Abstract
The calcium-activated K+ (BKCa) channel is one of the potassium-selective ion channels that are present in the nervous and vascular systems. Ca2+ is the main regulator of BKCa channel activation. The BKCa channel contains two high affinity Ca2+ binding sites, namely, regulators of K+ conductance, RCK1 and the Ca2+ bowl. Lysophosphatidic acid (LPA, 1-radyl-2-hydroxy-sn-glycero-3-phosphate) is one of the neurolipids. LPA affects diverse cellular functions on many cell types through G protein-coupled LPA receptor subtypes. The activation of LPA receptors induces transient elevation of intracellular Ca2+ levels through diverse G proteins such as Gαq/11, Gαi, Gα12/13, and Gαs and the related signal transduction pathway. In the present study, we examined LPA effects on BKCa channel activity expressed in Xenopus oocytes, which are known to endogenously express the LPA receptor. Treatment with LPA induced a large outward current in a reversible and concentration-dependent manner. However, repeated treatment with LPA induced a rapid desensitization, and the LPA receptor antagonist Ki16425 blocked LPA action. LPA-mediated BKCa channel activation was also attenuated by the PLC inhibitor U-73122, IP3 inhibitor 2-APB, Ca2+ chelator BAPTA, or PKC inhibitor calphostin. In addition, mutations in RCK1 and RCK2 also attenuated LPA-mediated BKCa channel activation. The present study indicates that LPA-mediated activation of the BKCa channel is achieved through the PLC, IP3, Ca2+, and PKC pathway and that LPA-mediated activation of the BKCa channel could be one of the biological effects of LPA in the nervous and vascular systems.
Calcium-activated K+ (BKCa) channels are high-conductance, voltage-dependent, and potassium-selective ion channels. BKCa channels are activated by depolarization and elevation of intracellular Ca2+ levels and causes a closure of voltage-dependent Ca2+ channels and reduces Ca2+ influx into the cell. Thus, BKCa channels are dually regulated through membrane voltage and intracellular Ca2+. BKCa channels comprise two subunits, α and β [1-3]. Ca2+-dependent kinases also can phosphorylate the α subunit and regulate the channel activity [4]. BKCa channels participate in diverse physiological processes such as neuronal excitability, smooth muscle contractility, hair cell tuning, and have a protective mechanism against ischemic cell death of neurons [5]. In addition, there are two regulators of K+ conductance, the RCK1 and RCK2 domains in the cytosolic domain of the BKCa channel [6]. These contain two high affinity Ca2+ binding sites. One is in the RCK1 domain at position Asp362/367 and the other is in the Ca2+ bowl that contains a series of Asps located in the RCK2 domain [7,8] (Fig. 1A).
Lysophosphatidic acid (LPA, 1-radyl-2-hydroxy-sn-glycero-3-phosphate) is the prototypical member of the family of lipid mediators [9] (Fig. 1B). The endothelial gene (EDG) family encodes for the GPCR-specific LPA and S1P [9]. LPA activates diverse cellular actions through interactions with the G protein-coupled LPA receptors, which are responsible for the transient elevation of intracellular Ca2+ levels [9-11]. In addition, it was demonstrated that neurite retraction and cell rounding in cell lines of neural origin are caused by LPA [12]. Applying LPA extracellularly induces cell polarization, directional motility, and metabolic burst in human neutrophils [13]. However, relatively little is known about how LPA regulates ion channel activity, especially in Ca2+-dependent ion channels.
In the present study, we examined the effects of LPA on BKCa (rat brain rSlo) channel activities expressed in Xenopus laevis oocytes by using the two-electrode voltage clamp technique. We found that LPA activates the BKCa channel in a concentration- and voltage-dependent manner. Moreover, when we treated the cells with LPA repeatedly, we could observe the desensitization of LPA-mediated BKCa channel activation. By using various inhibitors of phospholipase C, IP3 receptor, and protein kinase C, we examined the signaling pathway in LPA-mediated BKCa channel activation. In addition, the effects of site-directed mutagenesis on RCK1 and mutations in the Ca2+ bowl in LPAmediated BKCa channel activation were examined. Finally, we have discussed the physiological or pharmacological roles of LPA in the nervous and vascular systems.
