Abstract
Deiters' cells are the supporting cells in organ of Corti and are suggested to play an important role in biochemical and mechanical modulation of outer hair cells. We successfully isolated functionally different K+ currents from Deiters' cells of guinea pig using whole cell patch clamp technique. With high K+ pipette solution, depolarizing step pulses activated strongly outward rectifying currents which were dose-dependently blocked by clofilium, a class III anti-arrhythmic K+ channel blocker. The remaining outward current was transient in time course whereas the clofilium-sensitive outward current showed slow inactivation and delayed rectification. Addition of 5 mM tetraethylammonium (TEA) further blocked the remaining current leaving a very fast inactivating transient outward current. Therefore, at least three different types of K+ current were identified in Deiters' cells, such as fast activating and fast inactivating current, fast activating slow inactivating current, and very fast inactivating transient outward current. Physiological role of them needs to be established.
The modulation of auditory transduction process is mainly controlled by outer hair cells by their electro-motile properties and efferent innervations. Supporting cells in the organ of Corti, however, have been highlighted in the point of modulating auditory transduction process. In Deiters' and Hensen's cells, an efferent innervation to those cells was reported [1,2] and a cholinergic synapse was also found [3]. Intracellular calcium concentration was increased by the extracellular application of acetylcholine to Deiters' cells and this increase was inhibited by strychnine suggesting the presence of alpha 9 acetylcholine receptor [4]. Furthermore, extracellular ATP could increase intracellular calcium ion and this increase was inhibited by nitric oxide through nitric oxide/cyclic GMP pathway in Deiters' and pillar cells [5,6]. Active and reversible movement was also observed in isolated Deiters' cells by increased intracellular calcium [7,8] and supporting cells in the organ of Corti in vivo by intense sound [9]. These findings suggest that the supporting cells, especially Deiters' cells, can be influenced by efferent neurotransmission and may be involved in the auditory transduction process.
In the inner ear, endocochlear potential is the major driving force for the transduction current and ion channels are important structures in this transduction. While the role of Deiters' cells in the active mechanism of cochlea is still not clear, there have been reports on the ion currents in Deiters' cells. Nenov et al. [10] described the outward rectifying potassium current probably similar to the Kv1.5 type channel. They identified the voltage dependent K+ channels which are partially suppressible by potassium channel blockers, such as TEA and/or 4-AP, and also nimodipine, an L-type Ca2+ channel blocker known to block potassium channels. According to these findings, they suggested that more than one type of K+ selective channels may exist in Deiters' cells. Using Charybdotoxin and TEA, Szucs et al. [11] isolated two distinct potassium channels that are different from Kv1.5 type K+ channel in Dieters' cells. Deiters' cells are immersed in the perilymph except the part of phalangeal process, which is facing the endolymph. Because of this difference, a big potassium gradient may be generated through these cells, which may act as a source of cochlear potential and as a K+ buffer of inner ear space. Other than K+ channels, CLC-K chloride channel was also suggested to exist in the Deiters' cells along with fibrocytes in spiral ligament, marginal cells and spiral ganglion cells in adult rat cochlea [12].
In this study, more than two distinct potassium channels were suggested from the differential effects of clofilium and TEA on potassium current in Deiters' cells. Their characteristics and physiological implications will be discussed.
Albino guinea pigs (200~250 g) were obtained from Korean Animal Breeding Laboratory (Seoul). The experimental protocols were in compliance with the guidelines of the National Institute of Health and approved by the University of Ulsan's committee on Use and Care of Animal. Animal care was performed under the supervision of the Laboratory Animal Unit of Asan Institute of Life Science.
Animals were anesthetized with intraperitoneal injection of sodium pentobarbital (50 mg/kg). The cochlea was removed and immersed in normal Tyrode solution (pH 7.4, 300±2 mOsm). The bony wall was immediately removed and the stria vascularis and the tectorial membrane were detached. The organ of Corti was then separated from the spiral lamina using a fine metal probe, and Deiters' cells were dissociated. Dissected strips of organ of Corti were transferred into 50 µl droplet of normal Tyrode solution. A 50 µl aliquot of collagenase (Sigma type IV; 1 mg/ml in normal Tyrode solution) was added, and the strips were incubated for 20 min at room temperature. They were then transferred into a 50 µl droplet of fresh normal Tyrode solution, and gentle trituration of the tissue pieces through a 50 µl Hamilton syringe completed the dissociation procedure. The isolated cells were moved to the experimental bath (50 µl) and allowed to settle for 20 min prior to the experiment. Isolated Deiters' cell showed characteristic feature with a long phalangeal process and the nucleus located in the cell body (Fig. 1).
Whole cell current recording and data analysis were done using a patch clamp amplifier (List EPC-7, Heka, Germany) and pCLAMP software (version 8.0.2, Axon Instruments, Foster City, CA, USA). Patch electrodes were fabricated using thin-walled borosilicate glass (Clark, UK) and heat-polished. When the electrodes were filled with intracellular solution, they had a resistance of around 2~3 MΩ. Membrane currents were filtered at 3 kHz with a four-pole low-pass Bessel filter digitized with a 12-bit A/D converter (Digidata 1200, Axon Instruments) with 10 kHz sampling speed. Recordings were made at 37℃ with continuous perfusion which flowed with a rate of 2 ml/min. The bath volume was 100 µl. After whole cell patch mode was established, the holding potential was usually maintained at -40 mV.
Normal Tyrode solution was used as bath solution and the composition was as follows. (mM) : NaCl 143.5, KCl 5.4, CaCl2 2.0, MgCl2 1.0, HEPES 10, Glucose 11. The pH was adjusted to 7.4 by adding NaOH. The osmolality of the solution was adjusted to 300±2 mOsm by adding NaCl. The composition of intracellular (pipette) solution was as follows (mM) : KCl 140, NaCl 5.0, HEPES 10, Mg-ATP 2.0, MgCl2 1.0, EGTA 0.1. The pH of this solution was corrected to 7.4 by adding KOH and osmolality was adjusted to 300±2 mOsm by adding KCl. All chemicals were obtained from Sigma (St. Louis, MO, USA).
Whole cell capacitances were measured and the average capacitance of Deiters' cells was 36.34±0.71 pF (mean±S.E., n=399). The holding potential was -40 mV and the step pulses were applied from -110 mV to 50 mV with 10 mV increments for 5 sec and the activated current traces and their current voltage relationships were shown in Fig. 2. The current was peaked at 5 msec and very slowly decayed over 5 sec periods. The peak current density was 10.3±0.4 pA/pF (mean±S.E., n=46) at -110 mV and was about 225.6±35.4 pA/pF (mean±S.E., n=46) at 50 mV. Therefore, the activated current showed very strong outward rectifying characteristics which were the same as the previous results [10]. The activation of the current was not significantly affected by extracellular calcium as shown in Fig. 3.
