Abstract
To investigate the underlying mechanisms of C18 fatty acids (stearic acid, oleic acid, linoleic acid and α-linolenic acid) on mast cells, we measured the effect of C18 fatty acids on intracellular Ca2+ mobilization and histamine release in RBL-2H3 mast cells. Stearic acid rapidly increased initial peak of intracellular Ca2+ mobilization, whereas linoleic acid and α-linolenic acid gradually increased this mobilization. In the absence of extracellular Ca2+, stearic acid (100 µM) did not cause any increase of intracellular Ca2+ mobilization. Both linoleic acid and α-linolenic acid increased intracellular Ca2+ mobilization, but the increase was smaller than that in the presence of extracellular Ca2+. These results suggest that C18 fatty acid-induced intracellular Ca2+ mobilization is mainly dependent on extracellular Ca2+ influx. Verapamil dose-dependently inhibited stearic acid-induced intracellular Ca2+ mobilization, but did not affect both linoleic acid and α-linolenic acid-induced intracellular Ca2+ mobilization. These data suggest that the underlying mechanism of stearic acid, linoleic acid and α-linolenic acid on intracellular Ca2+ mobilization may differ. Linoleic acid and α-linolenic acid significantly increased histamine release. Linoleic acid (C18:2: ω-6)-induced intracellular Ca2+ mobilization and histamine release were more prominent than α-linolenic acid (C18:3: ω-3). These data support the view that the intake of more α-linolenic acid than linoleic acid is useful in preventing inflammation.
Mast cells play a central role in the innate immune response. They are particularly important in helping to recruit other cells, such as basophils, neutrophils and lymphocytes. Mast cells play an important role in a number of allergic diseases including atopic dermatitis and asthma, and contribute to chronic inflammatory conditions like atherosclerosis, vasculitis and rheumatoid arthritis. Mast cells have a number of secretory granules including histamine and 5-hydroxytryptamine, and rapidly synthesize lipid-derived mediators, such as prostaglandins and leukotrienes from arachidonic acid (C20:4: ω-6) released by the activation of phospholipase A2 [1]. The addition of arachidonic acid to RBL-2H3 cells distinctly alter the fatty acid composition of the cell membrane and enhance Fcε RI-mediated degranulation and tumor necrosis factor-alpha (TNF-α) release with augmented tyrosine phosphorylation of some proteins and Ca2+ influx [2]. We previously reported that exogenous arachidonic acid significantly increases histamine release from RBL-2H3 cells [3].
On the other hand, ω-3 polyunsaturated fatty acids (PUFAs) reportedly decrease the production of arachidonic acid-derived eicosanoids. These fatty acids decrease the production of inflammatory cytokines, such as TNF, interleukin (IL)-1, and IL-6, and the expression of adhesion molecules involved in inflammatory interactions between leukocytes and endothelial cells [4]. Babcock et al. reported that ω-3 PUFAs, such as eicosapentaenoic acid and docosahexaenoic acid, significantly inhibit the production of TNF-α and IL-10 in monocytes [5]. The anti-inflammation effects of ω-3 eicosapentaenoic acid and the inflammation reactions of ω-6 arachidonic acid have been studied largely, but α-linolenic acid (a precursor of eicosapentaenoic acid) and linoleic acid (a precursor of arachidonic acid) had been not. In this study, to investigate the underlying mechanisms of C18 fatty acids on anti-inflammatory response, we measured the effect of C18 fatty acids such as stearic acid (C18, saturated fatty acid), oleic acid (C18:1: ω-9), linoleic acid (C18:2: ω-6) and α-linolenic acid (C18:3: ω-3) on intracellular Ca2+ mobilization and histamine release in RBL-2H3 mast cells.
C18 fatty acids (stearic acid, oleic acid, linoleic acid and α-linolenic acid), verapamil, 8-(diethylamino)octyl-3,4,5-trimethoxybenzoate hydrochloride (TMB-8), bisindolylmaleimide (BIM), 3-(4,5-dimethyl-2-thiazolyl)-2,5-diphenyl-2H-tetrazolium bromide (MTT) and o-phthaldialdehyde (OPT) were obtained from Sigma Chemical (St. Louis, MO, USA). Fura-2/AM was purchased from Enzo Life Science (Farmingdale, NY, USA). [3H] Inositol was obtained from PerkinElmer (Waltham, MA, USA). AG 1-X8 resin was purchased from BIO-RAD (Hercules, CA, USA). The materials for cell culture were purchased from Life Technologies (Grand Island, NY, USA). Unless otherwise stated, all reagents were of the highest purity and were purchased from Sigma Chemical.
Rat basophilic leukemia (RBL)-2H3 cells were obtained from the American Type Culture Collection (ATCC, Manassas, VA, USA) and cultured as recommended by ATCC at 37℃ in Dulbecco's modified Eagle's medium (DMEM) supplemented with 10% heat-inactivated fetal bovine serum (FBS) and antibiotics (100 U/mL penicillin G, 100 µg/mL streptomycin) in a 5% CO2 incubator. Cells between 5 and 20 passages were used for experiments at a density of 1×106 cells/mL.
Cell viability testing was performed using a MTT-based colorimetric assay [6]. Cells were diluted and cultured in 96-well plates (1×104 cells/mL), with the select C18 fatty acid added (100~200 µM). An equal amount of ethanol was added in the control group, which resulted in a final concentration of 0.1% in the culture medium. MTT was added to the medium to a final concentration of 200 µg/mL and incubated for another 4 h at 37℃. After removing the culture medium, 200 µL of dimethylsulfoxide was added to the cells to dissolve the formazan. The absorbance of each well was measured by using a FlexStation device (Molecular Devices, Sunnyvale, CA, USA) at wavelength of 520 nm [7].
