Abstract
In the present study, the anti-depressant like effect of methyl gallate (MG) isolated from the stem bark of Acer barbinerve was examined in ICR mice. Body weight (BDW) and blood glucose (BDG) levels significantly decreased in the repeated restraint stress (RRS) group (2 h/day for 14 days) compared to the no stress (NS) group. To examine the effect of MG on RS-induced BDW loss and hypoglycemia, MG (10 mg/kg) and the anti-depressant fluoxetine (10 mg/kg) were administered daily for 14 days. Orally administered MG and fluoxetine significantly attenuated the RS-induced BDW loss and hypoglycemia. Interestingly, MG administered mice showed increased BDG levels in the normal and glucose feeding condition. Chronic RS-subjected mice showed immobilized and depressed behaviors. The effect of MG on the depressed behaviors was evaluated using the tail-suspension test (TST) and the forced swimming test (FST). In both tests, RS-induced immobilized behaviors were significantly reversed in MG and fluoxetine administered groups. Taken together, MG significantly attenuated the RS-induced BDW loss, hypoglycemia, and depressed behaviors. Considering that decreased BDG levels (hypoglycemia) can cause depression, MG may exert its anti-depressant like effect by preventing hypoglycemia. Our results suggest that MG isolated from A. barbinerve can exert anti-depressant like effect, and could be used as a new and natural anti-depressant therapy.
Physical and psychological stresses are closely related to the onset of many diseases including metabolic syndrome, cardiovascular disease, and psychiatric disorders. One of the most prominent environmental risk factors is chronic exposure to stressful situations. Stress alters homeostasis in many body regions, including nervous, endocrine, immune, and digestive systems [1-3]. Chronic stress is a risk factor for psychiatric illnesses including anxiety and depression disorders. Depression is characterized by symptoms such as altered mood, cognitive impairment, and recurrent thoughts of death or suicide [4]. About two-third of depressed patients respond to the currently available treatments but the magnitude of improvement is still disappointing. Although there are many effective antidepressants available today, the current therapy is often inadequate with unsatisfactory results in about one-third of all patients treated. Thus, this necessitates the development of new and more effective antidepressants from traditional medicinal plants whose psychotherapeutic potential has been assessed in a variety of animal models. Chronic restraint stress (CRS) induce the depressive symptoms, such as decreased social interaction, decreased sucrose intake, increased immobility response in helpless conditions including tail-suspension and forced swimming [5-9]. Thus, in this study, we used a repeated RS (RRS; 2 hr/day for 14 days) mice model, which causes an increased immobility.
Methyl gallate (MG; Fig. 1A) is a derivative of gallic acid. Several lines of evidence indicate that MG possesses a several biological properties, such as anti-oxidant [10,11], anti-inflammatory [12], and anti-microbial properties [13,14]. MG also has a significant anti-tumor effect by inhibiting tumor infiltration of CD4+CD25+ regulatory T cells [15], and by inhibiting focal adhesion formation and Akt phosphorylation in glioma cells [16]. However, its biological activity has not been extensively examined in neurological disorders, especially depression. In the present study, we examined the possible anti-depressant like effect of MG isolated from the stem bark of Acer barbinerve in a repeated restraint stress (RRS) model. Here we demonstrate that MG has a significant inhibitory effect on RRS-induced BDW loss and hypoglycemia. Furthermore, MG significantly attenuated RRS-induced immobility or depressed behavior in tail-suspension test (TST) and the forced swimming test (FST). These results indicate that MG has an anti-depressant like effect in an animal model of depression.
The experiments were approved by the Dankook University Animal Care and Use Committee. The animals used in the present study were 20~24 g male ICR mice, supplied by MJ Co. (Seoul, Korea). Animals were provided a commercial diet and water ad libitum under controlled temperature (22±2℃), humidity (55±5%), and lighting conditions (12-h light/12-h dark cycle). The number of animals used and their suffering was minimized in all cases. Experiments were performed during the light phase of the cycle (10:00~17:00).
MG (Fig. 1A) was isolated from the stem bark of A. barbinerve. MG used in the present study was a kind gift from J.K. Kim (Gyeonggi Institute of Science & Technology Promotion, Suwon, Korea). A detailed isolation procedure has been described previously [16]. Briefly, the air-dried stem bark of A. barbinerve (3.5 kg) was extracted with 70% aqueous acetone by maceration. The extract was suspended in water and then successively partitioned with n-hexane, CH2Cl2, ethyl acetate (EtOAc), and water. The EtOAc extract (48 g) was subjected to column chromatography over a Sephadex LH-20 column eluted with methanol (MeOH)-H2O (3:1, v/v) to give 4 fractions (designated fractions 1~4). Fraction 2 was subjected to column chromatography on RP-18 silica gel with MeOH-H2O (1:1, 1:3, v/v), and then purified on Sephadex LH-20 with MeOH-H2O (1:2, 1:5, 1:7, v/v) and (EtOH)-hexane (3:1, 3:2, 5:4, v/v) to yield MG (13.7 g). MG was identified by a combination of spectroscopic methods including mass spectrometry (MS), 1H, and, 13C nuclear magnetic resonance (NMR).