Reactivation time courses were compared at different holding voltages. The reactivation was faster at a lower holding voltage and its time constant was 19 msec at -80 mV and 512 msec at -40 mV, respectively (Fig. 4). Steady state inactivation states of each different voltage were achieved by applying pre-pulses from -100 mV to 50 mV with 5 mV increments for 10 sec. The steady state inactivation curve was obtained and plotted with current amplitudes activated by the application of 50 mV step pulse after each pre-pulses (Fig. 5. ■). The inactivation curve was well fitted by single Boltzmann equation and the half inactivation voltage (Vh) was -29.72 mV and the slope factor (K) was 7.36 mV. The activation curve was obtained and plotted with the peak current amplitude by the step pulses from -80 mV to 50 mV with 5 mV increments after -80 mV pre-pulse for 150 msec which is enough to reactivate the currents completely. The activation curve was not fitted very well with single Boltzmann equation. Instead it was fitted with double Boltzmann equation (Fig. 5. ●). The half activation voltages (Vh) were 10.12 mV and 4.28 mV while slope factors (K) were 8.21 mV and 9.73 mV, respectively. The presence of these two different parameters suggested the existence of more than two types of outward rectifying currents.
Clofilium, the class-III anti-arrhythmic K+ channel blocker, inhibited the outward rectifying current in a dose-dependent manner (Fig. 6A). The blocking effects of clofilium were different between time at the peak and at 100 msec of step pulses as shown in Fig. 6C (peak, ▼; at 100 msec, △). The half-maximal inhibitory concentration (Kh) were 6.1 µM at the peak and 56.5 µM at 100 msec, respectively, showing considerable differences. TEA also had dose-dependent blocking effect but much less potent than clofilium (Fig. 6B, C). The inhibitory effect of TEA was different from those of clofilium. TEA inhibited both the peak current and the current at 100 msec in a similar way. Khs of TEA were 5.9 mM at the peak and 3.6 mM at 100 msec, respectively (peak, ●; 100 msec, ◯). In the presence of very high concentration of TEA, the initial transient outward current was still noticeable. At 30 µM clofilium, more than 90% of slow inactivating current was inhibited whereas the peak of initial transient outward current was inhibited as little as 20% (Fig. 6C, 7B). The remaining currents after 30 µM clofilium were further blocked by 5 mM TEA and the residual currents showed much faster inactivation (Fig. 7C). We isolated a clofilium-sensitive current and a TEA-sensitive current. Clofilium-sensitive currents (Fig. 7D) were obtained by subtracting traces of Fig. 7B from those of Fig. 7A and the resulting clofilium-sensitive currents showed slower activation and slower inactivation kinetics than those of TEA-sensitive. The time to peak current amplitude at 40 mV stimulus was 54.6±4.7 msec (mean±S.E, n=16) which was significantly delayed compared to 5.2±0.6 msec (mean±S.E., n=43) in the control current activation. The TEA-sensitive current was obtained by subtracting traces of Fig. 7C from those of Fig. 7B and was shown in Fig. 7E. It showed a characteristic feature of a rapid activating and rapid inactivating transient outward potassium current. The time to peak current amplitude was 4.7±0.4 msec (mean±S.E., n=14) which is much faster than that of clofilium-sensitive current. The time to peak current amplitude of the residual current (Fig. 7C) was 3.5±0.1 msec (mean±S.E., n=16). I-V curves of each current component were summarized and plotted in Fig. 8A. The peak amplitude of control current at 80 mV was 427±33 pA/pF (mean±S.E., n=12), that of clofilium-sensitive current was 298±30 pA/pF (mean±S.E., n=9), that of TEA-sensitive current was 270±23 pA/pF (mean±S.E., n=9) and that of residual current was 87±12 pA/pF (mean±S.E., n=9).
Using the current trace activated by a depolarizing step pulse to 60 mV in 9 cells, the inactivation time constants (tau) of each condition were fitted and compared. The time constant (tau) of the clofilium-sensitive current was the largest (505±160 ms, mean±S.E.) and the residual current with clofilium and TEA was the smallest (5.6±0.1 ms, mean±S.E.). In case of the TEA-sensitive current, tau was 27.6±3.1 ms (mean±S.E.) (Fig. 8B).
The activation and steady state inactivation curves of three currents are shown in Fig. 9. Each curve was fitted by single Boltzmann equation. In case of activation curve, the half activation voltage and slope factors were 26.4 mV and 16.3 for the clofilium-sensitive current, 22.9 mV and 14.5 for the TEA-sensitive current, and 15.4 mV and 10.8 for the residual current, respectively. The steady state inactivation of clofilium-sensitive current could not be obtained because of the necessity of long prepulse with and without clofilium. The half inactivation voltage and slope factors were -5.6 mV and 4.0 for the TEA-sensitive current, and -13.7 mV and 4.7 for the residual current, respectively. Clofilium-sensitive current must show the more negative half inactivation voltage and the larger slope factors because those of the whole outward rectifying current were -29.7 mV and 7.4, respectively. Clofilium-sensitive current and TEA-sensitive current showed the similar activation and the curves of the residual current was shifted to the left.
Based on the biphasic time course of inactivation (Fig. 7A) and pharmacological separation of it by drugs such as clofilium and TEA, we concluded that the Deiters' cells have more than two types of K+-selective channels and tried to simulate the outward rectifying K+ currents shown in Fig. 7. We assumed the outward rectifying K+ currents have two components; one is fast activating and fast inactivating and the other is fast activating and slow inactivating. Castle [13] proposed that clofilium inhibits the transient outward K+ channels in open state rather than in closed or inactivated state considering the slow onset of inhibition.
In our model, we assumed that the clofilium blocks only the fast activating and slow inactivating component whereas the TEA non-selectively blocks both components. We did not incorporate TEA-resistant component into our model just for simplicity. We adopted C-O-I transition model for fast activating and slow inactivating component since the model can be easily expanded to incorporate the transition of open to blocked state. Hodgkin-Huxley-type model was used for fast activating and fast inactivating component for its simplicity in calculation.
In order to validate our model, we tried to reproduce the experimentally obtained reactivation of currents in Fig. 4. Plotting peaks at different length of prepulse and fitting them to single exponential functions, it was possible to obtain similar time constants with those in Fig. 4 (Fig. 10). Then, we tried to reproduce currents in control, after 30 µM clofilium, and clofilium-sensitive in Fig. 7. Fig. 11 shows the summated current (Fig. 11A) as well as current corresponding to each component (Fig. 11B and 11C). The summated current (Fig. 11A) clearly shows the biphasic time course of inactivation similar to that in Fig. 7A whereas the current corresponding to each component (Fig. 11, 11C) shows mono-exponential time course. In order to simulate the effect of clofilium, we assumed that the clofilium selectively blocks the open state of fast activating and slow inactivating component without affecting the fast activating and fast inactivating component (see appendix). As shown in Fig. 11D, incorporating a transition from open to blocked state into the C-O-I model resulted in a fast inactivating current which is very similar to that in Fig. 7B. The clofilium-sensitive current (Fig. 11E) was obtained by subtracting the current after clofilium (Fig. 11D) from the current without clofilium (Fig. 11A). It faithfully reproduced the slow activating and slow inactivating time course of the experimentally obtained clofilium-sensitive current in Fig. 7D.
