be recruited to the site of inflammation [16]. During tissue damage, NAD may be leaked to the extracellular environment [17]. At the inflammatory site, it may also be released from a mechanically stressed cell to the extracellular environment [17]. Thus, released or leaked NAD may impose autocrine or paracrine effects on the intracellular signaling of a variety of cells including human neutrophils [8,9]. Our observation of intact human neutrophils with scant extracellular etheno-NAD cleaving activity (Fig. 1) suggests that extracellular intact NAD than its metabolites might be more important for modulation of human neutrophil functions. This contention is in line with the previous report that extracellular application of as low as 1 µM NAD, but not ADPR, increases [Ca2+]i in human neutrophils [8]. However, about 25 fold higher concentration of NAD is required for [Ca2+]i increase in human monocytes [9]. Our observation indicates that human monocytes have a much higher extracellular NAD metabolizing activity than human neutrophils, with the consequence of a much higher NAD clearance in the vicinity of these cell membranes than that of human neutrophils, and thus, may explain why higher concentration of extracellular NAD is required for [Ca2+]i increase in human monocytes [9] than human neutrophils [8].
Differentiation of monocytes into macrophages is accompanied with down-regulation of CD38 (the major extracellular NADase) expression [18]. Thus, the rather fully differentiated state of peripheral neutrophils could be compatible with the scant extracellular NAD metabolizing activity, compared with other kinds of immune cells. In line with this contention, ethenoNADase activity of mouse peripheral neutrophils was less than that of mouse bone marrow neutrophils (Fig. 1A).
The limitation of this study is lack of definitive evidence for the specific enzyme responsible for the scant extracellular NAD metabolizing activity of human neutrophils. Prototypic members of cell surface NAD glycohydrolases are CD38 and CD157 that show both ADP-ribosylcyclase and cADPRhydrolase activity apart from their intrinsic NAD glycohydrolase activity [13,19,20,21,22,23,24,25]. Presence of enzymatically inactive CD157 in human neutrophils [13], and also our observation of the generation of extracellular ADPR from extracellular NAD degradation by human neutrophils (Fig. 2A) made us assume that CD38 might be involved in the scant extracellular NAD metabolizing activity of human neutrophils. Interestingly, the scant extracellular etheno-NAD cleaving activity of intact human neutrophils was further decreased by fMLP (100 nM~1 µM) (Fig. 3A). It is notable that fMLP-induced CD38 cleavage was previously reported in human neutrophils [26]. However, the phenomenon reported by Fujita et al. occurs at much late time point (~1~2 hr) with 10 nM fMLP. Our study indicates that fMLP-induced decrease in the extracellular etheno-NAD cleaving activity occurs at much earlier time point starting from around 5 min with a relatively higher fMLP concentration (100 nM~1 µM) (Fig. 3A). Further, we were unable to find any changes induced by fMLP (1 µM) in surface expression of CD38 determined by flow cytometry, CD38 expression determined by Western blot, and modification of CD38 by phosphorylation or ubiquitination (data not shown). The molecular mechanism for the fMLP effect (Fig. 3A) as well as the identity of the enzyme for the scant extracellular NAD cleaving activity of human neutrophils needs to be clarified.
To our knowledge, this is the first report that explicitly shows scant extracelluar etheno-NAD cleaving activity of intact human neutrophils compared to other immune cell types, providing the physiological basis for the more sensitive regulation by NAD of human neutrophils compared with other immune cells [8,9]. Interestingly, this scant extracelluar etheno-NAD cleaving activity of human neutrophils was further decreased with fMLP in the WRW4 (an FPRL1 antagonist)-sensitive manner (Fig. 4B). The specific signaling processes downstream to the activation of FPRL1 [27] involved in the fMLP-induced inhibition of etheno-NADase activity remain to be delineated. However, though human monocyte expresses FPRL1 [28], extracellur etheno-NAD cleaving activity of human monocytes was found to be unaffected by micromolar fMLP (Fig. 3B). Extracellullar NAD-induced [Ca2+]i increase in human neutrophils has been shown to translate into neutrophil activation as determined by superoxide and nitric oxide production and chemotaxis [8]. fMLP-like molecules as well as NAD would be abundant at the bacterial infection foci, where neutrophils are highly accumulated. Our observation of fMLP-induced inhibition of extracellular NAD cleaving activity of human neutrophils (Fig. 3A) would thus lead to an enhanced activation of neutrophils via NAD in the bacterial infection foci with the beneficial effect on the local bacterial clearance.
ACKNOWLEDGEMENTS
This research was supported by Hallym University Research Fund, 2012 (HRF-201211-017) and Basic Science Research Program through the National Research Foundation of Korea (NRF) funded by the Ministry of Education (NRF-2013R1A1A2063432).
References
1. Grahnert A, Grahnert A, Klein C, Schilling E, Wehrhahn J, Hauschildt S. Review: NAD+: a modulator of immune functions. Innate Immun. 2011; 17:212–233. PMID: 20388721.
2. Muller HM, Muller CD, Schuber F. NAD+ glycohydrolase, an ecto-enzyme of calf spleen cells. Biochem J. 1983; 212:459–464. PMID: 6192807.


