Abstract
Nitric oxide (NO) is recognized as a mediator and regulator of inflammatory responses. NO is produced by nitric oxide synthase (NOS), and NOS is abundantly expressed in the human dental pulp cells (HDPCs). NO produced by NOS can be cytotoxic at higher concentrations to HDPCs. However, the mechanism by which this cytotoxic pathway is activated in cells exposed to NO is not known. The purpose of this study was to elucidate the NO-induced cytotoxic mechanism in HDPCs. Sodium nitroprusside (SNP), a NO donor, reduced the viability of HDPCs in a dose- and time-dependent manner. We investigated the in vitro effects of nitric oxide on apoptosis of cultured HDPCs. Cells showed typical apoptotic morphology after exposure to SNP. Besides, the number of Annexin V positive cells was increased among the SNP-treated HDPCs. SNP enhanced the production of reactive oxygen species (ROS), and N-acetylcysteine (NAC) ameliorated the decrement of cell viability induced by SNP. However, a soluble guanylate cyclase inhibitor (ODQ) did not inhibited the decrement of cell viability induced by SNP. SNP increased cytochrome c release from the mitochondria to the cytosol and the ratio of Bax/Bcl-2 expression levels. Moreover, SNP-treated HDPCs elevated activities of caspase-3 and caspase-9. While pretreatment with inhibitors of caspase (z-VAD-fmk, z-DEVD-fmk) reversed the NO-induced apoptosis of HDPCs. From these results, it can be suggested that NO induces apoptosis of HDPCs through the mitochondria-dependent pathway mediated by ROS and Bcl-2 family, but not by the cyclic GMP pathway.
Dental pulp inflammation (pulpitis) is caused mainly by bacterial infections of the dentin or root canal [1]. In addition to caries-associated bacteria, pulp exposure to a chemical stimulus, mechanical stimulus, and trauma can trigger an inflammatory response [2]. Inflammation is a multifaceted response mediated by the activation of cells of the immune system. Cells of the immune system produce nitric oxide (NO) during inflammatory processes [3]. NO is a short lived, highly reactive free radical gas synthesized by nitric oxide synthase (NOS) through conversion of the guanidinic group of L-arginine to citrulline [4,5]. NO is a gaseous signaling molecule that regulates various physiological and pathophysiological processes in the human body. These processes include circulation and blood pressure, platelet function, host defense, and neurotransmission in the central nervous system and in peripheral nerves. It possesses cytotoxic properties that are aimed against pathogenic microbes, but it can also have damaging effects on host tissue [6].
Three different NOS isoforms have been characterized. The neuronal NOS (nNOS, NOS I) is expressed in the neurons of the brain and peripheral nervous system [7]. Endothelial NOS (eNOS, NOS III) is mainly expressed in endothelial cells [8,9]. Both nNOS and eNOS are constitutively expressed and are inactive in resting cells. The third isoform of the NOS family is the inducible NOS (iNOS, NOSII). No iNOS expression is found in most resting cells. Exposure to microbial products, such as lipopolysaccharide (LPS) and dsRNA or proinflammatory cytokines such as interleukin-1 (IL-1), tumor necrosis factor-α (TNF-α) and interferon-γ (IFN-γ) induces the expression of iNOS gene in various inflammatory and tissue cells [6]. These NOS isoforms also exist in the dental pulp tissue. nNOS is localized within the nerve fibers in the dental pulp, and NO produced by nNOS may act as a neurotransmitter within the pulp tissue [10]. eNOS is localized in endothelial cells and odontoblasts of the human dental pulp cells [11], indicating that NO synthesized by eNOS is responsible for regulation of the microcirculation within the pulp chamber and may control proliferation and differentiation of odontoblasts [12]. iNOS is expressed in the inflamed dental pulp cells and is mainly involved in inflammatory process and immune reaction [13-15]. The dental pulp cells abundantly express three NOS isoforms such as eNOS, nNOS and iNOS, which are known to play a pivotal role in physiologic and pathologic processes [11-13].
Apoptosis is an energy-dependent programmed cell death that is crucial to the balance between cellular survey and death. Previous studies have shown that mitochondrial dysfunction leads to an increase in expression of pro-apoptotic members of the B cell lymphoma 2 (Bcl-2) protein family as well as causes a loss of the mitochondrial membrane potential (MMP) and an increase in the release of cytochrome c from the mitochondrion to the cytoplasm. This process ultimately results in the activation of caspase-3, -6, and -7, as well as the activation of caspase-8, which cleaves Bid into tBid, which causes the release of apoptogenic proteins from the mitochondria and thus induces cell apoptosis [16-19].
In previous studies, NO has been shown to suppress apoptosis in endothelial cells [20], hepatocytes [21], eosinophils [22], and splenocytes [23], while inducing apoptosis in other cell types such as VSMCs [24], macrophages [25], neuronal cells [26], and pancreatic islet cells [27]. Large amounts of NO produced by NOS can induce apoptotic and necrotic cell death because NO can be cytotoxic at high concentrations in various cells including neuronal cells [28,29]. The molecular mechanism of the bifunctional action of NO remaines unclear and in controversy.