RS treatment procedure has been described previously [8,17]. ICR mice were individually placed head-first into a well-ventilated 50 ml conical tube and plugged with a 3 cm long middle tube and finally the cap of the 50 ml tube. Mice were not able to move forward or backward in this device during 2 h. This RS was delivered to animals at set times daily from 10 AM. Control (no stress; NS) mice remained in their original cages and were left undisturbed in this home environment. After RS treatment, animals were returned to their normal home environments by housing them in pairs. This procedure was repeated for 14 days (2 h/day) unless otherwise indicated (Fig. 1B). Mice were fed with a diet supplemented for 14 days and during the behavioral test period with fluoxetine (10 mg/kg/day) and MG (10 mg/kg/day).
The BDG level was measured every 3 days after MG and fluoxetine administration during RS stimulation. A brief procedure has been described previously [18], the blood was collected shortly as much as possible with a minimum volume (1 microL) from the tail-vein. The glucose level was measured using Accu-Chek Performa blood glucose monitoring system (glucometer) (Mannheim, Baden-Württemberg, Germany).
After 16 h fasting, OGTT was performed. D-glucose solution (2 g/kg BDW) was administered orally, and the BDG level was measured from tail vein at 0, 30, 60, and 120 min after the initial glucose feeding. BDG level was determined using a glucometer.
Mice were suspended individually by their tail from a metal rod. The rod was fixed 50 cm above the surface of a table covered with soft cloth in a sound-isolated room. The tip of the tail was fixed using adhesive Scotch tape; the duration of the test was 6 min. The immobility time was determined by an observer, using a stopwatch, who was unaware of the strain [8,17].
Mice were placed in a Plexiglas cylinder (height: 27 cm, diameter: 15 cm) containing water at 24℃ and a depth of 14 cm so that they could neither escape nor touch the bottom. Mice were subjected to a pre-swim (15 min). After pre-testing, the animals were dried quickly with a towel and returned to home cages. One day later, mice were forced to swim for 6 min. The animals were habituated for the first 1 min and behavior was noted over the next 5 min. Immobility time was defined as the total time during which animals remained floating with all limbs motionless. This scoring method has been described previously [8,17].
All values shown in the figures are expressed as the mean±SEM obtained from at least three independent experiments. Statistical analysis was carried out by Student t test (Fig. 2A, 3A, 3C, 4) and one-way analysis of variance (ANOVA) with Tukey's post-hoc test (Fig. 2B, 3B, 5B, 5C) using GraphPad Prism (GraphPad Software, San Diego, CA, USA). Statistically significant differences between groups were assumed when p<0.05.
As shown in Fig. 1B, ICR mice were acclimated to the animal behavior test room during 1 wk, and then were subjected to RS (2 h/day for 2 wks) in the same room. BDW was measured daily for all mice before RS treatment. We observed that BDW decreased significantly in the RS-subjected group compared to NS group (Fig. 2A). To examine the effect of MG on RS-induced BDW loss, MG (10 mg/kg) and the anti-depressant fluoxetine (10 mg/kg) were administered daily for 14 days. ICR mice orally administered MG as well as fluoxetine showed a significant reverse in BDW on day 14 (Fig. 2B).
In addition to BDW, BDG levels were measured daily for all mice before RS treatment. We observed that hypoglycemia occurred frequently in the chronic RS group (Fig. 3A). To examine the effect of MG on RS-induced hypoglycemia, MG (10 mg/kg) and fluoxetine (10 mg/kg) were administered daily for 14 days. And then we measured BDG levels on day 14. Oral administration of MG and fluoxetine significantly reversed the RS-induced hypoglycemia (Fig. 3B). To confirm the recovering effect of MG on hypoglycemia, MG (10 mg/kg) was administered daily for up to 21 days in both NS and RS groups. BDG levels were measured every 3 days before the RS condition began. The MG-induced reversal of hypoglycemia was evident within 3 days and was maintained over the duration of MG treatment (21 days) (Fig. 3C).
Interestingly, mice treated with MG alone showed significantly elevated BDG levels compared to the NS group (Fig. 3B). To further examine the hyperglycemic effect of MG, we performed OGTT after orally pre-treatment with MG (10 and 20 mg/kg). The BDG levels were measured 30, 60, and 120 min after D-glucose feeding. As shown in Fig. 4, orally pre-treatment with MG (10 and 20 mg/kg) itself did not affect BDG levels as compared to the vehicle treated group. However, MG (20 mg/kg) further increased the elevated BDG level 30 min after glucose feeding. This result indicates that MG attenuated the glucose elimination rate.