3. Pekala PH, Anderson BM. Studies of bovine erythrocyte NAD glycohydrolase. J Biol Chem. 1978; 253:7453–7459. PMID: 212424.


4. Balducci E, Micossi LG. NAD-dependent inhibition of the NADglycohydrolase activity in A549 cells. Mol Cell Biochem. 2002; 233:127–132. PMID: 12083366.
5. Wang J, Nemoto E, Kots AY, Kaslow HR, Dennert G. Regulation of cytotoxic T cells by ecto-nicotinamide adenine dinucleotide (NAD) correlates with cell surface GPI-anchored/arginine ADP-ribosyltransferase. J Immunol. 1994; 153:4048–4058. PMID: 7930612.
6. Okazaki IJ, Zolkiewska A, Takada T, Moss J. Characterization of mammalian ADP-ribosylation cycles. Biochimie. 1995; 77:319–325. PMID: 8527484.


7. Bortell R, Moss J, McKenna RC, Rigby MR, Niedzwiecki D, Stevens LA, Patton WA, Mordes JP, Greiner DL, Rossini AA. Nicotinamide adenine dinucleotide (NAD) and its metabolites inhibit T lymphocyte proliferation: role of cell surface NAD glycohydrolase and pyrophosphatase activities. J Immunol. 2001; 167:2049–2059. PMID: 11489987.


8. Bruzzone S, Moreschi I, Guida L, Usai C, Zocchi E, De Flora A. Extracellular NAD+ regulates intracellular calcium levels and induces activation of human granulocytes. Biochem J. 2006; 393:697–704. PMID: 16225456.


9. Gerth A, Nieber K, Oppenheimer NJ, Hauschildt S. Extracellular NAD+ regulates intracellular free calcium concentration in human monocytes. Biochem J. 2004; 382:849–856. PMID: 15233622.


10. Podestà M, Zocchi E, Pitto A, Usai C, Franco L, Bruzzone S, Guida L, Bacigalupo A, Scadden DT, Walseth TF, De Flora A, Daga A. Extracellular cyclic ADP-ribose increases intracellular free calcium concentration and stimulates proliferation of human hemopoietic progenitors. FASEB J. 2000; 14:680–690. PMID: 10744625.


11. Repnik U, Knezevic M, Jeras M. Simple and cost-effective isolation of monocytes from buffy coats. J Immunol Methods. 2003; 278:283–292. PMID: 12957415.


12. Porfiri E, Hoffbrand AV, Wickremasinghe RG. Retinoic acidinduced granulocytic differentiation of HL60 human promyelocytic leukemia cells is preceded by downregulation of autonomous generation of inositol lipid-derived second messengers. Blood. 1991; 78:1069–1077. PMID: 1651133.


13. Funaro A, Ortolan E, Ferranti B, Gargiulo L, Notaro R, Luzzatto L, Malavasi F. CD157 is an important mediator of neutrophil adhesion and migration. Blood. 2004; 104:4269–4278. PMID: 15328157.


14. Kontani K, Nishina H, Ohoka Y, Takahashi K, Katada T. NAD glycohydrolase specifically induced by retinoic acid in human leukemic HL-60 cells. Identification of the NAD glycohydrolase as leukocyte cell surface antigen CD38. J Biol Chem. 1993; 268:16895–16898. PMID: 8394323.


15. Le Y, Murphy PM, Wang JM. Formyl-peptide receptors revisited. Trends Immunol. 2002; 23:541–548. PMID: 12401407.


16. Jung YY, Nam Y, Park YS, Lee HS, Hong SA, Kim BK, Park ES, Chung YH, Jeong JH. Protective effect of phosphatidylcholine on lipopolysaccharide-induced acute inflammation in multiple organ injury. Korean J Physiol Pharmacol. 2013; 17:209–216. PMID: 23776397.


17. Haag F, Adriouch S, Braβ A, Jung C, Möller S, Scheuplein F, Bannas P, Seman M, Koch-Nolte F. Extracellular NAD and ATP: Partners in immune cell modulation. Purinergic Signal. 2007; 3:71–81. PMID: 18404420.


18. Pfister M, Ogilvie A, da Silva CP, Grahnert A, Guse AH, Hauschildt S. NAD degradation and regulation of CD38 expression by human monocytes/macrophages. Eur J Biochem. 2001; 268:5601–5608. PMID: 11683883.


