Abstract
Electroacupuncture (EA) is a modified form of acupuncture that utilizes electrical stimulation. We previously showed that EA stimulated rats were divided into responders that were sensitive to EA and non-responders that were insensitive to EA based on the tail flick latency (TFL) test. The dopamine beta-hydroxylase (DBH) gene was more abundantly expressed in the hypothalamus of responder rats than non-responder rats. To determine whether overexpression of DBH gene expression in the hypothalamus modulate EA analgesia, we constructed a DBH encoding adenovirus and which was then injected into the hypothalamus of SD rats. Microinjection of DBH or control GFP virus into the hypothalamus had no changes on the basal pain threshold measured by a TFL test without EA treatment. However, the analgesic effect of EA was significantly enhanced from seven days after microinjection of the DBH virus, but not after injection of the control GFP virus. DBH expression was significantly higher in the hypothalamus of DBH virus injected rat than control GFP virus or PBS injected rats. Moreover, expression of the DBH gene did not affect the body core temperature, body weight, motor function or learning and memory ability. Although the functional role of DBH in the hypothalamus in the analgesic effect of EA remains unclear, our findings suggest that expression of the DBH gene in the hypothalamus promotes EA analgesia without obvious side-effects.
Acupuncture has been practiced for thousands of years in Eastern countries to manage pain, and it is now considered as one of the most important strategies in complementary and alternative medicine. Electroacupuncture (EA) is a modified acupuncture technique that utilizes electrical stimulation. Zusanli (ST36) is one of several the acupuncture point that are used for clinical pain control [1, 2]. Many studies have demonstrated that acupuncture and/or EA stimulation applied to the ST36 acupoint can increase the experimental pain threshold mediated by the release of endogenous opioid peptides [3] and/or by activation of the descending pain inhibitory system [3-10].
However, there are marked individual variations in the sensitivity to EA, which often cause clinical and experimental difficulties. Previous studies reported that 20~40% of rats were insensitive to EA in an acute thermal pain test [tail flick latency (TFL) test] [11]. We previously identified and characterized the genes that cause these differences between the responder rats that were sensitive to EA and the non-responder rats that were insensitive to EA based on the TFL test, using the hypothalamus, a main center of the descending inhibitory system and β-endorphin secretion [12]. Among the genes that were more abundantly expressed in the hypothalamus of responder rats compared to the non-responder rats, dopamine beta-hydroxylase (DBH) gene was significantly higher in the responder rats [12]. DBH catalyzes the conversion of dopamine to norepinephrine. Norepinephrine is an essential neurotransmitter of descending pain inhibitory system. Many pharmacological studies indicate that norepinephrine is antinociceptive and potentiate the analgesic effect [13-15].
Based on those observations, we hypothesized that the expression of DBH gene in the hypothalamus modulates EA analgesia in rats. To explore this hypothesis, we constructed a DBH-encoding adenovirus and a control virus expressing only green fluorescence protein (GFP) and evaluated the effects of microinjection of the viruses into the hypothalamus on EA analgesia in rats using the TFL test. Furthermore, we examined the effects of microinjection of the DBH virus into hypothalamus on the body core temperature, body weight, and learning and memory ability, all of which may be influenced by hypothalamic dysfunction.
Male Sprague-Dawley (SD) rats were housed in groups of four, with water and food available ad libitum in a controlled environment (12:12-hour light:dark cycle, temperature 23±2℃, humidity 50±10%). The SD rats were purchased from Orient Animal Corp. (Korea). The experiments began when the rats were seven weeks old, and all of the procedures involving animals were approved by the Institutional Animal Care and Use Committee of Kyung Hee University.
The protocol for generation of recombinant adenovirus has been described elsewhere [16]. Briefly, human DBH genes were cloned to the multicloning site of a pShuttle-IRES-CMV vector with Xho I / Sal I through PCR. The constructs were bicistronic and contained a GFP gene controlled by a separate cytomegalovirus (CMV) promoter. After homologous recombination, the recombinants were selected with kanamycin and screened by restriction enzyme analysis. Recombinant adenoviral DNA was then extracted from the correctly recombined clones, digested with Pac I to expose its ITR (inverted terminal repeats) and transfected into packaging 293 cells using Lipofectmine 2000 reagent (Invitrogen, Rockville, USA) to produce viral particles. Viruses were harvested and purified using Vivapure AdenoPACK 500 (Vivagen, Seoul, Korea) according to the manufacturer's instructions. The final titer of the adenovirus was about 2.5×109 plaque-forming units (pfu)/ml. Small-volume aliquots were kept in liquid nitrogen, and fresh aliquots were used for each experiment.