The intracellular Ca2+ level was measured using Fura-2/AM by monitoring a fluorospectrometer [8]. Briefly, culture medium was replaced and cells were washed three times with phosphate buffered saline (PBS). Cells were detached using 0.5% trypsin/EDTA buffer and suspended in 10 mL Krebs buffer [120.8 mM NaCl, 4.5 mM KCl, 1.2 mM MgCl2, 1.8 mM CaCl2, 5.6 mM glucose, 1.2 mM NaH2PO4 and 25 mM HEPES (pH 7.4)]. Fura-2/AM was added to a final concentration of 2 µM and incubated at 37℃ for 1 h. The cells were washed twice with Krebs buffer and centrifuged at 3,000×g for 10 min. For the experiments performed in the absence of external calcium, Krebs-EGTA buffer [120.8 mM NaCl, 4.5 mM KCl, 1.2 mM MgCl2, 1.0 mM EGTA, 5.6 mM glucose, 1.2 mM NaH2PO4 and 25 mM HEPES (pH 7.4)] was used. Fura-2 fluorescence was monitored by a Quanta Master device (Photon Technology International, Birmingham, NJ, USA.) at 37℃ with excitation at 340 and 380 nm and emission at 500 nm. The ratio of F340/F380 was recorded, and the maximum fluorescence ratio (Rmax) was measured using 0.1% Triton X-100. The minimum fluorescence ratio (Rmin) was measured following depletion of external Ca2+ by adding 5 mM EGTA/Tris, pH 8.5 [9,10].
The cells were labeled with [myo-3H]inositol (1 µCi/106 cells) for 24 h at 37℃ and rinsed twice with inositol-free medium containing 0.5% FBS, 20 mM of LiCl and bovine serum albumin (1 mg/mL) and then resuspended at a density of 2×107 cells/mL. A portion (1 mL) of the cell suspension was transferred to a microcentrifuge tube and incubated at 37℃ for 15 min. Phosphatidylinositol 4,5-bisphosphate (PIP2) hydrolysis was initiated by adding 100 µM fatty acids for 30 min. Reactions were terminated by adding 200 µL of ice-cold 10% perchloric acid (HClO4). After 30 min in an ice bath, the tubes were centrifuged and the supernatants were diluted 5-fold with distilled water and applied to Dowex AG 1-X8 anion exchange columns. Each column was washed with 2 mL of distilled water followed by 10 mL of 60 mM ammonium formate containing 5 mM sodium tetraborate. Total inositol phosphates were eluted with a solution containing 1 M ammonium formate and 0.1 M formic acid. The radioactivity of the [3H]inositol phosphates was determined using a Tri-Carb Liquid Scintillation Counter (PerkinElmer, Waltham, MA, USA) [11].
The method that the determination of histamine is based on the reaction of histamine with OPT, which results in a highly fluorescent condensation product [12]. The harvested RBL-2H3 cells were washed with Krebs buffer and suspended in Krebs buffer at a density of 106 cells/mL. The cells were treated with BIM for 10 min and histamine release was induced by C18 fatty acids for 30 min in 37℃. After centrifugation, histamine contents in both supernatant and pellet were measured with 0.1 mL of 1% OPT in methanol. After 4 min, the reaction was terminated by adding 0.2 mL of 3 N HCl. The fluorescence intensity was measured using excitation and emission wavelengths of 355 and 455 nm, respectively, with a FlexStation apparatus. Data are expressed as % release (histamine contents in supernatant / histamine contents in supernatant and pellet ×100).
To confirm cytotoxicity of C18 fatty acids, cell viability was determined by the ability of the cells to metabolically reduce MTT to a formazan dye. It was performed after 6, 12 and 24 h exposure to the C18 fatty acid stearic acid, oleic acid, linoleic acid or α-linolenic acid. After 24 h exposure to oleic acid, linoleic acid and α-linolenic acid, which contain a double bond, no cytotoxicity was evident at a concentration of 200 µM. In contrast, stearic acid, which lacks a double bond, reduced the cell viability by 16% at 200 µM (Fig. 1). However, stearic acid showed no cytotoxicity at 6 h.
To investigate the effects of C18 fatty acids on intracellular Ca2+ concentration, we measured Ca2+ mobilization in Fura-2/AM-loaded RBL-2H3 cells. As shown in Fig. 2A, stearic acid rapidly increased the initial peak of intracellular Ca2+ mobilization, whereas linoleic acid and α-linolenic acid gradually increased intracellular Ca2+ mobilization. Oleic acid (100 µM) slightly increased intracellular Ca2+ concentration, which was smaller than the effects of other C18 fatty acids (Fig. 2A). In the next experiment, we excluded oleic acid. Stearic acid, linoleic acid and α-linolenic acid dose-dependently increased intracellular Ca2+ mobilization (Figs. 2B-2D). In the absence of extracellular Ca2+, stearic acid (100 µM) did not cause any increase of intracellular Ca2+ mobilization, but both linoleic acid and α-linolenic acid increased intracellular Ca2+ mobilization; the increase was smaller than that in the presence of extracellular Ca2+ (Fig. 3). These data suggest that C18 fatty acid-induced intracellular Ca2+ mobilization is mainly dependent on extracellular calcium influx.
Verapamil (10 µM), a voltage-dependent calcium channel blocker, dose-dependently inhibited stearic acid-induced intracellular Ca2+ mobilization (Figs. 4A and 5), but did not affect linoleic acid- and α-linolenic acid-induced intracellular Ca2+ mobilization (Figs. 4B and 4C). At 10 µM TMB-8, a intracellular calcium release blocker, did not influence intracellular Ca2+ mobilization induced by stearic acid, linoleic acid and α-linolenic acid (data not shown). At 10 µM, BIM, a protein kinase C (PKC) inhibitor, significantly inhibited both linoleic acid- and α-linolenic acid-induced intracellular Ca2+ mobilization, but did not affect stearic acid-induced intracellular Ca2+ mobilization (Fig. 6).