Our results showed that the very strong voltage activated outward rectifying K+ currents exist in Deiters' cells of guinea pig cochlea. The steady state activation curve suggested that there may be at least two different kinds of voltage activated K+ current in Deiters' cells. These results were well matched to the previous results [10]. Clofilium, a quaternary nitrogenous compound, was known to block the Kv 1.5 channel as an open channel blocker and showed that clofilium accelerated the inactivating process [14]. The similar changes by clofilium were also shown in our results and therefore it may be possible that our results simply showed the way of clofilium action if major potassium current of Deiters' cells is Kv1.5. But our results are against this hypothesis in many aspects. The first is the activation curve of control current. We could not fit the activation curve to the single Boltzmann equation at all which means that the current activation has at least two distinct different voltage dependences. The second is TEA effect. The residual current with TEA clearly showed, at least, two kinds of inactivating kinetics as shown in Fig. 6B. In addition, without clofilium, 5 mM TEA could block 51% at peak and 61% at 100 msec. With 30 µM clofilium, 5 mM TEA could block 73% at peak and 100% at 100 msec. This kind of effect could not be simply explained by the open channel blocking phenomena. These results strongly suggest that there is a TEA-sensitive component in addition to the clofilium-sensitive component, even though both agents could block both components at a higher concentration. It is very complicated to isolate each current because of overlapping inhibition by both agents. We tried to leave the peak component and to block the slow inactivating component by using 30 µM clofilium which blocked the peak current only 20% and the slow inactivating component more than 90%. In the presence of 30 µM clofilium, TEA-sensitive current was isolated. TEA-sensitive current was very similar to transient outward K+ current. Its inactivation time constant was 28 msec which is in the range of inactivation time constants of transient outward currents [15]. In case of Kv4.3, TEA has virtually no inhibitory effect [16] but in case of Kv1.4 and Kv3.4, TEA was known to be very sensitive blocker [17]. Therefore the TEA-sensitive transient outward current might be Kv1.4 or Kv3.4. The mathematical simulations shown in Fig. 11 also support our idea that the Deiters' cell has a separate entity of channels whose kinetics and pharmacology are different each other. Those simulations faithfully reproduced time courses and voltage-dependence of control current (Fig. 11A), residual current after clofilium (Fig. 11D), and clofilium-sensitive current (Fig. 11E).
What is the residual current in the presence of TEA and clofilium? This residual current may simply reflect the incomplete block of the drugs but the inactivation time constant was too fast to think like that way. And also it showed distinct activation and steady state inactivation curve. Therefore it is probably the other unknown K+ current but its physiological role may be minimal since the amplitude of this current was very small compared to the other current amplitude. The activation and the steady state inactivation curves of three currents were obtained except the steady state inactivation curve of clofilium-sensitive current. This was due to the technical difficulties to maintain the pulses for a long time to obtain the complete data set for the inactivation curve. Those obtained activation and inactivation data were well fitted by single Boltzmann equation which also supported the existence of three distinct K+ currents in Deiters' cell.
Szucs et al. [11] reported that two distinct K+ channels were identified in Deiters' cells. They suggested the corpulent Deiters' cells mainly had slow inactivating K+ current and the lanky Deiters' cells had fast inactivating K+ current in addition. This result also supports our results partially that Deiters' cell had a slow inactivating K+ current and a rapid inactivating K+ current.
K+ channel mutation produced deafness and the related K+ channel genes are KCNQ1 and KCNQ4 [18]. KCNQ1 is related to the endolymphatic fluid production. Even though K+ channel has a crucial role in hearing function, the physiological role is still obscure. Potassium concentration is much higher in endolymph and this huge difference in potassium ion concentration between the compartments mainly contributes to generation of endocochlear potential. Since organ of Corti is located between the perilymph and endolymph, a precise mechanism of ionic homeostasis for potassium ions is required to maintain the physiological process. And also, even though there is a K+ gradient, a K+ channel between the compartments is necessary to produce endocochlear potential which is derived from the K+ diffusion potential [19]. Marcus et al. [20] showed that the knock-out of KCNJ10 in mouse abolished the endocochlear potential. To utilize this endocochlear potential for auditory transduction process, K+ circuit needs to be established. The only way of forming K+ circuit is through cells. Deiters' cells contact the endolymph and also faces the perilymph. Our results and other results [10] showed very large K+ channel activity (44.5 pA/pF at 0 mV in Fig. 8A) which supports Deiters' cell can be a part of K+ circuitry. In this pathway, it may be possible that each different K+ channel play its role in each different region. It was reported that clofilium could decrease endocochlear potential [21]. In frog semicircular canal, endolymphatic side application of clofilium abolished the transepithelial potential [22].
The presence of outward rectifying voltage-gated K+ current has been regarded as an evidence of modulating role of Deiters' cells [10]. Because Deiters' cells can also be depolarized by ATP or acetylcholine [4,6], K+ current-induced prompt repolarization may be needed to be ready for the next stimulation. The presence of the different types of outward rectifying K+ currents from our results suggests that the modulating procedure may be precise and complex in Deiters' cells. The role of outward rectifying current in Deiters' cells is suggested as repolarizing the membrane potential after depolarization driven by stimulations, such as neurotransmitters [10,11]. Outward K+ current can be involved in K+ buffering and transportation [23,24] in organ of Corti. By the K+-Cl- co-transporter, Kcc4 [25], K+ released from outer hair cells could be restored in through Deiters cells [11]. Supporting cells of organ of Corti possess gap junctions and one important role of this epithelial gap junction system is the tunnel of the recirculation of potassium ion. Using double voltage clamp technique, Zhao [26] showed the asymmetric preponderance in voltage gating in the gap junctions between Deiters' cells and suggested that this direction-dependent voltage gating could result in asymmetric current flow and influence K+ passage. Taken together, K+ current in Deiters' cells can maintain the sensitivity of Deiters' cells for the external stimuli, such as ATP, acetylcholine or physical change of the adjacent outer hair cells. In Deiters' cells, the Na+ and Ca2+ current were not identified [10] as shown in our study (data not shown).
In conclusion, functionally different types of voltage gated K+ channels are present in Deiters' cells and these channels may play a role in the K+ buffering around the outer hair cells and prompt repolarization of Deiters' cells.
ACKNOWLEDGEMENTS
This work was supported by the National Research Foundation of Korea (NRF) funded by the Ministry of Science, ICT & Future Planning (2013056801).
References
1. Liberman MC, Dodds LW, Pierce S. Afferent and efferent innervation of the cat cochlea: quantitative analysis with light and electron microscopy. J Comp Neurol. 1990; 301:443–460. PMID: 2262601.