SD Rats were positioned in a stereotaxic apparatus (David Kopf Instruments, Tujunga, CA) under isoflurane anesthesia. After making a longitudinal incision of the scalp and disinfecting an exposed dorsal cranium with betadine, a small hole approximately 2 mm in diameter was drilled into the skull over the hypothalamic arcuate nucleus (-3.8 anterior-posterior, 0.5 mediolateral, 9.8 dorsoventral), based on the rat brain atlas Paxinos and Watson (1998). All injections were made using a Hamilton syringe equipped with a 30S gauge beveled needle and attached to a syringe pump (KD Scientific, New Hope, PA). Infusions were made at a rate of 0.2 µl/min for PBS (4 µl of sterile PBS; Sigma, St. Louis, MO), for control GFP virus (1×107 pfu/4 µl) and for DBH virus (1×107 pfu/4 µl). Following injection, the syringe was left in place for an additional 10 min, after which the needle was removed from the brain very slowly.
We allowed the rats to adapt to handling for at least three weeks to minimize stress so that they would remain keep clam during the TFL testing and EA stimulation [17,18]. This acclimation enabled us to eliminate the need for anesthetics or cylindrical restrainers that cause the TFL values to be more variable (unpublished observations). Rats were individually placed on the palm of an experimenter's hand with its tail between the middle and ring fingers, and their head and back were continuously rubbed and stroked so that they became accustomed to being handled. The antinociceptive response was assessed by the TFL test, which records tail-flick latency as the time from the onset of stimulation to the withdrawal of the tail from a heat stimulus produced by a light beam in the TFL test. Three successive assessments of TFL were recorded at time intervals of 1-min and these values were averaged. The cut-off time was set at 15 s to eliminate tissue damage. For EA stimulation, a pair of stainless steel acupuncture needles (0.25 mm in diameter and 3 cm long) was inserted into the Zusanli acupoint (ST36) on the right leg located in the tibialis cranials muscle, 5 mm below lateral to the anterior tubercle of the tibia, and into the point 5 mm distal from the first needle. The two acupuncture needles, which were vertically inserted to a depth of 5 mm, were connected to an electrical stimulator to generate electrical stimulation, and train pulses (2 Hz, 0.5 ms pulse duration) and an intensity of the threshold of muscle contraction (0.2~0.3 mA) were then applied for 20 minutes. To determine if adenoviral gene transfer of DBH alone changes the TFL, the baseline was recorded before starting the experiments. We then compared the TFL between the DBH virus-injected (n=16) and GFP control virus-injected rats (n=16) in the absence of EA stimulation at -1d, 3d, 7d and 14d following viral injection. We also compared the increase in the EA analgesia ratio between the DBH virus-injected (n=16) and the GFP control virus-injected (n=16) rats to determine if viral gene transfer of DBH increased EA-induced analgesic effects. Following EA stimulation at -1d, 3d, 7d and 14d after viral injection, the average of three successive TFL assessments was determined. The effects of viral gene transfer on EA analgeisa were then presented as percent changes from the pre-injection TFL (i.e. -1d TFL) according to the following formula:
We evaluated body core temperature (rectal temperature) and body weight in the DBH virus-injected and GFP control virus-injected rats (n=7~8, per group) at -1d, 3d and 7d after viral injection to determine if microinjection of the DBH virus into the hypothalamus induced side effects. Learning and memory ability were also tested based on passive avoidance 7d after viral injection as previously described [19]. The apparatus consisted of equal-sized light and dark chambers (28×23.5×20 cm) separated by a guillotine door (6×6.5 cm) leading to a runway (platform) at one end and to the grid floor of a dark chamber for electrical foot shock. The retention test session was measured when the rats entered the dark chamber. The hesitation time was measured, with the maximum time being set at 90 s.
Animals were anesthetized with chloral hydrate (360 mg/kg, i.p.) at 15 days after injection and transcardially perfused with saline solution containing 0.5% sodium nitrate and heparin (10 U/ml), followed by fixation with 4% paraformaldehyde dissolved in 0.1M phosphate buffer (PB). Brains were removed and postfixed overnight at 4℃ in buffered 4% paraformaldehyde, and stored at 4℃ in 30% sucrose solution until they sank. Brains were frozen sectioned using a sliding microtome into 40 µm coronal sections and collected in twelve separate series. For Nissl staining, some of the Hypothalamus tissues were mounted on gelatin-coated slides, dried for 1 h at RT, stained with 0.5% cresyl violet (Sigma), dehydrated, coverslipped, and then analyzed under a bright-field microscope (Olympus Optical). The correct transfection of the adenovirus was verified by the presence of green fluorescence in the hyphothalamus under a confocal microscope (Zeiss, Germany).
For analyses of DBH expression, the ipsilateral hypothalamus were dissected 7 days after injection with PBS, control GFP or DBH virus in the absence of EA stimulation. Hypothalamic samples were homogenized with ice-cold lysis buffer containing 20 mM Tris-HCl, pH 7.5, 1 mM EDTA, 5 mM MgCl2, 1 mM dithiothreitol, 0.1 mM phenylmethylsulfonyl fluoride and protease inhibitor cocktail (Sigma) in a Dounce homogenizer (Wheaton, Millville, NJ, USA). Proteins (50 µg/lane) were separated by 10% sodium dodecyl sufate-polyacrylamide gel (SDS-PAGE), transferred to polyvinylidene difluoride membrane (Millipore, Bedford, MA USA) using an electrophoretic transfer system (Bio-Rad Laboratories, Hercules, CA, USA), and subjected to immunoblotting with rabbit anti-DBH (1:500; Santa Cruz Biotechnology, Santa Cruz, CA, USA). The blots were subsequently stripped and re-probed with rabbit anti-actin (1:2000; Cell Signaling Technology, Beverly, MA, USA).