In general, NO acts as an intra- and intercellular messenger with various functions at the physiological level, whereas it can be cytotoxic at high concentrations, resulting in necrotic and apoptotic cell death [28,29]. Therefore, large amounts of NO synthesized by NOS can be cytotoxic to the dental pulp cells since previous studies have demonstrated that the inflamed pulp cells exhibit remarkably enhanced expression of iNOS which can produce large amounts of NO [14,15]. Nevertheless, NO-induced cytotoxicity in dental pulp cells and its underlying mechanism have not yet been elucidated. On the basis that the dental pulp cells abundantly express NOS, the present study aimed to investigate the mechanisms underlying NO-induced cell death of the HDPCs.
The study was approved by the Ethics Review Board of Chonnam National University. All the studies involving human participants were conducted in full compliance with government policies and the Declaration of Helsinki. All participants completed an informed consent.
HDPCs were obtained from the teeth of dental patients in Chonnam National Hospital. Teeth were immediately placed in phosphate-buffered saline (PBS) supplemented with antibiotics (100 µM/ml penicillin and 100 µg/ml streptomycin) and 0.25 µg/ml fungizone. The teeth were then transported to the laboratory on ice within 15 min of extraction. The teeth were sectioned horizontally at 1 mm below the cementoenamel junction (CEJ) using a #330 carbide bur mounted on a high-speed handpiece with an air-water spray and then they were split open. The pulp tissues were removed aseptically and minced with a blade into small fragments. They were then placed in a 6-well cell culture plate and incubated in DMEM (Gibco-BRL, USA) supplemented with 10% fetal bovine serum (FBS, Gibco-BRL, USA) and antibiotics. The cultures were maintained at 37℃ in a humidified atmosphere of 5% CO2. Cell cultures between the fifth and sixth passage were used in this study.
The effect of sodium nitroprusside (SNP, Sigma, USA) treatment on the cytotoxicity of HDPCs was determined by MTS assay. Breifly, cells were cultured overnight in 96-well plates (~1×104 cells/well). Cell viability was assessed after the addition of SNP at the indicated concentrations for 3, 6, 12 or 24 hrs in 48-well plates. The number of viable cells was assessed by determination of the A490 nm of the dissolved formazan product after addition of MTS for 1 hr at 37℃ as described by the manufacturer (Promega, USA).
Morphological changes in apoptotic cells were investigated by 4'6'-diamidino-2-phenylindole (DAPI) staining. Cells were plated in an 8-well chamber slide at a density of 1×104 cells/ml and incubated for 24 hrs, subsequently followed by treatment with 4 mM SNP for 5 hrs. The cells were then washed with 1×PBS and fixed with 4% paraformaldehyde. After incubation for 5 min at room temperature (RT), cells were stained with 10 µg/ml of DAPI (Sigma, USA) in PBS and observed under an LSM 510 confocal microscope (Zeiss, Germany).
Cells undergoing apoptotiis was determined by Annexin V-FITC Apoptosis Kit II (Pharmingen, USA). Cells were treated with SNP (1, 2, 3, 4 and 5 mM) and incubated for 24 hrs, harvested at a density of 106 cells/ml and resuspended in 1×binding buffer. In a 5 ml tube, Annexin V-FITC and PI were added and incubated for 15 min at RT in the dark. Then, 1×binding buffer was mixed to each tube and stained cells were measured by flowcytometry (Beckman, Germany).
Reactive oxygen species (ROS) production was monitored by a fluorescence spectrometer (Hitachi F-4500, Japan) using 2',7'-dichlorofluor-bescin diacetate (DCF-DA, Sigma, USA). Cells (5×104 cells/well) were plated in a 96-well plate for 24 hrs and incubated with 5 mM N-acetyl-cysteine (NAC, Sigma, USA) and SNP (1, 2, 3, 4 and 5 mM) treatment. After DCF-DA (25 µM) was treated and incubated for 15 min at 37℃, the fluorescence excitation was analyzed at 480 nm and the fluorescence emission was analyzed at 530 nm using a fluorescence spectrometer (Hitachi F-4500, Japan).
Caspase activities were determined by the caspase-3 and -9 activity assay kits (MBL, USA) according to the manufacturer's protocol. Briefly, HDPCs were grown on 100 mm dishes and treated with SNP for the indicated times (3, 6, 9, 12 and 24 hrs). The media were removed from the culture dishes and the cells were collected and washed with PBS, then resuspended in cell lysis buffer. After incubation on ice for 10 min, the lysates were centrifuged for 20 min at 11,000×g, and the supernatants were collected and protein concentrations were determined (BCA assay, Pierce). Fifty µl/ml of cell lysates were mixed with the reaction buffer containing the DEVD-pNA substrate (200 µM) for caspase-3 activity and the LEHD-pNA substrate (200 µM) for caspase-9 activity. After incubation for 24 hrs at 37℃, absorbance was measured in the wells at 405 nm by an ELISA reader.