The phospholipase C (PLC) pathway is closely involved in the intracellular Ca2+ mobilization. In [3H]inositol-labeled cells, melittin as a positive control significantly increased [3H]inositol phosphate formation by 245%. However, three C18 fatty acids did not increase [3H]inositol phosphate formation (Table 1), indicating that C18 fatty acids-induced intracellular Ca2+ mobilization is not associated with intracellular Ca2+ release by the PLC pathway.
At 100 µM, linoleic acid and α-linolenic acid significantly increased histamine release from 15.2±4.8% (control) to 54.3±7.7% and 44.2±8.8%, respectively, which was smaller than the effect of 100 µM arachidonic acid (69.7±2.4%). However, stearic acid, which lacks a double bond, did not induce histamine release at a concentration of 100 µM (Fig. 7A). At 10 µM, the BIM, PKC inhibitor, significantly inhibited linoleic acid- and α-linolenic acid-induced histamine release (Fig. 7B), suggesting the involvement of PKC in linoleic acid- and α-linolenic acid-induced histamine release in RBL-2H3 cells.
In the measurement of histamine release, it is important to confirm the cytotoxicity of C18 fatty acids. To measure histamine release from RBL-2H3 cells, C18 fatty acids were exposed to the cells for 1 h. This exposure was not toxic, since no cytotoxicity was apparent after 6 h exposure of the tested fatty acids. These data suggest that exocytosis of histamine may be independent on cell membrane rupture. It has been reported that exposure of stearic acid at 150 µM for 24 h induces cell death and that ω-6 PUFAs have a greater protective effect on the deleterious effect caused by stearic acid in ECV-304 endothelial cells than ω-3 PUFAs [13].
Stearic acid rapidly increased the initial peak of intracellular Ca2+ mobilization, whereas linoleic acid and α-linolenic acid gradually increased intracellular Ca2+ mobilization. In the absence of extracellular Ca2+, stearic acid (100 µM) did not cause any increase of intracellular Ca2+ mobilization. Both linoleic acid and α-linolenic acid increased intracellular Ca2+ mobilization, but the increase was smaller than that in the presence of extracellular Ca2+. These results suggest that C18 fatty acid-induced intracellular Ca2+ mobilization is mainly dependent on extracellular calcium influx. A prior study reported that 40 µM of three ω-6 polyunsaturated fatty acids (arachidonic acid, γ-linolenic acid and linoleic acid) increased intracellular calcium concentration, while 40 µM ω-3 and ω-9 PUFAs did not [14]. The discrepancy between the present and previous study may be due to the concentration of C18 fatty acids because we used 100 µM of the C18 fatty acids.
At 10 µM, verapamil, a voltage-dependent calcium channel blocker [15], inhibited stearic acid-induced intracellular Ca2+ mobilization, but did not affect both linoleic acid and α-linolenic acid-induced intracellular Ca2+ mobilization. The PKC inhibitor BIM used at 10 µM significantly inhibited both linoleic acid and α-linolenic acid-induced intracellular Ca2+ mobilization, but it did not affect stearic acid-induced intracellular Ca2+ mobilization. These data suggest that the underlying mechanism of stearic acid, linoleic acid and α-linolenic acid on intracellular Ca2+ mobilization may be different from each other. Also, C18 fatty acids-induced intracellular Ca2+ mobilization appeared not to be associated with intracellular Ca2+ release by the PLC pathway, since they failed to induce [3H]inositol phosphates formation in [3H]inositol-labeled cells. This finding is consistent with a previous report that 40 µM arachidonic acid did not result in the formation of inositol-1,4,5-triphosphates [14]. Long chain fatty acids and ω-3 PUFAs (EPA and DHA) have been demonstrated to act as ligands of several G-protein-coupled receptors, such as GRP40 and GRP120, respectively [16,17]. Especially GRP40 activation which is strongly expressed in the pancreas results in an increase of intracellular Ca2+ mobilization via the phosphoinositide pathway. Further study will be needed to elucidate novel physiological roles of GRP40 and GRP120 in RBL-2H3 cells.
Clinical studies [18,19,20] indicated that the ingested ratio of ω-6 to ω-3 (especially linoleic vs. α-linolenic) fatty acids is important for maintaining cardiovascular health. While ω-3 PUFAs are extremely beneficial in preventing heart disease in humans, the levels of ω-6 PUFAs are insignificant [21,22]. Both ω-3 α-linolenic acid and ω-6 linoleic acid are essential fatty acids in humans. ω-3 and ω-6 C18 PUFAs compete for the same metabolic enzymes; thus, the ω-6: ω-3 ratio will significantly influence the ratio of the ensuing eicosanoids (hormones) including prostaglandins, leukotrienes and thromboxanes, and will alter the body's metabolic function [23]. Especially, linoleic acid (C18:2: ω-6)-induced intracellular Ca2+ mobilization and histamine release are more prominent than α-linolenic acid (C18:3: ω-3). These data support the view that increased intake of α-linolenic acid compared to linoleic acid is useful in preventing inflammation.
References
2. Nakano N, Nakao A, Uchida T, Shirasaka N, Yoshizumi H, Okumura K, Tsuboi R, Ogawa H. Effects of arachidonic acid analogs on FcepsilonRI-mediated activation of mast cells. Biochim Biophys Acta. 2005; 1738:19–28. PMID: 16403671.
3. Lee JH, Lee JY, Kang HS, Jeong CH, Moon H, Whang WK, Kim CJ, Sim SS. The effect of acteoside on histamine release and arachidonic acid release in RBL-2H3 mast cells. Arch Pharm Res. 2006; 29:508–513. PMID: 16833020.