In our previous study, we showed that EA stimulated rats were divided into responders that were sensitive to EA and non-responders that were insensitive to EA based on the results of a TFL test. The ratio of responders to non-responders stimulated EA was approximately 3:2. In addition, the TFL increase ratio was 72.3% and 11.9% for responders and non-responders following EA stimulation, respectively [12,20]. We previously found that the DBH gene (gene profiling) was more abundantly expressed in the hypothalamus of responders than non-responders based on the TFL test [12]. Based on these results, we evaluated to prioritize the effects of adenoviral gene transfer of DBH into the hypothalamus on EA analgesia in responders and non-responders. As a result, both the responder and the non-responder rats showed a significant increase in TFL to a similar extent following microinjection of the DBH virus (data not shown) (i.e. the initial responders became more responsive to EA after the viral expression of DBH and the initial non-responders rats became responders). Therefore, we did not classify the rats into responders or non-responders in the current study.
To determine if gene transfer of DBH affects the basal pain threshold and analgesic effect of EA stimulation, we injected adenovirus co-expressing the DBH and GFP genes into the hypothalamus. As a control, the adenovirus expressing the GFP gene alone was injected into a different group of rats. Next, the change in TFL or the increase in the ratio of EA analgesia in the DBH virus-injected rats was compared to GFP control virus-injected rats at -1, 3, 7 and 14 days following viral injection. As shown in Fig. 1A, there were no significant differences in the TFL values of the DBH virus-injected and the GFP control virus-injected rats during the 2-week experimental period (t-test; t=0.5, df=30, p=0.618 at -1 day; t=0.34, df=30, p=0.739 at 3 days; t=0.34, df=30, p=0.738 at 7 days, t=1.5, df=30, p=0.139 at 14 days). In addition, TFL values measured at 3, 7 and 14 days after the injection of DBH or control virus were not significantly different from the value at -1 day following the injection (ANOVA; F3,45=3.975, p>0.05, DBH; F3,45=1.230, p>0.05, control). Fig. 1B shows the ratio of EA analgesia following microinjection of the DBH or GFP control virus. The EA-induced analgesic effect gradually increased in the DBH virus-injected rats, but not in the GFP control virus-injected rats. A statistically significant increase in EA analgesia was observed from 7 days after injection of the DBH virus (t-test; t=2.4, df=30, p=0.025 at 3 days; t=3.8, df=30, p=0.001 at 7 days; t=2.4, df=30, p=0.024 at 14 days).
To confirm the expression of the DBH adenoviral gene, we performed confocal microscopic analysis at 15 days after viral injection. The results revealed the localization of GFP expression in the adenoviral injected hypothalamus (Fig. 2A). Moreover, Nissle staining showed the location of the needle tip in the hypothalamus, showing only a little tissue damage, which was primarily limited to the needle track (Fig. 2B, D). The hypothalamic DBH expression was analyzed by western blotting using anti-DBH antibody at 7 days after viral injection. DBH expression in the DBH virus-injected rats was also significantly higher than GFP control virus-injected rats (Fig. 2C).
Because the hypothalamus controls a variety of body functions in addition to pain modulation, we determined if microinjection of the DBH virus into the hypothalamus generates side-effects. There were no significant differences in body temperature (rectal temperature) (Fig. 3A) and body weight gain (Fig. 3B) between the DBH virus-injected rats and the GFP control virus-injected rats at -1, 3 and 7 days after viral injection (body temperature in the GFP group versus DBH group: 36.9±0.1℃, 37.0±0.2℃ and 37.0±0.1℃ versus 36.9±0.1℃, 37.0±0.1℃ and 37.0±0.1℃; body weight gain: 288.9±4.1, 325.8±9.1 and 357.1±4.8 g versus 292.0±2.8, 335.7±6.9 and 358.3±5.4 g). In addition, learning and memory ability were assessed by a passive avoidance test administered 7 days after viral injection. The test revealed no signs of memory impairment in the GFP virus-injected and the DBH virus-injected rats because the hesitation time of all rats in both groups was greater than the cut-off time (90 seconds) (Fig. 3C).
Although it has been difficult to establish the biological basis of acupuncture, it has long been a unique method used to treat various diseases and to relieve pain in East Asia, and it is a common complementary and alternative medicine in Western countries [21]. Previous studies have shown that acupuncture or EA increases the experimental pain threshold in animals as well as humans and that EA is more effective than acupuncture alone [6,9,22]. More recently, reasonable neurophysiological theories have been reported that signaling molecules, such as opioid peptides, glutamate, 5-hydroxytryptamine, adenosine, and cholecystokinin octapeptide, are involved in the acupuncture induced analgesia [5,8,23,24]. Specifically, low frequency EA stimulation, such as the 2 Hz EA that was used in the present study, activates the arcuate nucleus of the hypothalamus leading to the release of beta-endorphin and subsequent activation of the downstream descending inhibitory system [3].