Cells were washed twice with PBS and proteins were solubilized in the lysis buffer (500 mM Tris-HCl, pH 7.4, 150 mM NaCl, 5 mM EDTA, 1 mM Benzamide, 1 µg/ml Trypsin inhibitor) containing a protease inhibitor cocktail (Complete, Germany). Lysates were incubated for 30 min at 4℃, centrifuged at 11,000×g for 20 min at 4℃ and protein concentrations were determined by BCA protein assay (Pierce, IL). Protein extracts (50~100 µg) were boiled for 5 min in SDS-sample buffer and then subjected to electrophoresis on 12% polyacrylamide gel. Proteins were electroblotted onto a nitrocellulose membrane (Amersham Pharmacia Biotech, UK) and blocked with 5% skim milk (Becton Dickinson, USA) in tris-buffered saline-0.1%Tween 20 (TBS-T). Primary antibodies of rat monoclonal anti-cytochrome c (BD, USA), Bcl-2 (Santa Cruz, USA), Bax, cleaved caspase-3 (Cell signaling, USA) were used. After rinsing membrane three times in TBS-T and subsequently incubated with specific peroxidase-conjugated secondary antibodies (Sigma, USA) for 1 hr. Bound antibodies were visualized using an enhanced chemiluminescence detection system (Amersham Pharmacia Biotech, UK).
Each experiment was repeated at least three times unless stated otherwise. The data were expressed as means±S.D. The statistical significance of was performed using one-way and two-way analysis of variance (ANOVA) followed by a Student-Newman-Keuls test. Results were considered statistically significant when the p value was less than 0.05.
SNP, is widely used NO donor, that in vivo and in vitro releases NO [4]. To investigate the cytotoxic effect of SNP on HDPCs, cells were incubated with different concentrations of SNP (1, 2, 3, 4 and 5 mM) or 4 mM SNP for the indicated times (3, 6, 12 and 24 hrs). As shown in Fig. 1A, SNP was gradually attenuated cell viability of HDPCs in a dose- and time-dependent manner. The cell survival was less than 60% when the cells were treated with 4 mM SNP for 24 hrs. After exposure to 4 mM SNP for 5 hrs in HDPCs, cells showed typical apoptotic morphology including cell shrinkage, chromatin condensation and nuclear fragmentation (Fig. 1B). Annexin V is phosphatidylserine, which appears on the cell surface as a general indicator of apoptosis. SNP-treated HDPCs caused an increase in the number of Annexin V-positive cells in a dose-dependent manner (Fig. 1C). Together, these results strongly suggest that NO induces apoptosis in HDPCs.
To validate whether mitochondria are involved in NO-induced apoptotic cell death of HDPCs, cytosolic cytochrome c level was assessed as a consequence of cytochrome c released from the mitochondria into cytoplasm. Cytosolic cytochrome c level was dose-dependently increased in response to exposure to SNP in HDPCs (Fig. 2A). Because NO increased the release of cytochrome c into the cytosol from the mitochondria in HDPCs, we determined if NO alters the level of Bcl-2 family, which are important regulators of mitochondrial permeability. The expression level of Bax, a pro-apoptotic member of the Bcl-2 family, was upregulated, whereas the expression of Bcl-2, an anti-apoptotic member of the Bcl-2 family, was downregulated in response to SNP treatment in HDPCs. Also, Bax/Bcl-2 ratio was increased in SNP-treated HDPCs compared to control (Fig. 2). These results demonstrate that NO-induced apoptosis is likely mediated by mitochondrial pathway in HDPCs.
The release of cytochrome c from the mitochondria into the cytosol can activate caspases during apoptosis. SNP-treated HDPCs enhanced the activities of caspase-9, an initiator caspase, and caspase-3, an executioner caspase, in a time-dependent manner (Fig. 3A and B). In addition, to assess the role of caspases in NO-induced apoptosis, the specific inhibitor for caspase-3 (z-DEVD-fmk) and pan-caspase inhibitor (z-VAD-fmk) were pretreated for 30min before SNP treatment and MTS was performed. The results of the MTS assay showed that these caspase inhibitors significantly attenuated NO-induced cell death, indicating that these caspases play a crucial role in NO-induced apoptosis in HDPCs (Fig. 3C).
To determine the involvement of ROS in NO-induced cell death of the HDPCs, ROS production was measured using DCF-DA. SNP-treated HDPCs exhibited enhanced ROSproduction in a dose-dependent manner (Fig. 4A), whereas pretreatment of cells with 5 mM NAC, a ROS scavenger, alleviated the decrease in cell viability induced by SNP (Fig. 4B). These data suggest that ROS produced by NO plays as a crucial mediator in NO-induced apoptosis of HDPCs. To confirm the involvement of ROS in NO-induced apoptosis in HDPCs, cells were treated with 4 mM SNP alone or in the presence with 5 mM NAC. The expression levels of Bax, Bcl-2, cytochrome c and cleaved caspase-3 induced by SNP treatment were ameliorated by NAC (Fig. 4C).
In addition, NO can affect a variety of physiological processes, including programmed cell death (PCD) through cyclic guanosine monophosphate (cGMP)-dependent and -independent pathways. To examine whether NO acts through the cGMP pathway, effects of the soluble guanylate cyclase inhibitor on NO-induced cell death in HDPCs were examined. ODQ (100 µM), a guanylate cyclase inhibitor, did not rescue the cell viability decreased by SNP (Fig. 4D), indicating that NO-induced apoptosis was not mediated through the cGMP pathway.
Irreversible pulpitis has been associated with pain and an increase in the number of pulp inflammatory cells. Based on the action of NO elsewhere, NO may possibly participate in the sensory and autonomic innervation of the dental pulp, and may influence local inflammatory responses [14]. Indeed, the inflamed dental pulp cells exhibit high expression of iNOS which can result in mass production of NO [13-15]. However, the cellular mechanisms underlying NO-induced cell death in dental pulp cells was poorly understood.