We used previously reported cDNA for the rat brain BKCa channel (Gene bank ID: NM_000218) [14]. Lysophosphatidic acid (LPA, 1-oleoyl-2-hydroxy-sn-glycero-3-phosphate, 18:1) was purchased from Avanti Polar Lipids, Inc (Alabaster, Al, USA). LPA was dissolved in dimethyl sulfoxide (DMSO) and stored at -20℃ as a stock. All other reagents were purchased from Sigma-Aldrich (St. Louis, MO, USA).
Xenopus laevis frogs were purchased from Xenopus I (Ann Arbor, MI, USA). All procedures for their care and handling were approved by the Konkuk University Institutional Animal Care and Use Committee. To isolate oocytes, frogs were anesthetized with an aerated solution of 3-aminobenzoic acid ethyl ester. Ovary was removed and frogs are sacrificed under anesthetized. Oocytes were separated by treatment with collagenase and agitated for 2 h in a CaCl2-free medium containing 82.5 mM NaCl, 2 mM KCl, 1 mM MgCl2, 5 mM N-(2-hydroxyethyl)piperazine-N'-2 ethanesulfonic acid (HEPES), 2.5 mM sodium pyruvate, 100 units penicillin/ml, and 100 µg streptomycin/ml. Stage V~VI oocytes were collected and stored in ND96 medium (96 mM NaCl, 2 mM KCl, 1 mM MgCl2, 1.8 mM CaCl2, and 5 mM HEPES, pH 7.5) supplemented with 50 µg gentamycin/ml [15]. The oocyte-containing solution was maintained at 18℃ with continuous gentle shaking and daily medium changes [16]. All electrophysiological experiments were performed within 3 to 4 days following isolation of the oocytes.
BKCa channel-encoding cRNAs were prepared using an mMessage mMachine transcription kit (Ambion, Austin, TX, USA) with T7 RNA polymerase. The cRNAs were injected into the animal or vegetal pole of each oocyte one day after isolation. The cRNA was dissolved in RNase-free water at a final concentration of approximately 1 µg/µl, divided into aliquots, and stored at -80℃ until further use. BKCa channel cRNAs (40 nl) were injected into the animal or vegetal pole of each oocyte using a 10 µl VWR microdispenser (VWR Scientific, West Chester, PA, USA). The injection pipette was made by pulling from glass capillary tubing used for recording electrodes and breaking the tip into a 15~20 µm diameter [16].
Single amino acid substitutions of the BKCa channel (Fig. 1B) were made using a QuikChange™ XL Site-Directed Mutagenesis Kit (Stratagene, La Jolla, CA, USA), Pfu DNA polymerase, and sense and antisense primers encoding the desired mutations. Overlap extension of the target domain by sequential polymerase chain reaction (PCR) was carried out according to the manufacturer's protocol. The final PCR products were transformed into E. coli strain DH5α, screened by PCR, and confirmed by sequencing of the target regions. The mutant DNA constructs were linearized at their 3' ends by digestion with NotI, and run-off transcripts were prepared using the methylated cap analog, m7G(5')-ppp(5')G. The absence of degraded RNA was confirmed by denaturing agarose gel electrophoresis followed by ethidium bromide staining. Similarly, recombinant plasmids containing rat BKCa channel cDNA inserts were linearized by digestion with the appropriate restriction enzymes, and cRNAs were obtained using the mMessage mMachine in vitro transcription kit (Ambion, Austin, TX, USA) with SP6 RNA polymerase or T7 polymerase. The final cRNA products were resuspended at a concentration of 1 µg/µl in RNase-free water and stored at -80℃ [16].
Data recording for BKCa channel currents was performed as described by [17]. A custom-made Plexiglas net chamber was used for two-electrode voltage-clamp recordings as previously reported. The oocytes were impaled with two microelectrodes filled with 3M KCl (0.2~0.7 MΩ), and electrophysiological experiments were carried out at room temperature using an Oocyte Clamp (OC-725C, Warner Instruments, Hamsden, CT, USA). Stimulation and data acquisition were controlled with a pClamp 8 (Axon Instruments, Union City, CA, USA). For most electrophysiological experiments, oocytes were perfused initially with Cl-- and Ca2+-free solution (in mM: 96 NaOH, 2 KOH, 8 Mg-gluconate, 5 HEPES, 5 EGTA, pH 7.4 with methanesulfonic acid) in the presence of a Cl- channel blocker (500 µM anthracene-9-carboxylic acid) [17] to inhibit endogenous Cl- channels. The oocytes were then clamped at a holding potential of -80 mV, membrane potential was depolarized to +40 mV for 400 ms at 10 s intervals, and currents were recorded as otherwise indicated.