The chronic RS model demonstrates depressive behaviors and is an appropriate animal model of depression. As shown in Fig. 5A, ICR mice were subjected to RS (2 h/day for 2 wks) in the animal behavior test room. Mice were administered daily MG (10 mg/kg) and fluoxetine (10 mg/kg) during the 14 days. Behavioral tests were performed on day 14, and were examined first for the TST and then for the FST. In the TST, the immobility time was increased in the chronic RS group compared to the NS group. Both MG and fluoxetine attenuated the total immobilized time to the normal levels (Fig. 5B). In addition, chronic RS significantly induced immobilized behavior responses in the FST. As with the TST, both MG and fluoxetine decreased the total immobilized time to normal levels (Fig. 5C).
The present study investigated the anti-depressant like effects of MG isolated from A. barbinerve in a RRS mice model. MG significantly attenuated BDW loss, hypoglycemia, immobility induced by RRS in mice. These findings indicate a remarkable anti-depressant like effect for MG. Mice or rats exposed to stressful situations over long periods show behavioral changes that mimic depression, such as decreased social interaction, decreased sucrose intake, and increased immobility and responses in helpless conditions including TST and FST [5-9]. Thus, in the present study, we used a RRS (2 h/day for 14 days) mice model, which causes an increased immobility (or depressed behavior).
In general, chronic stress induces a BDW loss, which serves as a physiologic marker of stress. RRS mice showed a decreased BDW compared to NS mice. Reduced BDW was significantly reversed by oral MG and fluoxetine administration. Since daily food and water intake by RS group was similar to that of NS group (data not shown), the reduced BDW did not result from a difference in food or water intake. In line with our previous results, mice exposed to daily restraint weighed less than the NS group over time [7]. This result indicates that the lowered BDW induced by RRS may result from an altered energy metabolism, such as glucose metabolism.
A large body of evidence shows that different types of stress, including oxidative, ER, and restraint stress, influence glucose metabolism [19-24]. Thus, the present study employed a 2-h RS for 14 days to measure its effects on BDG level. Our findings showed that RS led to hypoglycemia in mice (Fig. 3), which was reflected by abnormal glucose metabolism. MG as well as fluoxetine administration attenuated the RS-induced hypoglycemia. Interestingly, MG increased BDG level in the NS group and also slightly inhibited glucose elimination rate in OGTT (Fig. 4). Thus, the recovery effect of MG on BDG level may result from MG activating BDG level and glucose tolerance. In contrast, previous results showed that RS induced hyperglycemia [22,25-27]. Different measurement times for BDG level may result in different findings, such as hyperglycemia and hypoglycemia. Most of studies measured BDG level during the RS-exposed time, whereas this study measured BDG level 24 h after RS termination. Chronic stress induced expression of genes related to glucose metabolism including G6PC, GK, GLUT2, and GLUT8 [23,28,29]. In addition, contents of muscle and hepatic glycogen were reduced in RS mice [23]. Taken together, chronic stress eventually influenced glucose metabolic enzyme activities in mice, which in turn altered BDG level, such as hypoglycemia or hyperglycemia.
Hypoglycemia causes depressive symptoms in mice [24]. In addition, recent reports showed that there is a connection between depression and hypoglycemia in humans [30,31]. Our results also showed that RRS causes hypoglycemia and depressive behavior (immobility). MG and fluoxetine attenuated repeated RS-induced immobility (Fig. 5). These findings indicate that MG exerts an anti-depressant like effect in RRS mice. In this study, fluoxetine, an anti-depressant of the selective serotonin reuptake inhibitor (SSRI) serves as a positive control to evaluate the anti-depressant like effect of MG in every experiment. Fluoxetine also attenuated the RS-induced BDW loss, hypoglycemia, and depressed behaviors. In line with our results, fluoxetine prevented hypoglycemia-induced immobility in the FST [24]. In this sense, MG may inhibit serotonin reuptake as an SSRI. Taken together, MG attenuates hypoglycemia, which in turn attenuates BDW loss and depressed behaviors under RRS. However, further studies are necessary to confirm and extend these findings in RRS model.
In conclusion, MG significantly attenuated the RS-induced BDW loss, hypoglycemia, and depressed behaviors in the present study. Our results suggest that MG isolated from A. barbinerve exerts an anti-depressant like effect and possible attenuated hypoglycemia. MG may be developed as a new and natural anti-depressant therapy.
ACKNOWLEDGEMENTS
This research was supported by Basic Science Research Program through the National Research Foundation of Korea (NRF) funded by the Ministry of Education (2011-0012972).
References
1. Alkadhi KA. Chronic psychosocial stress exposes Alzheimer's disease phenotype in a novel at-risk model. Front Biosci (Elite Ed). 2012; 4:214–229. PMID: 22201866.