The differences in the response to pain and analgesics have been studied in many research fields and the results of these studies have indicated that it might be attributed to genetic factors [25-27]. However, many of these studies have been conducted to evaluate only a single factor. Therefore, we conducted a cDNA microarray experiment to assay the expression of thousands of genes in parallel and identified the genes that were differentially expressed in the hypothalamus of responder and non-responder rats that received EA analgesia. The microarray analysis revealed that the DBH gene was expressed in abundance in the responder group. In addition, brain factor-1, which is a transcription factor that is essential for the proliferation of progenitor cells in the cerebral cortex [28], and the acetylcholinesterase T subunit, which is the only catalytic subunit involved in all vertebrate cholinesterases [29], was also expressed abundantly in the responder group. Conversely, the neurodegeneration markers, tau and cathepsin B [30,31], were abundantly expressed in the non-responder group. In a previous study, we showed that the microinjection of the acetylcholinesterase T (AChET) adenovirus into the hypothalamus significantly enhanced the EA analgesia of without side-effect on the body core temperature, body weight, or learning and memory ability [20].
The present study focused on the relationship of EA analgesia and DBH expression in the hypothalamus. DBH is the catalytic enzyme involved in the conversion of dopamine to norepinephrine, which is a key neurotransmitter of the endogenous pain inhibitor system. Recent report showed that norepinephrine played an important role in antinociceptive modulation of hypothalamic paraventricular nucleus (PVN), with the result that pain stimulation increased endogenous norepinephrine and microinjection of norepinephrine into the PVN elevated pain threshold [13]. Moreover DBH deficiency caused chronic thermal hyperalgesia associated with a lack of norepinephrine [32].
To explore the role that the expression of DBH in the hypothalamus plays on EA analgesia in vivo, we constructed an adenovirus that expressed DBH and GFP control virus. The data obtained in this study revealed that there was no difference in the TFL value of DBH virus-injected and control virus-injected rats, and that the post-injection TFL values did not differ from the pre-injection TFL values in either group (Fig. 1A). These findings indicate that the basal pain threshold was not changed by adenoviral gene transfer of DBH in the hypothalamus. However, the present study clearly demonstrates that microinjection of the DBH virus, but not the control virus, potentiates the analgesic effect of EA (Fig. 1B). Taken together, these findings suggest that adenoviral expression of DBH in the hypothalamus enhances EA analgesia without affecting the basal pain threshold. The influence of the hypothalamus on body physiology is diverse. Indeed, it controls a variety of body functions, such as the autonomic nervous system and the feeding and neuroendocrine systems. Therefore, we evaluated microinjection of the DBH virus into the hypothalamus to determine if it generated side-effects that might disturb the exact analysis of the TFL data (Fig. 3). The results showed that DBH expression in the hypothalamus does not affect the body core temperature, body weight, or learning and memory ability, which suggests that viral gene transfer of DBH into the hypothalamus produces no apparent side-effects.
Currently, we cannot sufficiently explain why the results of basal pain threshold after manipulations of the DBH and norepinephrine in the present study and the others are different. Contradictory to our data showing no changes in baseline pain threshold in the TFL test after microinjection of DBH virus into the hypothalamic arcuate nucleus, DBH knockout mice showed thermal hyperalgesia in the hotplate (50℃) and cold-plate (4℃) test, as compared to their littermate controls [32]. In addition, microinjection of norepinephrine into the rat hypothalamic PVN dose-dependently elevated basal pain threshold measured by the potassium iontophoresis-induced tail flick test [13]. The differences in the manipulation site of the hypothalamus (arcuate nucleus vs. PVN), in the manipulation method (viral gene transfer vs. direct acute injection or genetic deletion resulting in DBH KO of the whole nervous system) and in the strain used (WT rats vs. KO mice) may contribute to such contradictory results. Specifically, the role of the hypothalamic arcuate nucleus in mediating EA analgesia is more related to the endotenous opioids (i.e. β-endorphin) [33,34]. Thus, it would be very interesting to identify a functional interrelationship between the noradrenergic system and opioid system in the hypothalamic arcuate nucleus during EA analgesia.
Even though many reports have indicated that the supraspinal mechanisms are involved in the TFL test, it is mainly based on a spinal reflex [35]. In addition, it would be of high importance to investigate the analgesic effects of EA on chronic pathological pain, such as neuropathic pain [36], the mechanism of which is somewhat different from acute nociceptive pain like the TFL test. Thus, further studies using non-reflexive (operant) pain assays, spontaneous pain behavior tests and chronic pain models [37] might provide a better understanding of EA-induced analgesia.
We found that expression of DBH in the hypothalamus is closely associated with individual differences in response to EA analgesia. Although, the functional role that DBH in the hypothalamus plays in the analgesic effect of EA is unclear, our findings suggest that expression of the DBH gene in the hypothalamus promotes EA analgesia without obvious side-effects. Taken together, these data provide a new perspective regarding the role that peripheral somatic stimulation plays in pain control.
References
1. Lee H, Lee JY, Kim YJ, Kim S, Yin C, Khil JH, Kwon K, Choi SM, Lee H, Park HJ. Acupuncture for symptom management of rheumatoid arthritis: a pilot study. Clin Rheumatol. 2008; 27:641–645. PMID: 18193382.