4. Calder PC. Polyunsaturated fatty acids and inflammation. Prostaglandins Leukot Essent Fatty Acids. 2006; 75:197–202. PMID: 16828270.


5. Babcock TA, Novak T, Ong E, Jho DH, Helton WS, Espat NJ. Modulation of lipopolysaccharide-stimulated macrophage tumor necrosis factor-alpha production by omega-3 fatty acid is associated with differential cyclooxygenase-2 protein expression and is independent of interleukin-10. J Surg Res. 2002; 107:135–139. PMID: 12384076.
6. Woerdenbag HJ, Merfort I, Passreiter CM, Schmidt TJ, Willuhn G, van Uden W, Pras N, Kampinga HH, Konings AW. Cytotoxicity of flavonoids and sesquiterpene lactones from Arnica species against the GLC4 and the COLO 320 cell lines. Planta Med. 1994; 60:434–437. PMID: 7997472.
7. Lee MJ, Song HJ, Jeong JY, Park SY, Sohn UD. Anti-Oxidative and Anti-Inflammatory Effects of QGC in Cultured Feline Esophageal Epithelial Cells. Korean J Physiol Pharmacol. 2013; 17:81–87. PMID: 23440684.


8. Tseng PH, Lin HP, Hu H, Wang C, Zhu MX, Chen CS. The canonical transient receptor potential 6 channel as a putative phosphatidylinositol 3,4,5-trisphosphate-sensitive calcium entry system. Biochemistry. 2004; 43:11701–11708. PMID: 15362854.