The pH was adjusted to 7.0 with 2-[N-morpholino]ethanesulfonic acid. To compare the channel characteristics accurately, an identical set of intracellular solutions was used throughout the experiments. Commercial software packages, which included Clampex 8.0 or 8.1 (Axon Instruments) and Origin 6.1 (OriginLab Corp., Northampton, MA, USA), were used for the acquisition and analysis of macroscopic recording data.
To obtain the concentration-response curve for the LPA effects on the K+ currents from the BKCa channel, the peak amplitudes at different concentrations of LPA were plotted. The current enhancements or inhibitions evoked by drug treatment were analyzed after subtraction of currents elicited by H2O injection. Origin software (Origin, Northampton, MA, USA) was used to fit the plot to the Hill equation: y/ymax=[A]nH/([A]nH+[EC50]nH), where y represents the peak current at a given concentration of LPA, ymax is the maximal peak current, EC50 is the concentration of LPA producing a half-maximal effect, [A] is the concentration of LPA, and nH is the Hill coefficient. All values are represented as mean±S.E.M. Significant differences between mean control and treatment values were determined using the Student's t-test, where p<0.05 was considered statistically significant.
Treatment of LPA in oocytes injected with cRNA encoding the BKCa α subunit elicited a large outward current with a voltage step from -80 to +40 mV with a 400 ms duration at 10-s intervals (Fig. 2A). We next examined the effects of various concentrations of LPA on the BKCa channel activity. As shown in Fig. 2, the application of LPA on oocytes induced BKCa channel activation in a concentration-dependent manner. Charybdotoxin and iberiotoxin, highly specific inhibitor of maxi-K channels [18,19], greatly attenuated the BKCa channel activations induced by LPA (data not shown), indicating that BKCa channels are functionally working [20]. Oocytes injected with only H2O elicited a basal outward current (data not shown). LPA activated the BKCa channel by an average of 761.42±124.10% at 1 µM. The EC50 was 0.12±0.05 µM (Fig. 2Ab). These results indicate that LPA induces BKCa channel activation.
The effect of LPA on the current-voltage (I~V) relationship was examined. The current response evoked by each voltage step (i.e., a series of voltage pulses of 400 ms duration given in 10 mV increments and 3-s intervals from the holding potential of -80 mV) was used to obtain the I~V curve. In the absence of LPA, voltage pulses greater than 0 mV exhibited slight BKCa channel currents (Fig. 2Ba, upper traces). The presence of LPA increased the current amplitude over 0 mV compared to the absence of LPA (Fig. 2Ba, lower traces), indicating that LPA activates the BKCa channel in a voltage-dependent manner (Fig. 2Bb).
We examined the possible changes caused by LPA on BKCa channel currents after repeated applications of LPA. As shown in Fig. 3A, treatment with 100 nM LPA for 1 min enhanced the BKCa channel current. Oocytes were then washed with recording buffer for 3 min. When the outward current returned to the basal level, oocytes were re-treated with LPA. The second, third, and fourth BKCa current responses for LPA were significantly diminished. The BKCa currents diminished as follows: 410±25, 137±25, 34.6±10.3, 2±1.25%, for the first, second, third, and forth response, respectively (n=5, oocytes from two different batches of donors), indicating that repeated treatment of LPA desensitized BKCa channel activation.
Since the previous report showed that Xenopus oocytes express the endogenous LPA1 receptor [21], we examined whether the LPA receptor 1/3 antagonist Ki16425 could inhibit LPA-induced BKCa channel activation. Oocytes were first incubated with 10 µM Ki16425 for 10 min. In the absence of Ki16425, LPA (100 nM) activated the BKCa channel by 350.52±21.91%; however, the presence of Ki16425 LPA (100 nM) activated the BKCa channel by only 12.50±2.53% (Fig. 3B). Thus, LPA activates the BKCa channel through LPA receptor activation.