2. Lin JG, Lo MW, Wen YR, Hsieh CL, Tsai SK, Sun WZ. The effect of high and low frequency electroacupuncture in pain after lower abdominal surgery. Pain. 2002; 99:509–514. PMID: 12406527.


3. Han JS. Acupuncture: neuropeptide release produced by electrical stimulation of different frequencies. Trends Neurosci. 2003; 26:17–22. PMID: 12495858.


4. Baek YH, Choi DY, Yang HI, Park DS. Analgesic effect of electroacupuncture on inflammatory pain in the rat model of collagen-induced arthritis: mediation by cholinergic and serotonergic receptors. Brain Res. 2005; 1057:181–185. PMID: 16139820.


5. Chang FC, Tsai HY, Yu MC, Yi PL, Lin JG. The central serotonergic system mediates the analgesic effect of electroacupuncture on ZUSANLI (ST36) acupoints. J Biomed Sci. 2004; 11:179–185. PMID: 14966368.
6. Kim SK, Park JH, Bae SJ, Kim JH, Hwang BG, Min BI, Park DS, Na HS. Effects of electroacupuncture on cold allodynia in a rat model of neuropathic pain: mediation by spinal adrenergic and serotonergic receptors. Exp Neurol. 2005; 195:430–436. PMID: 16054138.