2. Cyr NE, Earle K, Tam C, Romero LM. The effect of chronic psychological stress on corticosterone, plasma metabolites, and immune responsiveness in European starlings. Gen Comp Endocrinol. 2007; 154:59–66. PMID: 17681504.


3. Schmidt MV, Sterlemann V, Müller MB. Chronic stress and individual vulnerability. Ann N Y Acad Sci. 2008; 1148:174–183. PMID: 19120107.


4. Pittenger C, Duman RS. Stress, depression, and neuroplasticity: a convergence of mechanisms. Neuropsychopharmacology. 2008; 33:88–109. PMID: 17851537.


5. Berton O, McClung CA, Dileone RJ, Krishnan V, Renthal W, Russo SJ, Graham D, Tsankova NM, Bolanos CA, Rios M, Monteggia LM, Self DW, Nestler EJ. Essential role of BDNF in the mesolimbic dopamine pathway in social defeat stress. Science. 2006; 311:864–868. PMID: 16469931.


6. Greene J, Banasr M, Lee B, Warner-Schmidt J, Duman RS. Vascular endothelial growth factor signaling is required for the behavioral actions of antidepressant treatment: pharmacological and cellular characterization. Neuropsychopharmacology. 2009; 34:2459–2468. PMID: 19553916.


7. Kim KS, Han PL. Optimization of chronic stress paradigms using anxiety- and depression-like behavioral parameters. J Neurosci Res. 2006; 83:497–507. PMID: 16416425.


8. Kim KS, Kwon HJ, Baek IS, Han PL. Repeated short-term (2 h×14 d) emotional stress induces lasting depression-like behavior in mice. Exp Neurobiol. 2012; 21:16–22. PMID: 22438675.
9. Willner P. Chronic mild stress (CMS) revisited: consistency and behavioural-neurobiological concordance in the effects of CMS. Neuropsychobiology. 2005; 52:90–110. PMID: 16037678.


10. Crispo JA, Piché M, Ansell DR, Eibl JK, Tai IT, Kumar A, Ross GM, Tai TC. Protective effects of methyl gallate on H2O2-induced apoptosis in PC12 cells. Biochem Biophys Res Commun. 2010; 393:773–778. PMID: 20171161.