9. Grynkiewicz G, Poenie M, Tsien RY. A new generation of Ca2+ indicators with greatly improved fluorescence properties. J Biol Chem. 1985; 260:3440–3450. PMID: 3838314.
10. Hwang YH, Song HS, Kim HR, Ko MS, Jeong JM, Kim YH, Ryu JS, Sohn UD, Gimm YM, Myung SH, Sim SS. Intracellular Ca Mobilization and Beta-hexosaminidase Release Are Not Influenced by 60 Hz-electromagnetic Fields (EMF) in RBL 2H3 Cells. Korean J Physiol Pharmacol. 2011; 15:313–317. PMID: 22128265.
11. Bae YS, Lee TG, Park JC, Hur JH, Kim Y, Heo K, Kwak JY, Suh PG, Ryu SH. Identification of a compound that directly stimulates phospholipase C activity. Mol Pharmacol. 2003; 63:1043–1050. PMID: 12695532.


12. Håkanson R, Rönnberg AL, Sjölund K. Fluorometric determination of histamine with OPT: optimum reaction conditions and tests of identity. Anal Biochem. 1972; 47:356–370. PMID: 5036454.


13. Masi LN, Portioli-Sanches EP, Lima-Salgado TM, Curi R. Toxicity of fatty acids on ECV-304 endothelial cells. Toxicol In Vitro. 2011; 25:2140–2146. PMID: 21723937.


14. Teshima R, Amano F, Nakamura R, Tanaka Y, Sawada J. Effects of polyunsaturated fatty acids on calcium response and degranulation from RBL-2H3 cells. Int Immunopharmacol. 2007; 7:205–210. PMID: 17178388.