7. Kanai S, Taniguchi N, Kanda K, Higashino H. Study of acupuncture stimulation on experimental osteopenia. Orient Pharm Exp Med. 2006; 6:79–85.
8. Takeshige C, Sato T, Mera T, Hisamitsu T, Fang J. Descending pain inhibitory system involved in acupuncture analgesia. Brain Res Bull. 1992; 29:617–634. PMID: 1422859.


9. Ulett GA, Han S, Han JS. Electroacupuncture: mechanisms and clinical application. Biol Psychiatry. 1998; 44:129–138. PMID: 9646895.


10. Murotani T, Ishizuka T, Nakazawa H, Wang X, Mori K, Sasaki K, Ishida T, Yamatodani A. Possible involvement of histamine, dopamine, and noradrenalin in the periaqueductal gray in electroacupuncture pain relief. Brain Res. 2010; 1306:62–68. PMID: 19819232.


11. Lee G, Rho S, Shin M, Hong M, Min Bi, Bae H. The association of cholecystokinin-A receptor expression with the responsiveness of electroacupuncture analgesic effects in rat. Neurosci Lett. 2002; 325:17–20. PMID: 12023057.


12. Sur YC, Rho SW, Lee GS, Ko EJ, Hong MC, Shin MK, Min BI, Bae HS. Gene expression profile of the responder vs. the non-responder to the acupuncture mediated analgesic effects. Korean J Orient Physiol Pathol. 2003; 17:633–642.
13. Zhou XJ, Yang J, Yan FL, Wang DX, Li XY, Fan XQ, Hao F, Yan XQ, Li XP, Li H, Liu WY, Lin BC. Norepinephrine plays an important role in antinociceptive modulation of hypothalamic paraventricular nucleus in the rat. Int J Neurosci. 2010; 120:428–438. PMID: 20504214.


14. Zhang C, Davies MF, Guo TZ, Maze M. The analgesic action of nitrous oxide is dependent on the release of norepinephrine in the dorsal horn of the spinal cord. Anesthesiology. 1999; 91:1401–1407. PMID: 10551592.


15. Monasky MS, Zinsmeister AR, Stevens CW, Yaksh TL. Interaction of intrathecal morphine and ST-91 on antinociception in the rat: dose-response analysis, antagonism and clearance. J Pharmacol Exp Ther. 1990; 254:383–392. PMID: 1974633.
16. He TC, Zhou S, da Costa LT, Yu J, Kinzler KW, Vogelstein B. A simplified system for generating recombinant adenoviruses. Proc Natl Acad Sci U S A. 1998; 95:2509–2514. PMID: 9482916.


17. Ko ES, Kim SK, Kim JT, Lee G, Han JB, Rho SW, Hong MC, Bae H, Min BI. The difference in mRNA expressions of hypothalamic CCK and CCK-A and -B receptors between responder and non-responder rats to high frequency electroacupuncture analgesia. Peptides. 2006; 27:1841–1845. PMID: 16472889.


18. Lee GS, Han JB, Shin MK, Hong MC, Kim SW, Min BI, Bae H. Enhancement of electroacupuncture-induced analgesic effect in cholecystokinin-A receptor deficient rats. Brain Res Bull. 2003; 62:161–164. PMID: 14638390.


19. Shim I, Ha Y, Chung JY, Lee HJ, Yang KH, Chang JW. Association of learning and memory impairments with changes in the septohippocampal cholinergic system in rats with kaolin-induced hydrocephalus. Neurosurgery. 2003; 53:416–425. discussion 425. PMID: 12925261.