11. Hsieh TJ, Liu TZ, Chia YC, Chern CL, Lu FJ, Chuang MC, Mau SY, Chen SH, Syu YH, Chen CH. Protective effect of methyl gallate from Toona sinensis (Meliaceae) against hydrogen peroxide-induced oxidative stress and DNA damage in MDCK cells. Food Chem Toxicol. 2004; 42:843–850. PMID: 15046831.


12. Kang MS, Jang HS, Oh JS, Yang KH, Choi NK, Lim HS, Kim SM. Effects of methyl gallate and gallic acid on the production of inflammatory mediators interleukin-6 and interleukin-8 by oral epithelial cells stimulated with Fusobacterium nucleatum. J Microbiol. 2009; 47:760–767. PMID: 20127471.


13. Choi JG, Kang OH, Lee YS, Oh YC, Chae HS, Jang HJ, Shin DW, Kwon DY. Antibacterial activity of methyl gallate isolated from Galla Rhois or carvacrol combined with nalidixic acid against nalidixic acid resistant bacteria. Molecules. 2009; 14:1773–1780. PMID: 19471197.


14. Kang MS, Oh JS, Kang IC, Hong SJ, Choi CH. Inhibitory effect of methyl gallate and gallic acid on oral bacteria. J Microbiol. 2008; 46:744–750. PMID: 19107406.


15. Lee H, Lee H, Kwon Y, Lee JH, Kim J, Shin MK, Kim SH, Bae H. Methyl gallate exhibits potent antitumor activities by inhibiting tumor infiltration of CD4+CD25+ regulatory T cells. J Immunol. 2010; 185:6698–6705. PMID: 21048105.
16. Lee SH, Kim JK, Kim DW, Hwang HS, Eum WS, Park J, Han KH, Oh JS, Choi SY. Antitumor activity of methyl gallate by inhibition of focal adhesion formation and Akt phosphorylation in glioma cells. Biochim Biophys Acta. 2013; 1830:4017–4029. PMID: 23562553.


17. Park SH, Sim YB, Han PL, Lee JK, Suh HW. Antidepressant-like effect of kaempferol and quercitirin, isolated from Opuntia ficus-indica var. saboten. Exp Neurobiol. 2010; 19:30–38. PMID: 22110339.


18. Sim YB, Park SH, Kang YJ, Kim SS, Kim CH, Kim SJ, Jung JS, Ryu OH, Choi MG, Choi SS, Suh HW. Effect of cholera toxin administered supraspinally or spinally on the blood glucose level in pain and d-glucose fed animal models. Korean J Physiol Pharmacol. 2013; 17:163–167. PMID: 23626479.


19. Pedersen WA, Culmsee C, Ziegler D, Herman JP, Mattson MP. Aberrant stress response associated with severe hypoglycemia in a transgenic mouse model of Alzheimer's disease. J Mol Neurosci. 1999; 13:159–165. PMID: 10691302.


20. Zhou J, Shi MX, Mitchell TD, Smagin GN, Thomas SR, Ryan DH, Harris RB. Changes in rat adipocyte and liver glucose metabolism following repeated restraint stress. Exp Biol Med (Maywood). 2001; 226:312–319. PMID: 11368423.


21. Ozcan U, Yilmaz E, Ozcan L, Furuhashi M, Vaillancourt E, Smith RO, Görgün CZ, Hotamisligil GS. Chemical chaperones reduce ER stress and restore glucose homeostasis in a mouse model of type 2 diabetes. Science. 2006; 313:1137–1140. PMID: 16931765.


22. Sim YB, Park SH, Kang YJ, Kim SM, Lee JK, Jung JS, Suh HW. The regulation of blood glucose level in physical and emotional stress models: possible involvement of adrenergic and glucocorticoid systems. Arch Pharm Res. 2010; 33:1679–1683. PMID: 21052944.


23. Tsoi B, He RR, Yang DH, Li YF, Li XD, Li WX, Abe K, Kurihara H. Carnosine ameliorates stress-induced glucose metabolism disorder in restrained mice. J Pharmacol Sci. 2011; 117:223–229. PMID: 22123261.


24. Park MJ, Yoo SW, Choe BS, Dantzer R, Freund GG. Acute hypoglycemia causes depressive-like behaviors in mice. Metabolism. 2012; 61:229–236. PMID: 21820138.