15. Baliño P, Pastor R, Aragon CM. Participation of L-type calcium channels in ethanol-induced behavioral stimulation and motor incoordination: effects of diltiazem and verapamil. Behav Brain Res. 2010; 209:196–204. PMID: 20122967.


16. Ichimura A, Hirasawa A, Hara T, Tsujimoto G. Free fatty acid receptors act as nutrient sensors to regulate energy homeostasis. Prostaglandins Other Lipid Mediat. 2009; 89:82–88. PMID: 19460454.


17. Oh DY, Talukdar S, Bae EJ, Imamura T, Morinaga H, Fan W, Li P, Lu WJ, Watkins SM, Olefsky JM. GPR120 is an omega-3 fatty acid receptor mediating potent anti-inflammatory and insulin-sensitizing effects. Cell. 2010; 142:687–698. PMID: 20813258.


18. Griffin BA. How relevant is the ratio of dietary n-6 to n-3 polyunsaturated fatty acids to cardiovascular disease risk? Evidence from the OPTILIP study. Curr Opin Lipidol. 2008; 19:57–62. PMID: 18196988.


19. Lands WE. Biochemistry and physiology of n-3 fatty acids. FASEB J. 1992; 6:2530–2536. PMID: 1592205.


20. Okuyama H. High n-6 to n-3 ratio of dietary fatty acids rather than serum cholesterol as a major risk factor for coronary heart disease. Eur J Lipid Sci Technol. 2001; 103:418–422.


21. Willett WC. The role of dietary n-6 fatty acids in the prevention of cardiovascular disease. J Cardiovasc Med (Hagerstown). 2007; 8(Suppl 1):S42–S45. PMID: 17876199.


22. Mozaffarian D, Ascherio A, Hu FB, Stampfer MJ, Willett WC, Siscovick DS, Rimm EB. Interplay between different polyunsaturated fatty acids and risk of coronary heart disease in men. Circulation. 2005; 111:157–164. PMID: 15630029.


23. Hooper L, Thompson RL, Harrison RA, Summerbell CD, Ness AR, Moore HJ, Worthington HV, Durrington PN, Higgins JP, Capps NE, Riemersma RA, Ebrahim SB, Davey Smith G. Risks and benefits of omega 3 fats for mortality, cardiovascular disease, and cancer: systematic review. BMJ. 2006; 332:752–760. PMID: 16565093.