20. Kim SK, Park JY, Koo BH, Lee JH, Kim HS, Choi WK, Shim I, Lee H, Hong MC, Shin MK, Min BI, Bae H. Adenoviral gene transfer of acetylcholinesterase T subunit in the hypothalamus potentiates electroacupuncture analgesia in rats. Genes Brain Behav. 2009; 8:174–180. PMID: 19077179.


21. Kaptchuk TJ. Acupuncture: theory, efficacy, and practice. Ann Intern Med. 2002; 136:374–383. PMID: 11874310.


22. Kim JH, Min BI, Schmidt D, Lee HJ, Park DS. The difference between electroacupuncture only and electroacupuncture with manipulation on analgesia in rats. Neurosci Lett. 2000; 279:149–152. PMID: 10688051.


24. Zhang RX, Lao L, Wang L, Liu B, Wang X, Ren K, Berman BM. Involvement of opioid receptors in electroacupuncture-produced anti-hyperalgesia in rats with peripheral inflammation. Brain Res. 2004; 1020:12–17. PMID: 15312782.


25. Mogil JS. The genetic mediation of individual differences in sensitivity to pain and its inhibition. Proc Natl Acad Sci U S A. 1999; 96:7744–7751. PMID: 10393892.


26. Mogil JS, Wilson SG, Chesler EJ, Rankin AL, Nemmani KV, Lariviere WR, Groce MK, Wallace MR, Kaplan L, Staud R, Ness TJ, Glover TL, Stankova M, Mayorov A, Hruby VJ, Grisel JE, Fillingim RB. The melanocortin-1 receptor gene mediates female-specific mechanisms of analgesia in mice and humans. Proc Natl Acad Sci U S A. 2003; 100:4867–4872. PMID: 12663858.


27. Zubieta JK, Heitzeg MM, Smith YR, Bueller JA, Xu K, Xu Y, Koeppe RA, Stohler CS, Goldman D. COMT val158met genotype affects mu-opioid neurotransmitter responses to a pain stressor. Science. 2003; 299:1240–1243. PMID: 12595695.
28. Dou C, Lee J, Liu B, Liu F, Massague J, Xuan S, Lai E. BF-1 interferes with transforming growth factor beta signaling by associating with Smad partners. Mol Cell Biol. 2000; 20:6201–6211. PMID: 10938097.
29. Massoulié J, Anselmet A, Bon S, Krejci E, Legay C, Morel N, Simon S. The polymorphism of acetylcholinesterase: post-translational processing, quaternary associations and localization. Chem Biol Interact. 1999; 119-120:29–42. PMID: 10421436.


30. Gray J, Haran MM, Schneider K, Vesce S, Ray AM, Owen D, White IR, Cutler P, Davis JB. Evidence that inhibition of cathepsin-B contributes to the neuroprotective properties of caspase inhibitor Tyr-Val-Ala-Asp-chloromethyl ketone. J Biol Chem. 2001; 276:32750–32755. PMID: 11427531.


31. Tanemura K, Murayama M, Akagi T, Hashikawa T, Tominaga T, Ichikawa M, Yamaguchi H, Takashima A. Neurodegeneration with tau accumulation in a transgenic mouse expressing V337M human tau. J Neurosci. 2002; 22:133–141. PMID: 11756496.


32. Jasmin L, Tien D, Weinshenker D, Palmiter RD, Green PG, Janni G, Ohara PT. The NK1 receptor mediates both the hyperalgesia and the resistance to morphine in mice lacking noradrenaline. Proc Natl Acad Sci U S A. 2002; 99:1029–1034. PMID: 11805341.


33. Takeshige C. Synaptic transmission in acupuncture analgesia. Tokyo: Showa University School of Medicine;1992.
34. Han JS. The neurochemical basis of pain relief by acupuncture. Beijing: Chinese Medical Science and Techonology Press;1987.
35. Le Bars D, Gozariu M, Cadden SW. Animal models of nociception. Pharmacol Rev. 2001; 53:597–652. PMID: 11734620.
36. Kim W, Kim SK, Min BI. Mechanisms of electroacupuncture-induced analgesia on neuropathic pain in animal model. Evid Based Complement Alternat Med. 2013; 2013:436913. PMID: 23983779.