19. Takasawa S, Tohgo A, Noguchi N, Koguma T, Nata K, Sugimoto T, Yonekura H, Okamoto H. Synthesis and hydrolysis of cyclic ADP-ribose by human leukocyte antigen CD38 and inhibition of the hydrolysis by ATP. J Biol Chem. 1993; 268:26052–26054. PMID: 8253715.


20. Zocchi E, Franco L, Guida L, Benatti U, Bargellesi A, Malavasi F, Lee HC, De Flora A. A single protein immunologically identified as CD38 displays NAD+ glycohydrolase, ADP-ribosyl cyclase and cyclic ADP-ribose hydrolase activities at the outer surface of human erythrocytes. Biochem Biophys Res Commun. 1993; 196:1459–1465. PMID: 8250903.


21. Howard M, Grimaldi JC, Bazan JF, Lund FE, Santos-Argumedo L, Parkhouse RM, Walseth TF, Lee HC. Formation and hydrolysis of cyclic ADP-ribose catalyzed by lymphocyte antigen CD38. Science. 1993; 262:1056–1059. PMID: 8235624.


22. Berthelier V, Tixier JM, Muller-Steffner H, Schuber F, Deterre P. Human CD38 is an authentic NAD(P)+ glycohydrolase. Biochem J. 1998; 330:1383–1390. PMID: 9494110.


23. Yamamoto-Katayama S, Ariyoshi M, Ishihara K, Hirano T, Jingami H, Morikawa K. Crystallographic studies on human BST-1/CD157 with ADP-ribosyl cyclase and NAD glycohydrolase activities. J Mol Biol. 2002; 316:711–723. PMID: 11866528.


24. Ortolan E, Vacca P, Capobianco A, Armando E, Crivellin F, Horenstein A, Malavasi F. CD157, the Janus of CD38 but with a unique personality. Cell Biochem Funct. 2002; 20:309–322. PMID: 12415565.


25. Ortolan E, Tibaldi EV, Ferranti B, Lavagno L, Garbarino G, Notaro R, Luzzatto L, Malavasi F, Funaro A. CD157 plays a pivotal role in neutrophil transendothelial migration. Blood. 2006; 108:4214–4222. PMID: 16917007.


26. Fujita T, Zawawi KH, Kurihara H, Van Dyke TE. CD38 cleavage in fMLP- and IL-8-induced chemotaxis is dependent on p38 MAP kinase but independent of p44/42 MAP kinase. Cell Signal. 2005; 17:167–175. PMID: 15494208.


27. Selvatici R, Falzarano S, Mollica A, Spisani S. Signal transduction pathways triggered by selective formylpeptide analogues in human neutrophils. Eur J Pharmacol. 2006; 534:1–11. PMID: 16516193.


28. Yang D, Chen Q, Le Y, Wang JM, Oppenheim JJ. Differential regulation of formyl peptide receptor-like 1 expression during the differentiation of monocytes to dendritic cells and macrophages. J Immunol. 2001; 166:4092–4098. PMID: 11238658.