In the present study, we investigated the effects of NO on growth in HDPCs. SNP, an NO donor, decreased the cell viability and caused apoptotic morphological changes including chromatin condensation, DNA fragmentation, and cell shrinkage in HDPCs. Besides, SNP treatment of HDPCs caused an increase in the number of Annexin V-positive cells in a dose-dependent manner. These results demonstrate that NO induces apoptosis in HDPCs.
Mitochondria-dependent apoptosis, which is upstream of caspase activation, is regulated by members of the Bcl-2 family. Bcl-2 family proteins regulate the release of apoptogenic cytochrome c by the mitochondrial channel VDAC [30,31]. The Bcl-2 subfamily contains anti-apoptotic proteins such as Bcl-2 and Bcl-XL, which reduce cytochrome c release and loss of mitochondrial transmembrane potential (Δψm), whereas the Bax subfamily contains pro-apoptotic proteins such as Bax and Bak, which induce cytochrome c release and loss of Δψm [32-34]. Therefore, the ratio of pro-apoptotic to anti-apoptotic protein may be pivotal for the release of cytochrome c from mitochondria into the cytosol. In the present study, expression of Bax was increased and level of Bcl-2 was decreased in HDPCs, indicating that increased ratio of Bax to Bcl-2 in NO-induced apoptosis of HDPCs mediates the transition of cytochrome c from the mitochondria into the cytosol. These results suggest that NO alter the expression of the Bcl-2 family in HDPCs, which affects the permeability of the mitochondrial membrane and the release of cytochrome c from the mitochondria to the cytosol. These findings definitely demonstrate that NO-induced apoptosis in HDPCs is mediated by the mitochondria-dependent pathway. The present findings along with those from previous studies suggest that the change in the Bax/Bcl-2 ratio is an important factor of NO-induced apoptosis. However, the exact mechanism of how NO induces a change in the Bax/Bcl-2 gene expression is unclear. Some investigators have suggested that p53 is a mediator between NO and Bax/Bcl-2 genes because NO can induces the accumulation of p53 [35,36], which activates a direct transcription of the Bax gene and inhibits a transcription of the Bcl-2 gene in macrophages [37,38]. However, this may not be true for HDPCs and this issue needs to be investigated in further study.
A variety of free radicals such as ROS and peroxynitrite are known to impair mitochondrial function, subsequently resulting in loss of mitochondrial transmembrane potential and release of mitochondrial pro-apoptotic molecules including cytochrome c, Smac, apoptosis-inducing factor (AIF) [39,40]. The present study showed that SNP treatment resulted in an increase in cytochrome c release from the mitochondria into the cytoplasm in a dose-dependent manner. The released the cytoplasmic cytochrome c was reported to be an upstream mediator of caspase-3 activation in different cell types [30,41,42]. In general, caspase-3 is one of the key, common proteases in the mitochondria-dependent pathway and it is particularly important in free radical-induced apoptosis [43-45]. The present study showed that caspase-9 and -3 activities were enhanced in SNP-treated HDPCs. Additionally, pretreatment with z-DEVD-fmk (caspase-3 inhibitor) and z-VAD-fmk (pan-caspases inhibitor) significantly inhibited NO-induced apoptosis, indicating that NO-induced apoptosis in HDPCs is mediated by caspase-dependent pathway. However, there are some previous reports that a caspase-independent pathway such as the MAP kinase pathway has also been proposed to be involved in NO-induced cell death in HL-60 cells and PC12 cells [46,47].
Previous studies have shown that NO causes apoptosis through the cyclic GMP pathway [48] or can induce the production of ROS such as H2O2 in mitochondria and its reaction with superoxide, resulting in formation of peroxynitrite in various other cells [46,49]. In the present study, SNP treatment increased the production of ROS, whereas NAC, a free radical scavenger, rescued the cell viability of HDPCs decreased by SNP. Besides, SNP-induced activation of proapoptotic mitocondria-dependent pathways (Cytochrome c, Bax, Bcl-2, and caspase-3) is down-regulated in the presence of NAC, a ROS scavenger. Meanwhile, ODQ did not rescue the cell viability of HDPCs decreased by SNP. From these results, it can be speculated that NO-induced apoptosis in HDPCs may be mediated in part by ROS, but not by cyclic GMP.
In conclusion, the present study shows that NO induces apoptosis in HDPCs through the mitochondria-dependent pathway mediated by ROS and Bcl-2 family, but not by the cyclic GMP pathway.
ACKNOWLEDGEMENTS
This research was supported by Basic Science Research Program through the National Research Foundation of Korea (NRF) funded by the Ministry of Education, Science and Technology (2011-0029663) and by Chonnam National University, 2010. In addition, this work was supported the National Research Foundation of Korea (NRF) grant funded by the Korea government (MEST) (2011-0030761) and by a grant (CNHRICM-2010) from Chonnam National Hospital Research Institute of Clinical Medicine.
References
1. Massey WL, Romberg DM, Hunter N, Hume WR. The association of carious dentin microflora with tissue changes in human pulpitis. Oral Microbiol Immunol. 1993; 8:30–35. PMID: 8510981.