2. Wright CG, Preston RE. Efferent nerve fibers associated with the outermost supporting cells of the organ of Corti in the guinea pig. Acta Otolaryngol. 1976; 82:41–47. PMID: 948984.


3. Burgess BJ, Adams JC, Nadol JB Jr. Morphologic evidence for innervation of Deiters' and Hensens cells in the guinea pig. Hear Res. 1997; 108:74–82. PMID: 9213124.


4. Matsunobu T, Chung JW, Schacht J. Acetylcholine-evoked calcium increases in Deiters' cells of the guinea pig cochlea suggest alpha9-like receptors. J Neurosci Res. 2001; 63:252–256. PMID: 11170174.
5. Chung JW, Schacht J. ATP and nitric oxide modulate intracellular calcium in isolated pillar cells of the guinea pig cochlea. J Assoc Res Otolaryngol. 2001; 2:399–407. PMID: 11833612.


6. Matsunobu T, Schacht J. Nitric oxide/cyclic GMP pathway attenuates ATP-evoked intracellular calcium increase in supporting cells of the guinea pig cochlea. J Comp Neurol. 2000; 423:452–461. PMID: 10870085.


7. Bobbin RP. ATP-induced movement of the stalks of isolated cochlear Deiters' cells. Neuroreport. 2001; 12:2923–2926. PMID: 11588604.


8. Dulon D, Blanchet C, Laffon E. Photo-released intracellular Ca2+ evokes reversible mechanical responses in supporting cells of the guinea-pig organ of Corti. Biochem Biophys Res Commun. 1994; 201:1263–1269. PMID: 8024570.
9. Fridberger A, Flock A, Ulfendahl M, Flock B. Acoustic overstimulation increases outer hair cell Ca2+ concentrations and causes dynamic contractions of the hearing organ. Proc Natl Acad Sci U S A. 1998; 95:7127–7132. PMID: 9618550.
10. Nenov AP, Chen C, Bobbin RP. Outward rectifying potassium currents are the dominant voltage activated currents present in Deiters' cells. Hear Res. 1998; 123:168–182. PMID: 9745965.