Fig. 1
Cytotoxicity of C18 fatty acids in RBL-2H3 cells. Cells were incubated with C18 fatty acids (A: stearic acid, B: oleic acid, C: linoleic acid, D: α-linolenic acid) for 24 h in a 5% CO2 incubator at 37℃. The number of living cells was measured using MTT. Results are mean±S.D. from four separate experiments.
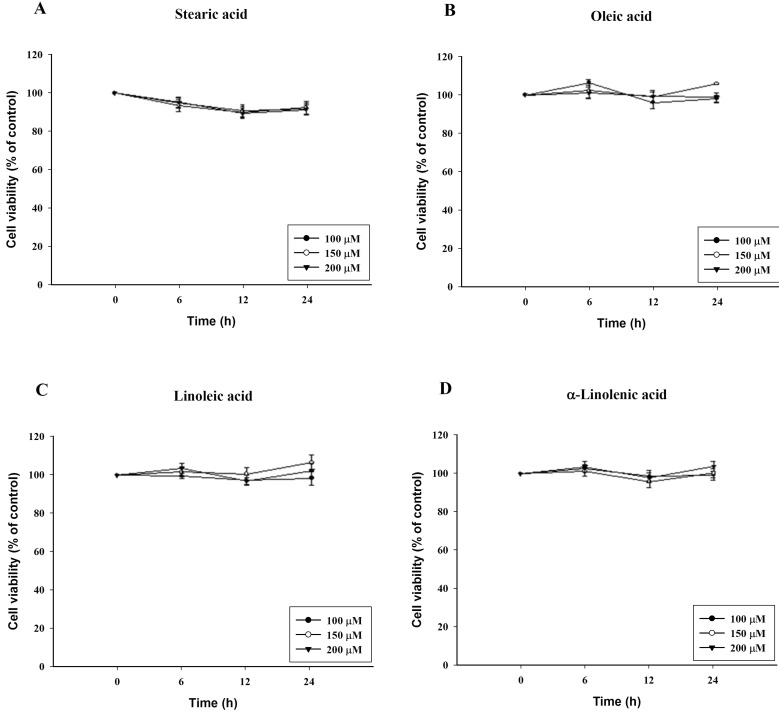
Fig. 2
C18 fatty acid-induced intracellular Ca2+ mobilization. Intracellular Ca2+ mobilization was measured in Fura-2/AM-loaded RBL-2H3 cells. Intracellular Ca2+ concentration induced by a 100-sec exposure to the C18 fatty acids [A: C18 fatty acids, B: stearic acid (SA), C: linoleic acid (LA), D: α-linolenic acid (LN)] was expressed as the ratio of F340/F380. Results are the representative data of four separate experiments.
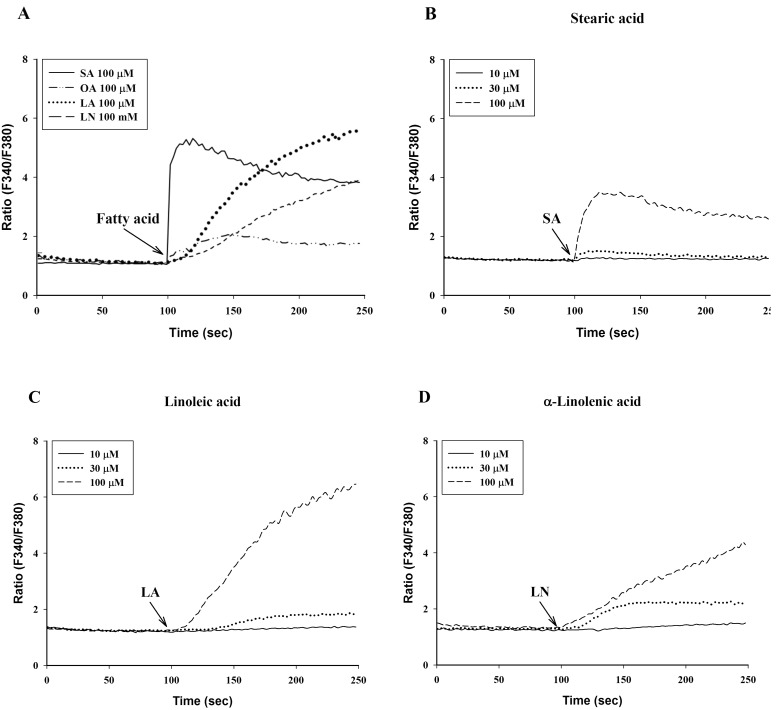
Fig. 3
C18 fatty acids-induced intracellular Ca2+ mobilization in the presence or absence of extracellular Ca2+. Intracellular Ca2+ concentration was induced by a 100-sec exposure to the C18 fatty acids [A: stearic acid (SA, 100 µM), B: linoleic acid (LA, 100 µM), C: α-linolenic acid (LN, 100 µM)]. Results are the representative data of four separate experiments.
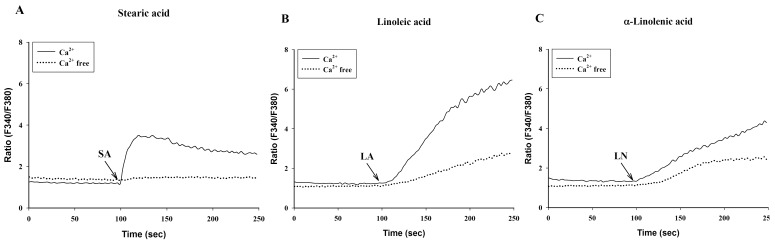
Fig. 4