2. Ohnishi T, Daikuhara Y. Hepatocyte growth factor/scatter factor in development, inflammation and carcinogenesis: its expression and role in oral tissues. Arch Oral Biol. 2003; 48:797–804. PMID: 14596869.


3. Carr AC, McCall MR, Frei B. Oxidation of LDL by myeloperoxidase and reactive nitrogen species: reaction pathways and antioxidant protection. Arterioscler Thromb Vasc Biol. 2000; 20:1716–1723. PMID: 10894808.
5. Förstermann U, Closs EI, Pollock JS, Nakane M, Schwarz P, Gath I, Kleinert H. Nitric oxide synthase isozymes. Characterization, purification, molecular cloning, and functions. Hypertension. 1994; 23:1121–1131. PMID: 7515853.


6. Korhonen R, Lahti A, Kankaanranta H, Moilanen E. Nitric oxide production and signaling in inflammation. Curr Drug Targets Inflamm Allergy. 2005; 4:471–479. PMID: 16101524.


7. Boissel JP, Schwarz PM, Förstermann U. Neuronal-type NO synthase: transcript diversity and expressional regulation. Nitric Oxide. 1998; 2:337–349. PMID: 10100489.


8. Shaul PW. Regulation of endothelial nitric oxide synthase: location, location, location. Annu Rev Physiol. 2002; 64:749–774. PMID: 11826287.


9. Shin W, Cuong TD, Lee JH, Min B, Jeon BH, Lim HK, Ryoo S. Arginase Inhibition by Ethylacetate Extract of Caesalpinia sappan Lignum Contributes to Activation of Endothelial Nitric Oxide Synthase. Korean J Physiol Pharmacol. 2011; 15:123–128. PMID: 21860589.
10. Lohinai Z, Székely AD, Benedek P, Csillag A. Nitric oxide synthase containing nerves in the cat and dog dental pulp and gingiva. Neurosci Lett. 1997; 227:91–94. PMID: 9180211.


11. Felaco M, Di Maio FD, De Fazio P, D'Arcangelo C, De Lutiis MA, Varvara G, Grilli A, Barbacane RC, Reale M, Conti P. Localization of the e-NOS enzyme in endothelial cells and odontoblasts of healthy human dental pulp. Life Sci. 2000; 68:297–306. PMID: 11191645.


12. Mei YF, Yamaza T, Atsuta I, Danjo A, Yamashita Y, Kido MA, Goto M, Akamine A, Tanaka T. Sequential expression of endothelial nitric oxide synthase, inducible nitric oxide synthase, and nitrotyrosine in odontoblasts and pulp cells during dentin repair after tooth preparation in rat molars. Cell Tissue Res. 2007; 328:117–127. PMID: 17216200.


13. Di Nardo Di Maio F, Lohinai Z, D'Arcangelo C, De Fazio PE, Speranza L, De Lutiis MA, Patruno A, Grilli A, Felaco M. Nitric oxide synthase in healthy and inflamed human dental pulp. J Dent Res. 2004; 83:312–316. PMID: 15044505.


14. da Silva LP, Issa JP, Del Bel EA. Action of nitric oxide on healthy and inflamed human dental pulp tissue. Micron. 2008; 39:797–801. PMID: 18337111.
15. Kawanishi HN, Kawashima N, Suzuki N, Suda H, Takagi M. Effects of an inducible nitric oxide synthase inhibitor on experimentally induced rat pulpitis. Eur J Oral Sci. 2004; 112:332–337. PMID: 15279652.


16. Kischkel FC, Hellbardt S, Behrmann I, Germer M, Pawlita M, Krammer PH, Peter ME. Cytotoxicity-dependent APO-1 (Fas/CD95)-associated proteins form a death-inducing signaling complex (DISC) with the receptor. EMBO J. 1995; 14:5579–5588. PMID: 8521815.


17. Li H, Zhu H, Xu CJ, Yuan J. Cleavage of BID by caspase 8 mediates the mitochondrial damage in the Fas pathway of apoptosis. Cell. 1998; 94:491–501. PMID: 9727492.


18. Riedl SJ, Salvesen GS. The apoptosome: signalling platform of cell death. Nat Rev Mol Cell Biol. 2007; 8:405–413. PMID: 17377525.