11. Szucs A, Somodi S, Batta TJ, Tóth A, Szigeti GP, Csernoch L, Panyi G, Sziklai I. Differential expression of potassium currents in Deiters' cells of the guinea pig cochlea. Pflugers Arch. 2006; 452:332–341. PMID: 16447073.
12. Qu C, Liang F, Hu W, Shen Z, Spicer SS, Schulte BA. Expression of CLC-K chloride channels in the rat cochlea. Hear Res. 2006; 213:79–87. PMID: 16466872.


13. Castle NA. Selective inhibition of potassium currents in rat ventricle by clofilium and its tertiary homolog. J Pharmacol Exp Ther. 1991; 257:342–350. PMID: 2019997.
14. Steidl JV, Yool AJ. Distinct mechanisms of block of Kv1.5 channels by tertiary and quaternary amine clofilium compounds. Biophys J. 2001; 81:2606–2613. PMID: 11606274.


15. Yeola SW, Snyders DJ. Electrophysiological and pharmacological correspondence between Kv4.2 current and rat cardiac transient outward current. Cardiovasc Res. 1997; 33:540–547. PMID: 9093524.


16. Dixon JE, Shi W, Wang HS, McDonald C, Yu H, Wymore RS, Cohen IS, McKinnon D. Role of the Kv4.3 K+ channel in ventricular muscle. A molecular correlate for the transient outward current. Circ Res. 1996; 79:659–668. PMID: 8831489.
17. Rudy B, Kentros C, Vela-Saenz De Miera E. Families of potassium channel genes in mammals: Toward an understanding of the molecular basis of potassium channel diversity. Mol Cell Neurosci. 1991; 2:89–102. PMID: 19912787.


18. Shieh CC, Coghlan M, Sullivan JP, Gopalakrishnan M. Potassium channels: molecular defects, diseases, and therapeutic opportunities. Pharmacol Rev. 2000; 52:557–594. PMID: 11121510.
19. Wangemann P. K+ cycling and the endocochlear potential. Hear Res. 2002; 165:1–9. PMID: 12031509.
20. Marcus DC, Wu T, Wangemann P, Kofuji P. KCNJ10 (Kir4.1) potassium channel knockout abolishes endocochlear potential. Am J Physiol Cell Physiol. 2002; 282:C403–C407. PMID: 11788352.


21. Mori N, Sakagami M, Fukazawa K, Matsunaga T. An immunohistochemical and electrophysiological study on Isk protein in the stria vascularis of the guinea pig. Eur Arch Otorhinolaryngol. 1993; 250:186–189. PMID: 8357611.


22. Rossi ML, Ferrary E, Martini A, Martini M, Pelucchi B, Bernard C, Teixeira M, Sterkers O, Fesce R. The effect of clofilium, a K-channel blocker, on the electrogenic K secretion and the sensory discharge at the frog semicircular canal. Brain Res. 1996; 721:174–180. PMID: 8793098.


23. Lagostena L, Cicuttin A, Inda J, Kachar B, Mammano F. Frequency dependence of electrical coupling in Deiters' cells of the guinea pig cochlea. Cell Commun Adhes. 2001; 8:393–399. PMID: 12064625.


24. Yang J, Wang J. Possible function of outward potassium currents in isolated Deiters' cells of guinea pig cochlea. Chin Med J (Engl). 2002; 115:264–267. PMID: 11940345.
25. Boettger T, Hübner CA, Maier H, Rust MB, Beck FX, Jentsch TJ. Deafness and renal tubular acidosis in mice lacking the K-Cl co-transporter Kcc4. Nature. 2002; 416:874–878. PMID: 11976689.


26. Zhao HB. Directional rectification of gap junctional voltage gating between dieters cells in the inner ear of guinea pig. Neurosci Lett. 2000; 296:105–108. PMID: 11108992.


Fig. 1
Isolated Deiters' cell. The cell is easily recognized by its long phalangeal process (arrow) and nucleus in cell body.

Fig. 2
Activated current traces (A) and I-V curve (B) recorded from Deiters' cells. This shows rapid activating outward rectifying current. Holding at -40 mV in whole cell recording, step pulse with 10 mV increment from -110 mV to 50 mV elicited outward current, showing very strong outward rectification.

Fig. 3
Effects of extracellular calcium change on the activated current traces and their I-V curves. (A) 2 mM Ca2+, (B) 0 mM Ca2+, (C) I-V curves. 2 mM Ca2+ (●) and 0 mM Ca2+ (■).