25. Ribeiro-de-Oliveira A, Guerra RM, Fóscolo RB, Marubayashi U, Reis AM, Coimbra CC. Bromocriptine-induced dissociation of hyperglycemia and prolactin response to restraint. Pharmacol Biochem Behav. 2001; 68:229–233. PMID: 11267627.


26. Bates HE, Kiraly MA, Yue JT, Goche Montes D, Elliott ME, Riddell MC, Matthews SG, Vranic M. Recurrent intermittent restraint delays fed and fasting hyperglycemia and improves glucose return to baseline levels during glucose tolerance tests in the zucker diabetic fatty rat--role of food intake and corticosterone. Metabolism. 2007; 56:1065–1075. PMID: 17618951.


27. Kainuma E, Watanabe M, Tomiyama-Miyaji C, Inoue M, Kuwano Y, Ren H, Abo T. Association of glucocorticoid with stress-induced modulation of body temperature, blood glucose and innate immunity. Psychoneuroendocrinology. 2009; 34:1459–1468. PMID: 19493627.


28. Piroli GG, Grillo CA, Charron MJ, McEwen BS, Reagan LP. Biphasic effects of stress upon GLUT8 glucose transporter expression and trafficking in the diabetic rat hippocampus. Brain Res. 2004; 1006:28–35. PMID: 15047021.


29. Lee CY. Chronic restraint stress induces intestinal inflammation and alters the expression of hexose and lipid transporters. Clin Exp Pharmacol Physiol. 2013; 40:385–391. PMID: 23586523.


30. Katon WJ, Young BA, Russo J, Lin EH, Ciechanowski P, Ludman EJ, Von Korff MR. Association of depression with increased risk of severe hypoglycemic episodes in patients with diabetes. Ann Fam Med. 2013; 11:245–250. PMID: 23690324.


31. Korczak DJ, Pereira S, Koulajian K, Matejcek A, Giacca A. Type 1 diabetes mellitus and major depressive disorder: evidence for a biological link. Diabetologia. 2011; 54:2483–2493. PMID: 21789690.


Fig. 1
Structure of methyl gallate and experimental design. (A) Structure of methyl gallate (MG). (B) Experimental design. Mice were subjected to RS (2 h/day for 14 days). Mice were orally fed with MG (10 mg/kg) and fluoxetine (10 mg/kg) daily up to 14 days. Body weight (BDW) and blood glucose (BDG) levels were measured on day before RS exposure at every day or every 3 days.