19. Boulares AH, Zoltoski AJ, Sherif ZA, Yakovlev A, Smulson ME. Roles of DNA fragmentation factor and poly(ADP-ribose) polymerase-1 in sensitization of fibroblasts to tumor necrosis factor-induced apoptosis. Biochem Biophys Res Commun. 2002; 290:796–801. PMID: 11785971.


20. Kim YM, Bombeck CA, Billiar TR. Nitric oxide as a bifunctional regulator of apoptosis. Circ Res. 1999; 84:253–256. PMID: 10024298.


21. Dimmeler S, Haendeler J, Nehls M, Zeiher AM. Suppression of apoptosis by nitric oxide via inhibition of interleukin-1beta-converting enzyme (ICE)-like and cysteine protease protein (CPP)-32-like proteases. J Exp Med. 1997; 185:601–607. PMID: 9034139.
22. Kim YM, de Vera ME, Watkins SC, Billiar TR. Nitric oxide protects cultured rat hepatocytes from tumor necrosis factor-alpha-induced apoptosis by inducing heat shock protein 70 expression. J Biol Chem. 1997; 272:1402–1411. PMID: 8995451.
23. Hebestreit H, Dibbert B, Balatti I, Braun D, Schapowal A, Blaser K, Simon HU. Disruption of fas receptor signaling by nitric oxide in eosinophils. J Exp Med. 1998; 187:415–425. PMID: 9449721.


24. Genaro AM, Hortelano S, Alvarez A, Martínez C, Boscá L. Splenic B lymphocyte programmed cell death is prevented by nitric oxide release through mechanisms involving sustained Bcl-2 levels. J Clin Invest. 1995; 95:1884–1890. PMID: 7706495.


25. Lincoln TM, Cornwell TL, Komalavilas P, Boerth N. Cyclic GMP-dependent protein kinase in nitric oxide signaling. Methods Enzymol. 1996; 269:149–166. PMID: 8791645.
26. Albina JE, Cui S, Mateo RB, Reichner JS. Nitric oxide-mediated apoptosis in murine peritoneal macrophages. J Immunol. 1993; 150:5080–5085. PMID: 7684418.
27. Heneka MT, Löschmann PA, Gleichmann M, Weller M, Schulz JB, Wüllner U, Klockgether T. Induction of nitric oxide synthase and nitric oxide-mediated apoptosis in neuronal PC12 cells after stimulation with tumor necrosis factor-alpha/lipopolysaccharide. J Neurochem. 1998; 71:88–94. PMID: 9648854.
28. Gross SS, Wolin MS. Nitric oxide: pathophysiological mechanisms. Annu Rev Physiol. 1995; 57:737–769. PMID: 7539995.


29. Dawson VL, Dawson TM. Nitric oxide neurotoxicity. J Chem Neuroanat. 1996; 10:179–190. PMID: 8811421.


30. Liu X, Kim CN, Yang J, Jemmerson R, Wang X. Induction of apoptotic program in cell-free extracts: requirement for dATP and cytochrome c. Cell. 1996; 86:147–157. PMID: 8689682.


31. Jun CD, Pae HO, Kwak HJ, Yoo JC, Choi BM, Oh CD, Chun JS, Paik SG, Park YH, Chung HT. Modulation of nitric oxide-induced apoptotic death of HL-60 cells by protein kinase C and protein kinase A through mitogen-activated protein kinases and CPP32-like protease pathways. Cell Immunol. 1999; 194:36–46. PMID: 10357879.


32. Kluck RM, Bossy-Wetzel E, Green DR, Newmeyer DD. The release of cytochrome c from mitochondria: a primary site for Bcl-2 regulation of apoptosis. Science. 1997; 275:1132–1136. PMID: 9027315.


33. Gottlieb E, Vander Heiden MG, Thompson CB. Bcl-x(L) prevents the initial decrease in mitochondrial membrane potential and subsequent reactive oxygen species production during tumor necrosis factor alpha-induced apoptosis. Mol Cell Biol. 2000; 20:5680–5689. PMID: 10891504.
34. Howard S, Bottino C, Brooke S, Cheng E, Giffard RG, Sapolsky R. Neuroprotective effects of bcl-2 overexpression in hippocampal cultures: interactions with pathways of oxidative damage. J Neurochem. 2002; 83:914–923. PMID: 12421364.


35. Hemish J, Nakaya N, Mittal V, Enikolopov G. Nitric oxide activates diverse signaling pathways to regulate gene expression. J Biol Chem. 2003; 278:42321–42329. PMID: 12907672.


36. Brüne B, von Knethen A, Sandau KB. Nitric oxide and its role in apoptosis. Eur J Pharmacol. 1998; 351:261–272. PMID: 9721017.


37. López-Farré A, Rodríguez-Feo JA, Sánchez de Miguel L, Rico L, Casado S. Role of nitric oxide in the control of apoptosis in the microvasculature. Int J Biochem Cell Biol. 1998; 30:1095–1106. PMID: 9785475.