Fig. 4
Voltage dependent changes of the reactivation time courses. (A) The voltage of prepulse was -80 mV. (B) The voltage of prepulse was -40 mV. (C) The time constant of the current reactivation was 19 msec. (D) The time constant of the current reactivation was 512 msec.
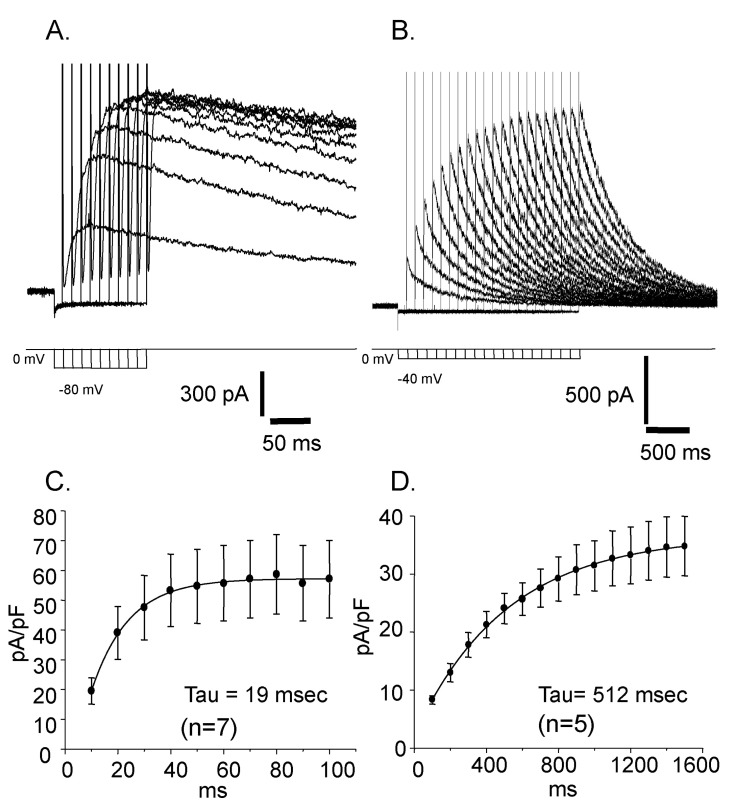
Fig. 5
Steady state inactivation and activation curves. (A) Current traces of the steady state inactivation. (B) Current traces of the activation. (C) The activation curve is fitted by double Boltzmann equation suggesting the presence of more than two channels. Vh: half activation (or inactivation) voltage, K: slope factor. Inactivation curve could be fitted by single Boltzmann equation and the half inactivation voltage (Vh) was -29.72 mV and the slope factor (s) was 7.36. Activation curve was fitted by double Boltzmann equation. The half activation voltages (Vh) and slope factors (s) were -10.12 mV and 4.28 for the first one and 8.21 mV and 9.73 for the second one, respectively.
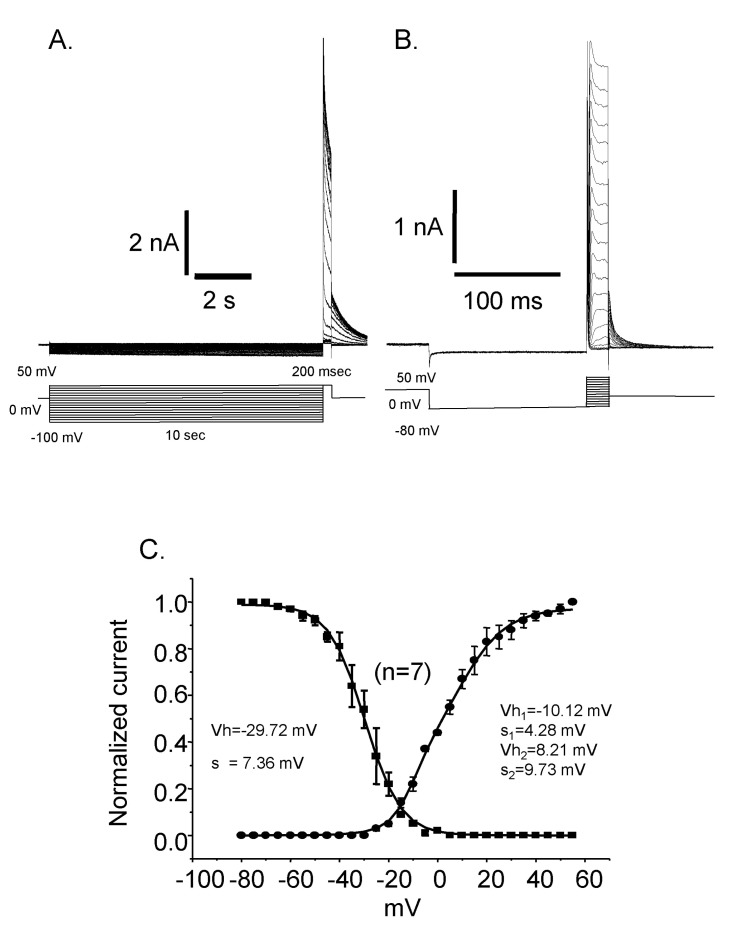
Fig. 6
Dose dependent effect of clofilium and TEA. (A) Current traces with different concentration of clofilium. (B) Current traces with different concentration of TEA. (C) Dose response curves. Effect of TEA on the peak current amplitude (●) and on the