Effect of verapamil on intracellular Ca2+ mobilization induced by C18 fatty acids. After a 50-sec treatment with 10 µM verapamil (VP), intracellular Ca2+ concentration was induced by a 100-sec exposure to the C18 fatty acids [A: stearic acid (SA), B: linoleic acid (LA), C: α-linolenic acid (LN)]. Results are the representative data of four separate experiments.
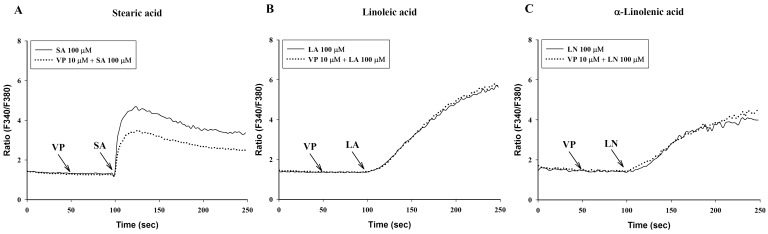
Fig. 5
(A) Dose-response of verapamil (VP) to intracellular Ca2+ mobilization and (B) peak ratio induced by stearic acid (SA, 100 µM). Results are the representative data of four separate experiments. *Significantly different from control (p<0.05).
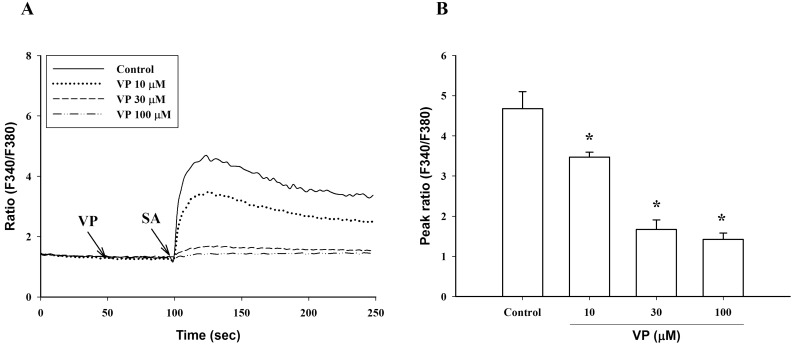
Fig. 6
Effect of bisindolylmaleimide (BIM) on intracellular Ca2+ mobilization induced by a 100-sec exposure to C18 fatty acids [A: stearic acid (SA), B: linoleic acid (LA), C: α-linolenic acid (LN)]. Results are the representative data of four separate experiments.
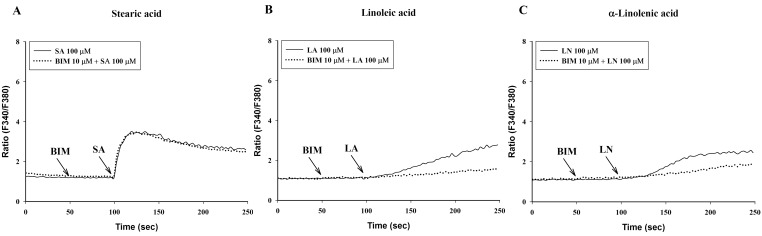
Fig. 7
C18 Fatty acid-induced histamine release in RBL-2H3 cells. (A) Histamine release induced by arachidonic acid (AA, 100 µM), stearic acid (SA, 100 µM), linoleic acid (LA, 100 µM) and α-linolenic acid (LN, 100 µM). (B) Effect of 10 µM bisindolylmaleimide (BIM) on histamine release induced by linoleic acid (LA, 100 µM) and α-linolenic acid (LN, 100 µM). *Significantly different from Control (p<0.05); aSignificantly different from LA (p<0.05); bSignificantly different from LN (p<0.05).
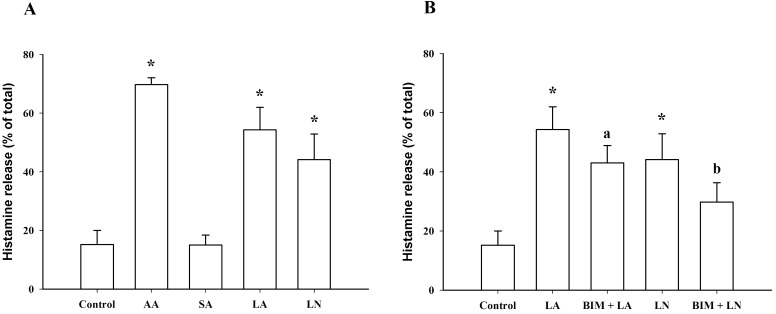