38. Miyashita T, Reed JC. Tumor suppressor p53 is a direct transcriptional activator of the human bax gene. Cell. 1995; 80:293–299. PMID: 7834749.


39. Brown GC. Nitric oxide and mitochondrial respiration. Biochim Biophys Acta. 1999; 1411:351–369. PMID: 10320668.


40. Fleury C, Mignotte B, Vayssière JL. Mitochondrial reactive oxygen species in cell death signaling. Biochimie. 2002; 84:131–141. PMID: 12022944.


41. Herrera B, Alvarez AM, Sánchez A, Fernández M, Roncero C, Benito M, Fabregat I. Reactive oxygen species (ROS) mediates the mitochondrial-dependent apoptosis induced by transforming growth factor (beta) in fetal hepatocytes. FASEB J. 2001; 15:741–751. PMID: 11259392.
42. Haunstetter A, Izumo S. Apoptosis: basic mechanisms and implications for cardiovascular disease. Circ Res. 1998; 82:1111–1129. PMID: 9633912.
44. Earnshaw WC, Martins LM, Kaufmann SH. Mammalian caspases: structure, activation, substrates, and functions during apoptosis. Annu Rev Biochem. 1999; 68:383–424. PMID: 10872455.


45. Bal-Price A, Brown GC. Nitric-oxide-induced necrosis and apoptosis in PC12 cells mediated by mitochondria. J Neurochem. 2000; 75:1455–1464. PMID: 10987825.


46. Taimor G, Hofstaetter B, Piper HM. Apoptosis induction by nitric oxide in adult cardiomyocytes via cGMP-signaling and its impairment after simulated ischemia. Cardiovasc Res. 2000; 45:588–594. PMID: 10728380.


47. Leist M, Single B, Naumann H, Fava E, Simon B, Kühnle S, Nicotera P. Nitric oxide inhibits execution of apoptosis at two distinct ATP-dependent steps upstream and downstream of mitochondrial cytochrome c release. Biochem Biophys Res Commun. 1999; 258:215–221. PMID: 10222263.
48. Murphy MP. Nitric oxide and cell death. Biochim Biophys Acta. 1999; 1411:401–414. PMID: 10320672.


49. Yuyama K, Yamamoto H, Nishizaki I, Kato T, Sora I, Yamamoto T. Caspase-independent cell death by low concentrations of nitric oxide in PC12 cells: involvement of cytochrome C oxidase inhibition and the production of reactive oxygen species in mitochondria. J Neurosci Res. 2003; 73:351–363. PMID: 12868069.