Fig. 2
Effect of MG on RS-induced BDW loss. (A) BDW was measured every 3 days in NS and RS (2 h/day for 14 days) groups. (B) Mice were orally fed with MG (10 mg/kg) and fluoxetine (10 mg/kg) daily up to 14 days. BDW was measured on day 14 before RS exposure. Values are mean±SEM (A: ***p<0.001; compared to each NS group, n=12, B: **p<0.01; compared to NS group, +p<0.05; RS+VHC vs RS+fluoxetine, RS+VHC vs RS+MG, n=8).
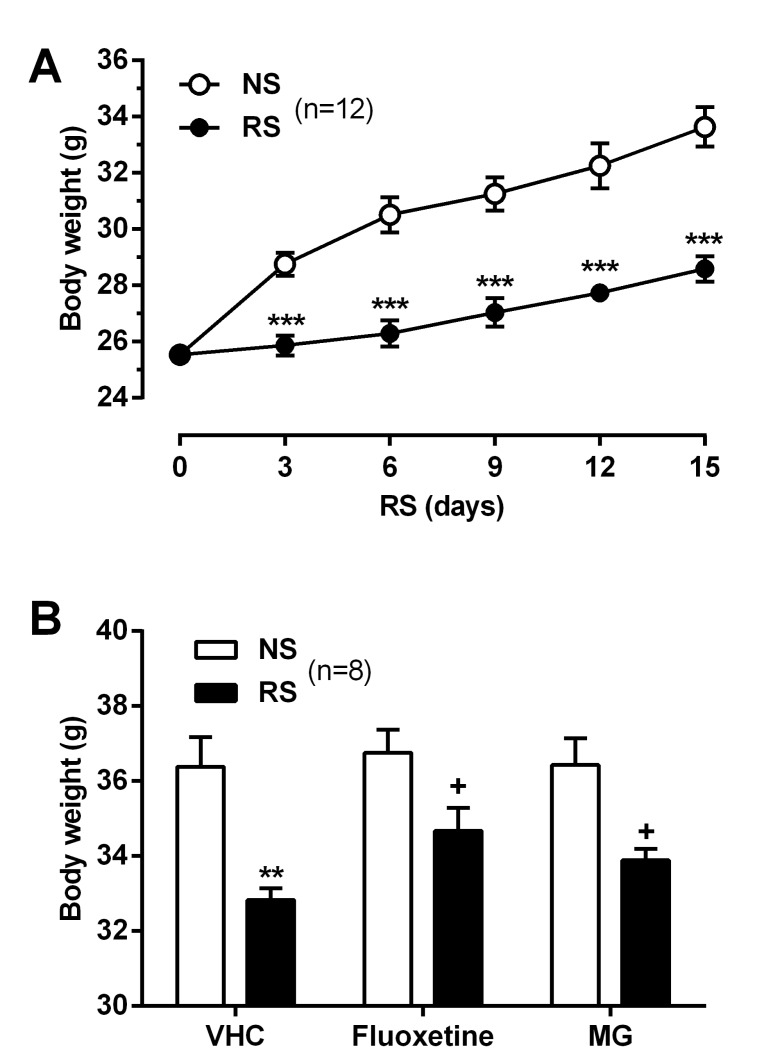
Fig. 3
Effect of MG on RS-induced hypoglycemia. (A) BDG level was measured every 3 days in NS and RS (2 h/day for 14 days) groups. (B) Mice were orally fed with MG (10 mg/kg) and fluoxetine (10 mg/kg) daily up to 14 days. BDG level was measured on day 14 before RS exposure. (C) Mice were orally fed with MG (10 mg/kg) daily up to 21 days. BDG level was measured on day before RS exposure every 3 days. Values are mean±SEM (A: ***p<0.001; compared to each NS group, n=14, B: *p<0.05, **p<0.01; compared to NS group, +p<0.05; RS-VHC vs RS-fluoxetine, RS-VHC vs RS-MG, n=7, C: **p<0.01; compared to each NS group, +p<0.05; RS+VHC vs RS+MG, n=6).