37. Mogil JS. Animal models of pain: progress and challenges. Nat Rev Neurosci. 2009; 10:283–294. PMID: 19259101.


Fig. 1
Effects of adenoviral gene transfer of DBH on the basal pain threshold and EA analgesia. (A) Basal TFL values in the DBH virus-injected and GFP control virus-injected rats at -1, 3, 7 and 14 days after viral injection. Data are presented as the mean±SEM. p>0.05, DBH versus GFP group at each day as determined by the unpaired t-test; p>0.05, -1 day TFL versus 3, 7 and 14 days TFL in either group as determined by one-way ANOVA. (B) The increase ratio of EA-induced analgesic effect at 3, 7 and 14 days following viral injection, compared to the EA effect prior to viral injection. *p<0.05 and ***p<0.001, between the two groups as determined by the unpaired t-test. The data are the means±S.E.M (n=16/group).
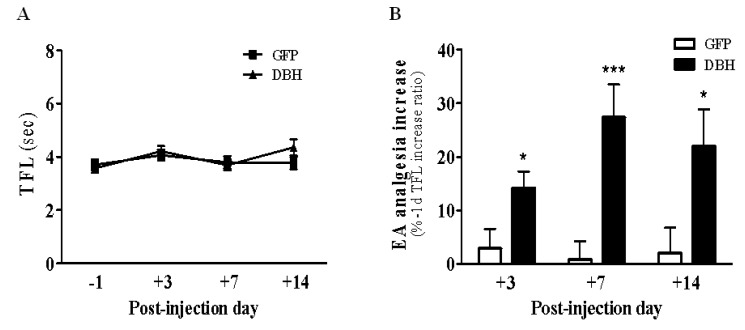
Fig. 2
Verification of the correct injection and infection of the adenovirus into the hypothalamus. Tissues were prepared 15 days after PBS or virus injection. (A) Representative confocal microphotographs of GFP fluorescence in the hypothalamus from DBH virus injected rats. 3v, 3rd ventricle. Scale bar, 200 µm. (B) Representative photographs of the Nissle staining following microinjection of the viral suspension. Arrow indicates needle track. (C) Western blot analysis showing significantly increase of DBH in the DBH virus-injected rat compared to PBS or GFP control virus injected rats at 7 days later virus injection. (D) High magnification of B.
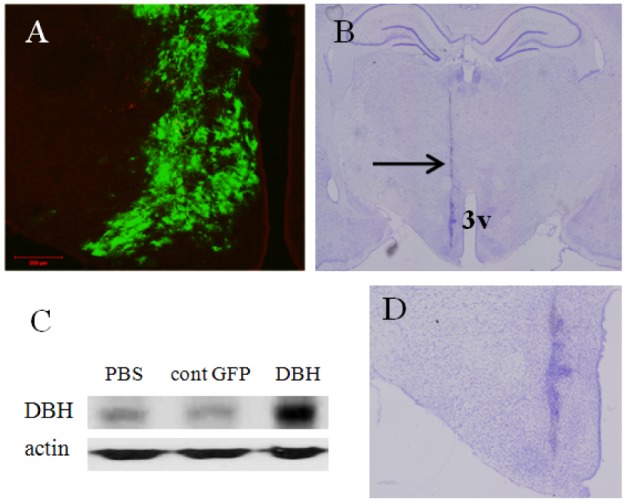
Fig. 3
Effects of adenoviral gene transfer of DBH on body temperature, body weight, and learning and memory ability. The body temperature (A) and body weight (B) of the DBH virus-injected and the GFP control virus-injected rats at -1, 3 and 7 days following viral injection (n=7~8/group). (C) The learning and memory ability in the passive avoidance test at 7 days after viral injection. The data shown are the means±S.E.M (n=7~8/group).
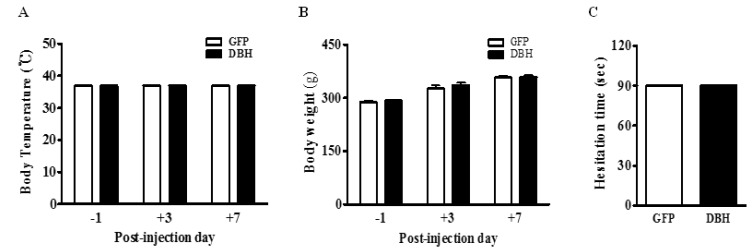