Fig. 1
NO-induced apoptosis in HDPCs. Cells were incubated with various concentrations (1, 2, 3, 4 and 5 mM) of SNP and 4 mM SNP for the indicated times. Cell viability was determined by the MTS assay as described in the 'Materials and Methods' section. Viability of the cells without SNP treatment was 100% (A). Cells were treated with 4 mM SNP for 5 hrs and fixed with 4% paraformaldehyde and cells were stained using DAPI. Apoptotic nuclei stained with DAPI show fluorescence as a result of chromatin condensation and fragmentation (arrow) (B). Cells treated with different concentrations of SNP for 24 hrs were stained with FITC-conjugated Annexin V in a buffer containing propidium iodide (PI), followed by flow cytometric analysis (C). Kinetic measurement of cells underwent apoptosis in HDPCs (D). Data are expressed as mean±S.D. from triplicate independent experiments. **p<0.01, vs. control.
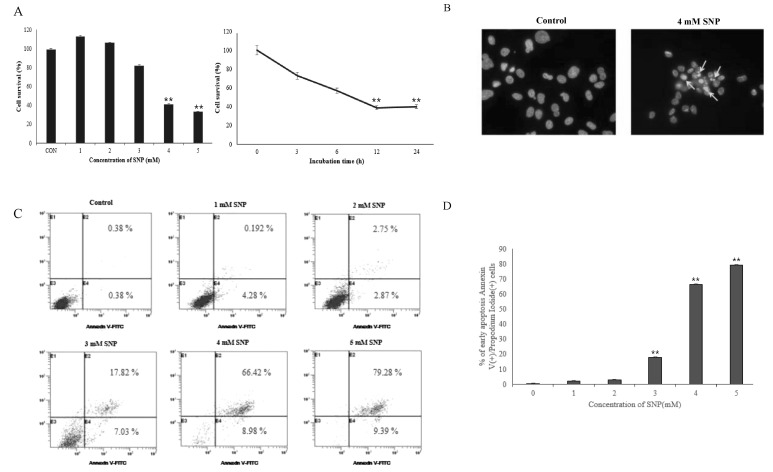
Fig. 2
Release of cytochrome c and expression of Bcl-2 family in SNP-treated HDPCs. After incubation of HDPCs with SNP for 24 hrs, levels of cytochrome c in cytosolic fraction, Bax and Bcl-2 were analyzed by Western blot (A). The ratio of Bax and Bcl-2 expression level was determined using a densitometer (B). Data are expressed as mean±S.D. from triplicate independent experiments. **p<0.01, vs. control.
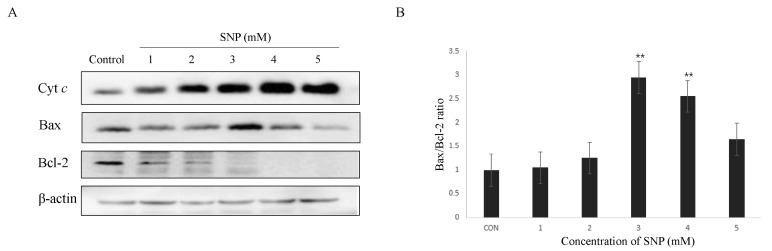
Fig. 3
Involvement of caspase-3,-9 in NO-induced apoptosis of HDPCs. Activation of caspases were assessed by absorbance for caspase-9 activity was measured at 405 nm by an ELISA reader after incubation with LEHD-pNA substrate (200 µM) for 4 hrs at 37℃ (A). Absorbance for caspase-3 activity was measured in the wells at 405 nm by an ELISA reader after incubation with DEVD-pNA substrate (200 µM) for 24 hrs at 37℃ (B). Cells were incubated for 24 hrs with 4 mM SNP in presence or absence of z-VAD-fmk (50 µM) or z-DEVD-fmk (50 µM) and then MST assay was performed (C). The data are represented as a mean±S.D. from triplicate independent experiments. **p<0.01 vs. control; ##p<0.01 vs. SNP-treated group.
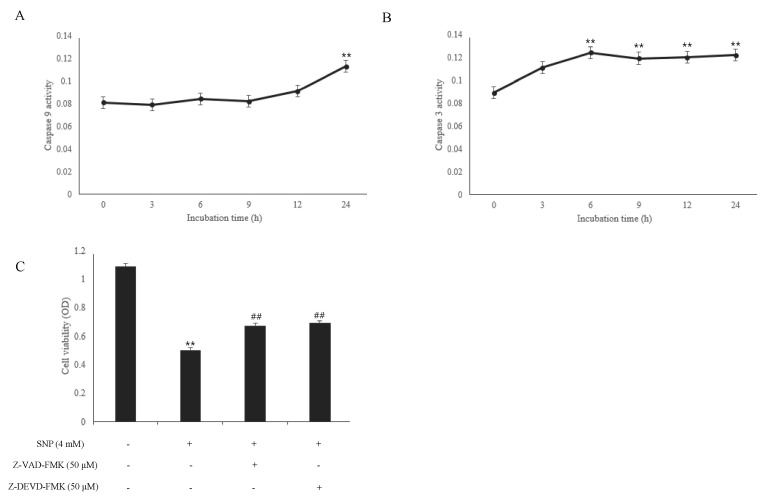
Fig. 4
Effects of NO on the ROS generation in HDPCs. DCF-loaded cells were incubated with various concentrations (1, 2, 3, 4 and 5 mM) of SNP for 1 hr and the intracellular levels of ROS were detected by measuring the DCF-DA fluorescence (A). Cells were treated with 4 mM SNP alone or co-incubated with 5 mM N-acetyl-L-cysteine (NAC) for 24 hrs and cell viability was analyzed by MTS assay. NAC, a free radical scavenger, ameliorated the decrease of cell viability induced by SNP. Data are expressed as mean±S.D. from triplicate independent experiments. **p<0.01, vs. control; ##p<0.01 vs. SNP-treated group (B). Cells were treated with 4 mM SNP alone or co-incubated with 5 mM NAC for 24 hrs and Bax, Bcl-2, Cytochrome c (Cyt c) and cleaved caspase-3 were detected by Western blot (C). ODQ, a soluble guanylate cyclase inhibitor, did not rescue the cell viability decreased by SNP. Results are expressed as mean±SD from triplicate independent experiments. Data are expressed as mean±S.D. from triplicate independent experiments. **p<0.01, vs. control (D).
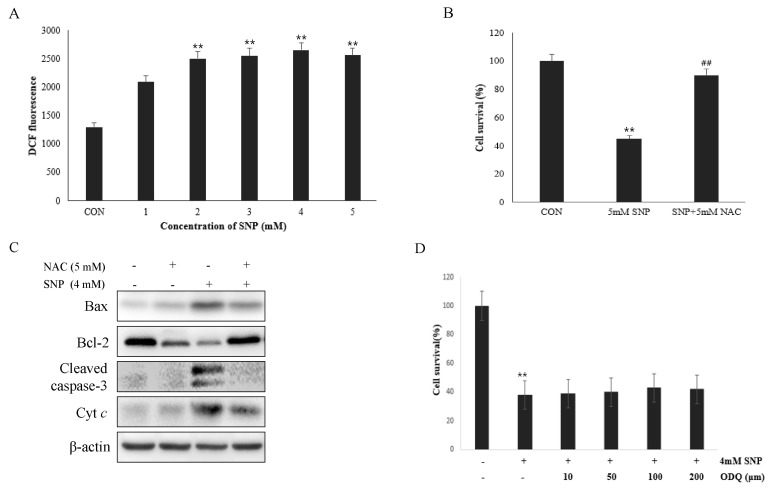