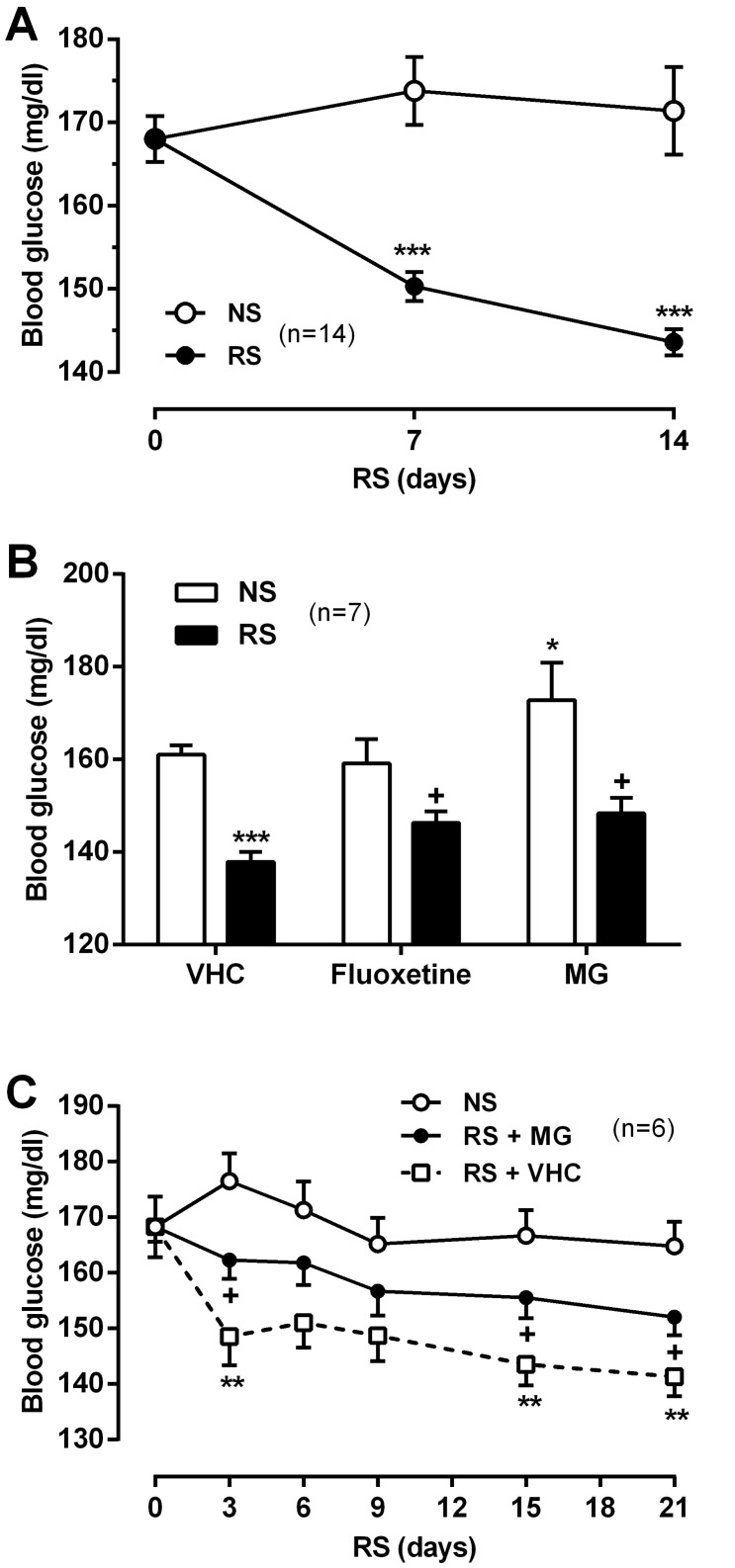
Fig. 4
Effect of MG on BDG level in OGTT. Mice were administered orally with D-glucose (2 g/kg) at 30 min after orally pretreatment with MG (10 and 20 mg/kg). The BDG level was measured at 30, 60, and 120 min after D-glucose feeding. Values are mean±SEM (*p<0.05; compared to VHC+D-Glu group, n=5).
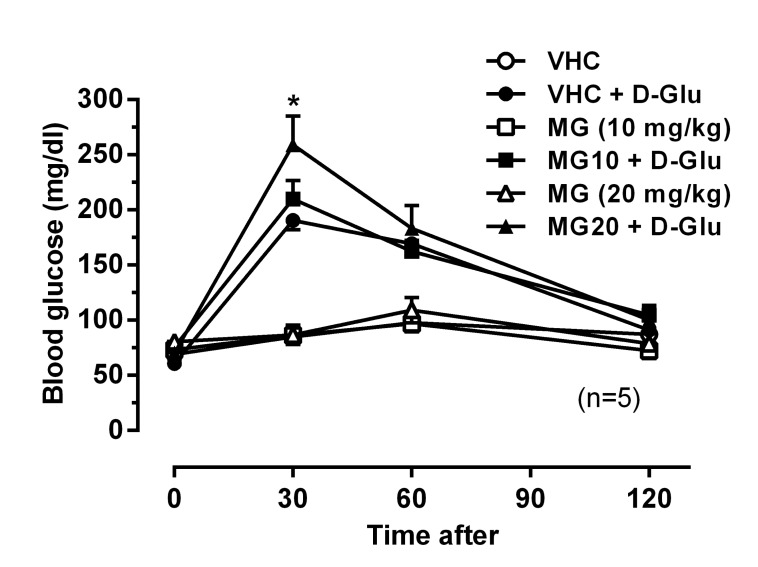
Fig. 5
Effect of MG on RS-induced depressive behaviors. (A) Experimental design for behavioral analyses. Mice were subjected to RS (2 h/day for 14 days). Mice were orally treated with MG (10 mg/kg) and fluoxetine (10 mg/kg) daily for up to 14 days. Total immobilized time was measured on day 14 after final RS exposure using tail-suspension test (TST) (B) and forced swimming test (FST) (C). Values are mean±SEM (B and C: *p<0.05, ***p<0.001; compared to NS group, +p<0.05, ++p<0.01, +++p<0.001; RS-VHC vs RS-fluoxetine, RS-VHC vs RS-MG, n=10).