current amplitude at 100 msec (◯) (1 mM, n=4; 5 mM, n=11; 40 mM, n=7; 149.8 mM, n=3). Effect of clofilium on the peak current amplitude (▼) and on the current amplitude at 100 msec (△) (3 µM, n=5; 30 µM, n=12; 50 µM, n=5; 100 µM, n=5).
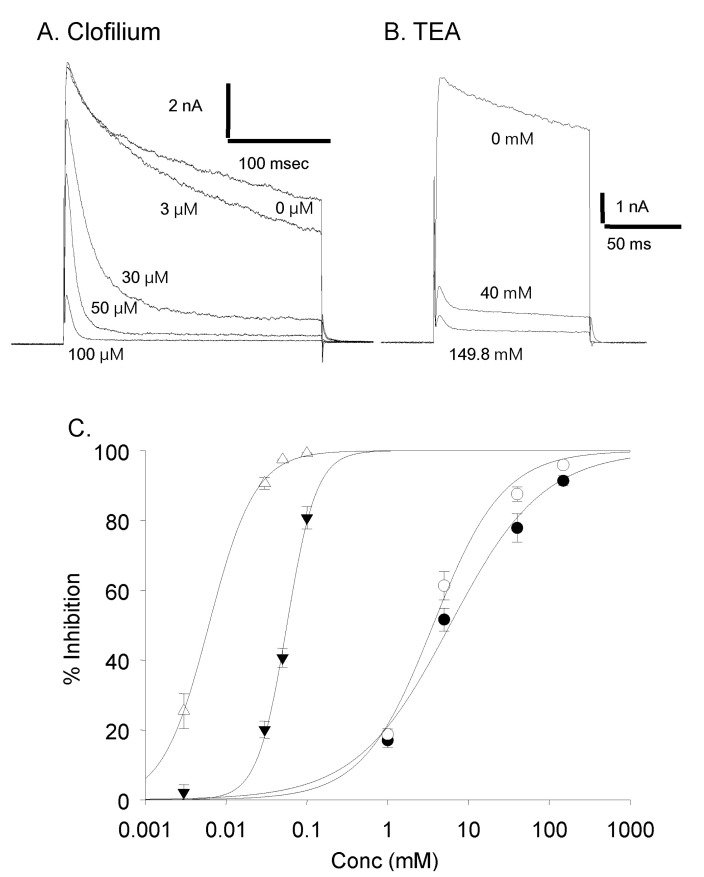
Fig. 7
Isolation of three different types of currents in Deiters' cells. At a concentration of 30 µM of clofilium, control outward rectifying current (A) was blocked leaving transient outward current (B). This residual current was further blocked by 5 mM TEA and only a small transient outward current was identified (C). Clofilium-sensitive current (D) was obtained by subtracting (B) from (A). TEA-sensitive current (E) was obtained by subtracting (C) from (B).
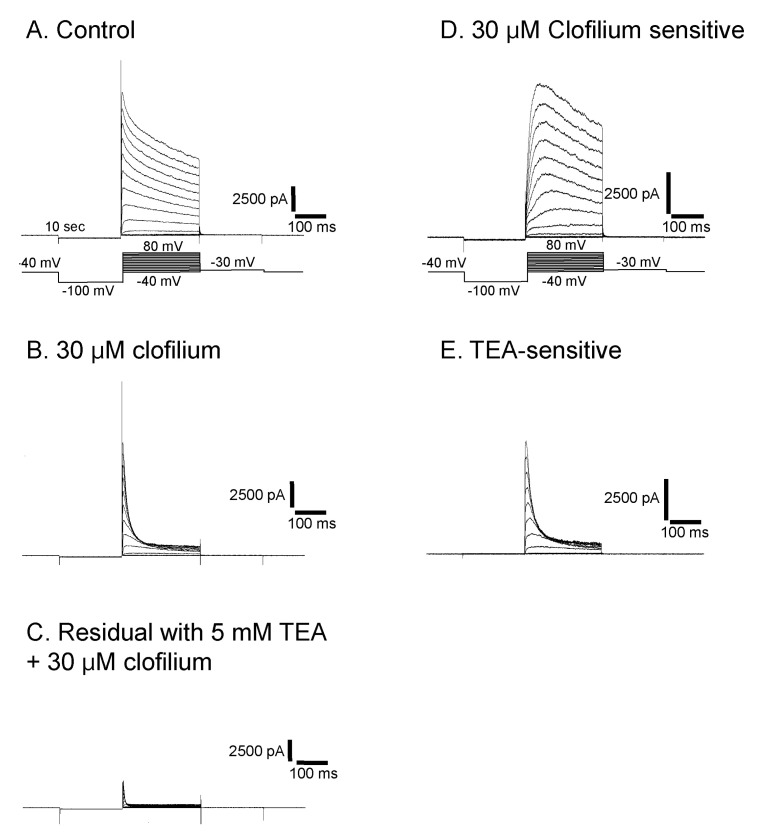
Fig. 8
Voltage-current relationship of three currents. (A) The I-V curves of three currents were similar to that of control currents. The peak current amplitude was used. All the curves showed outward rectification. ●: control current (n=12); ◯: clofiliumsensitive current (n=9); ▼: TEA-sensitive current (n=9); △: clofilium and TEA resistant current (n=9). (B) The time constant (tau) was 505±160 ms in the clofilium-sensitive current, 27.6±3.1 ms in the TEA-sensitive current and 5.6±0.1 ms in the residual current (n=9).