Fig. 1
Intact human peripheral neutrophils show much less extracellular etheno-NAD cleaving activity than other immune cell types. (A) Comparison of extracellular etheno-NAD cleaving activity of human peripheral neutrophils with mouse bone marrow neutrophils and mouse peripheral neutrophils. (B) Comparison of extracellular etheno-NAD cleaving activity of human peripheral neutrophils with other primary immune cells and comparison of neutrophil-like cells with other immune cell lines. Briefly, each cell type was suspended in HBSS and seeded to 96 well-plate at a cell density of 1×107 cells/ml. Substrate etheno-NAD (final concentration 20 µM) was added following 5 min pre-read. Cleavage of etheno-NAD was continuously followed for 15 min as described in Materials and Methods. Activity was defined as the fluorescence change (▴F)/min/2×106 cells. ns, no significant difference; ***p<0.0001.
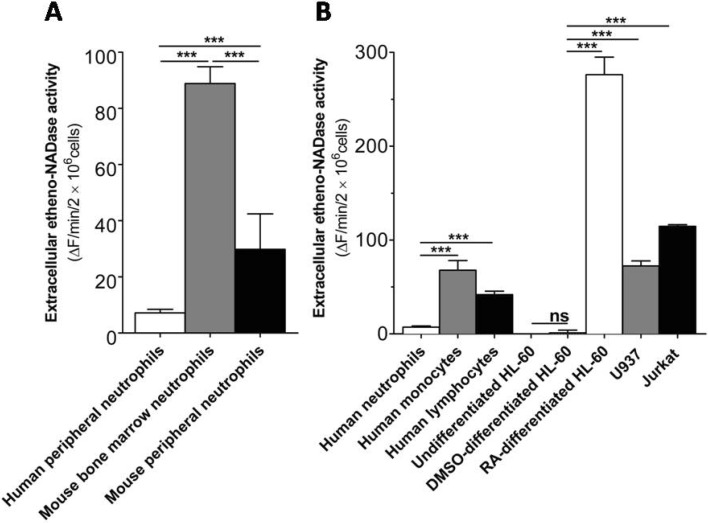
Fig. 2
ADPR is the major extracellular metabolites generated from the degradation of NAD by both intact human peripheral neutrophils and mouse bone marrow neutrophil as determined by HPLC. Human peripheral neutrophils at a density of 1×107 cells/ml (A) and mouse bone marrow neutrophils at a density of 3×106 cells/ml (B) were incubated with or without NAD (1 µM) and incubated for 15 min or 1 hour at 37℃. Then the extracellular media were collected after centrifugation. Aliquots were analyzed by reverse-phase HPLC (Jasco Instruments) as described in Methods.
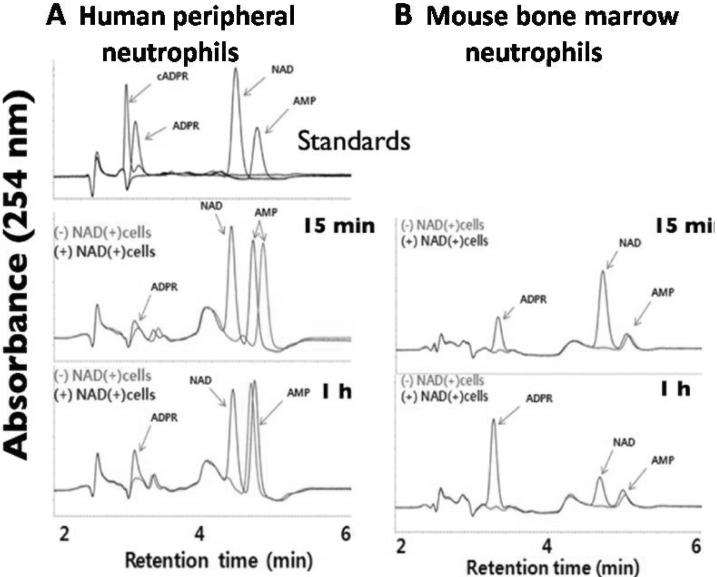
Fig. 3
fMLP induces a decrease in extracellular etheno-NAD cleaving activity of human neutrophils, but not of human monocytes. (A) Human neutrophils and (B) human monocytes were suspended in HBSS and seeded to 96 well-plate at a cell density of 1×107 cells/ml and incubated in the CO2 humidified chamber for 10 min. Then vehicle or fMLP of different concentration was treated. Substrate etheno-NAD (final concentration 20 µM) was added following 5 min pre-read. Cleavage of etheno-NAD was continuously followed at 37℃ for 15 min. Activity was defined as the fluorescence change (▴F)/min/2×106 cells. **p<0.001; ***p<0.0001.

Fig. 4
fMLP-induced decrease in extracellular etheno-NAD cleaving activity of intact human neutrophils is reversed by WRW4, an FPRL1 antagonist. Human neutrophils were suspended in HBSS and seeded to 96 well-plate at a cell density of 1×107 cells/ml. Then, the cells were treated with vehicle or WRW4 (1 µM (A) or 10 µM (B)) for 5 min before the treatment of vehicle or fMLP (1 µM). Substrate etheno-NAD (final concentration 20 µM) was added following 5 min pre-read. Cleavage of etheno-NAD was continuously followed at 37℃ for 15 min. Activity was defined as the fluorescence change (▴F)/min/2×106 cells. ns, no significant difference; **p<0.001; ***p<0.0001.
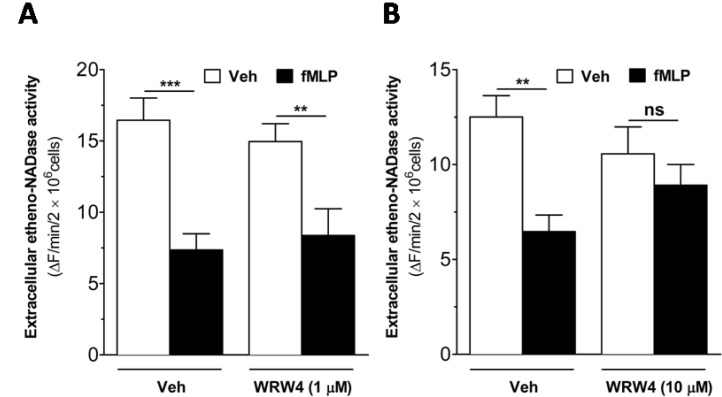