Fig. 9
Characteristics of activation and inactivation of three different currents. the clofilium-sensitive current (●); TEA-sensitive current (◯); clofilium and TEA resistant current (▼). All the currents were fitted by each single Boltzmann equation. Each fitting parameters were shown on the figure.
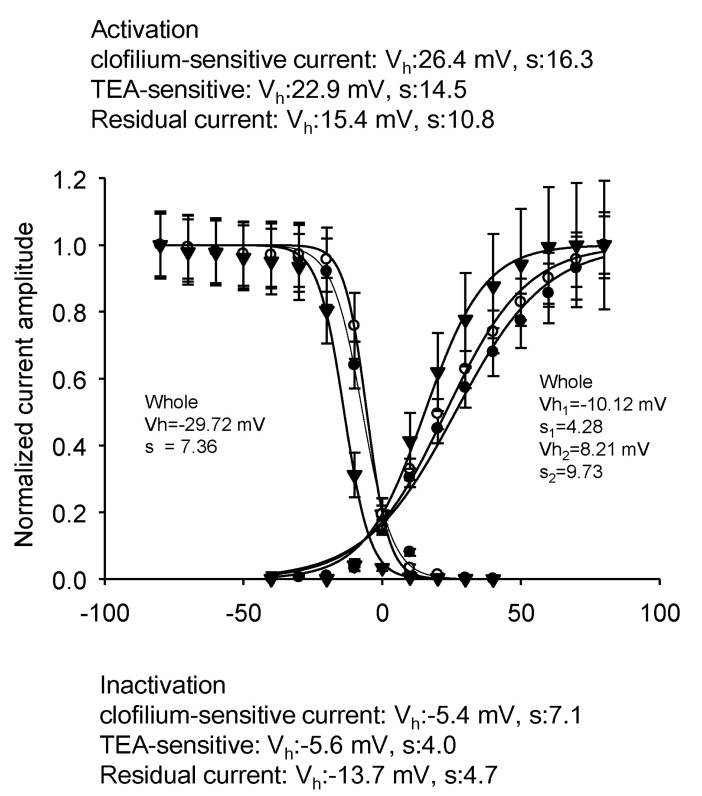
Fig. 10
Validation of our mathematical model. Reactivation time courses from prepulse at -80 mV (A) and -40 mV (B) were obtained by our mathematical simulation. By plotting current density (pA/pF) against length of prepulse and fitting them to single exponential functions (C, D), time constants of reactivation were estimated to be 21 msec at -80 mV and 647 msec at -40 mV, respectively which is comparable to experimentally obtained values in Fig. 4 (19 msec at -80 mV and 512 msec at -40 mV).
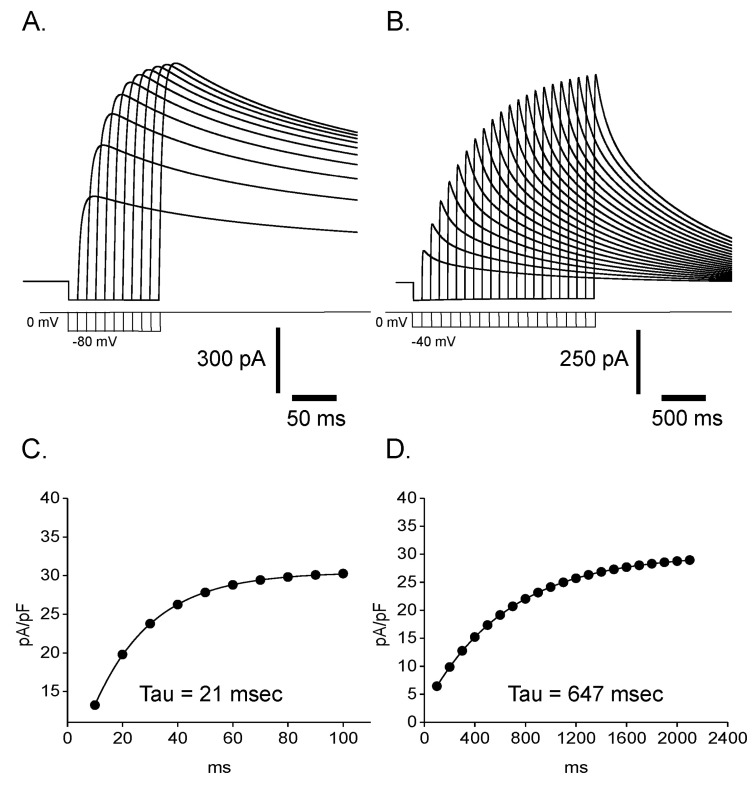
Fig. 11
Mathematical simulation of outward K+ current and clofilium effect in Deiters' cells. Fast activating and fast inactivating current (B) and fast activating and slow inactivating current (C) were summated to reproduce experimentally obtained outward rectifying K+ current in Fig. 7A. Summated current (A) clearly shows biphasic time course of inactivation. The pulse protocol is identical with that used in Fig. 7A. Incorporation of transition from open to blocked state into the C-O-I model successfully reproduced the effect of clofilium in Fig. 7B. Time course and voltage-dependence of simulated current (D) are similar with those of experimentally obtained current in Fig. 7B. Simulated clofilium-sensitive current (E) was obtained by subtracting (D) from (A). Time course and voltage-dependence of simulated current are very similar to those of experimentally obtained current in Fig. 7D.
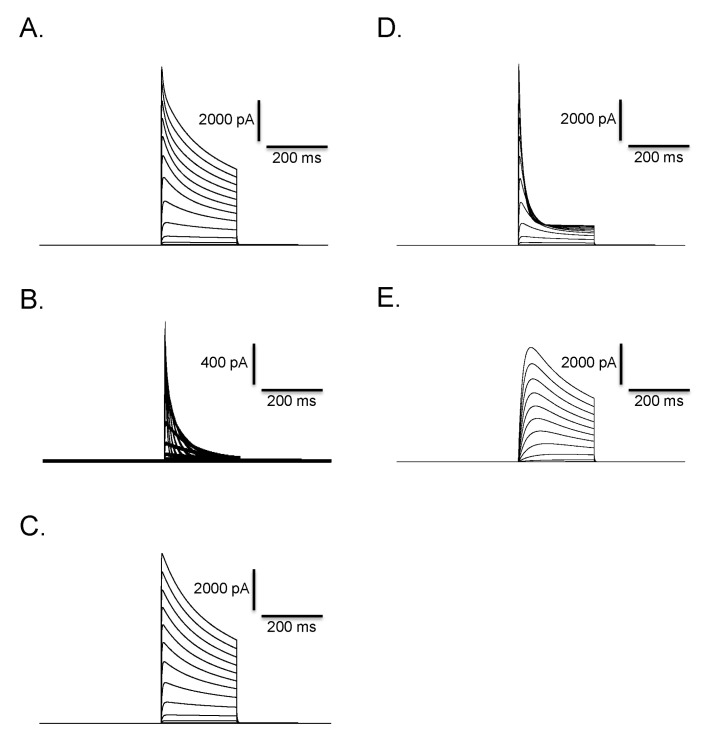