We next investigated the signal transduction pathway for LPA-mediated activation of BKCa channels. First, we used an active PLC inhibitor, U-73122, and an inactive PLC inhibitor, U-73343 (Fig. 4A). Oocytes expressing the BKCa channel were incubated with U-73122 (1 µM) or U-73343 (1 µM) for 20 min. Treatment of LPA with U-73122 significantly attenuated the current from 332.50±26.41 to 41.43±32.95%. However, treatment of LPA with U-73343 did not show a significant difference (332.50±26.41 to 315.00±51.05%). These results demonstrate that the activation of the BKCa channel by LPA is also mediated by the PLC pathway.
To study the relationship between the inositol 1,4,5-trisphosphate receptor and BKCa channel activation by LPA, we used 2-aminoethoxydiphenyl borate (2-APB), which blocks the inositol 1,4,5-trisphosphate (IP3) receptor. The oocytes expressing the BKCa channel were pre-incubated with 100 µM 2-APB for 20 min before recording. In the absence of 2-APB, LPA (100 nM) activated the BKCa channel by 312.63±38.01%, whereas in the presence of 2-APB LPA (1 µM), the BKCa channel was activated by only 81.58±13.62% (Fig. 4B). Thus, LPA-mediated activation of the BKCa channel involves the IP3 receptor.
Next, we used BAPTA-AM (1,2-bis(O-aminophenoxy) ethane-N,N,N',N'-tetraacetic acid), a cell permeable intracellular Ca2+ chelator, to discover whether LPA-mediated activation of the BKCa channel was from elevated levels of free cytosolic Ca2+. As shown in Fig. 4C, pretreatment with BAPTA-AM (100 µM, 3 h) on oocytes significantly attenuated LPA-mediated BKCa channel activation. Thus, the LPA-mediated BKCa channel current after BAPTA-AM incubation was abolished. Therefore, LPA-mediated enhancement of BKCa channel currents is dependent on intracellular Ca2+.
We also investigated the involvement of the PKC pathway in BKCa channel activation by LPA. Oocytes expressing the BKCa channel were incubated with 1.5 µM calphostin, an inhibitor of PKC, for 2 h. Calphostin also significantly attenuated LPA-mediated BKCa channel activation (Fig. 5A). Thus, LPA-mediated BKCa channel activation includes the phospholipase C-IP3 receptor-Ca2+-protein kinase C pathways.
The BKCa channel contains two regulators of K+ conductance (RCK) domains in the cytoplasmic domain [3]. We constructed mutant BKCa channels in the RCK1 domain to decipher whether BKCa channel activation by LPA involves the RCK1 domain. For this, mutant BKCa channels with two RCK1s such as M579I and D433A were prepared [22]. In wild-type, LPA (100 nM)-mediated BKCa channel activation was 316.5±75.10%. However, in the mutant M579I channel, LPA-mediated BKCa channel activation was significantly decreased by 30.5±14.12% (Fig. 5Ba). In the mutant D433A channel, LPA-mediated (100 nM) BKCa channel activation was also significantly decreased by 77.5±38.62% (Fig. 5Ba). The EC50 values for the RCK1 mutants were 121.8±43.0, 134.3±7.9, and 177.2±16.3 nM for wild type, D433A, and M579I, respectively (Fig. 5Ba).
In addition, we constructed six mutant BKCa channels at the Ca2+ bowl, such as D989A, D991A, D992A, D993A, D994A, and D995A [23]. The percentages of activation from 100 nM LPA were 317.50±60.90, 23.60±2.73, 41.20±5.49, 49.16±10.13, 28.14±6.16, 7.05±2.12, and 49.30±16.19% for wild-type, D989A, D991A, D992A, D993A, D994A, and D995A, respectively (n=9). In addition, the EC50 values were 121.80±42.96, 334.22±13.20, 147.54±34.34, 434.53±120.27, 404.29±73.91, 411.30±76.58, and 183.46±3.23 nM for wild-type, D989A, D991A, D992A, D993A, D994A, and D995A, respectively (Fig. 5Bb). These results showed that RCK1 and the Ca2+ bowl are involved in LPA-mediated BKCa channel activation.
In the present study, we investigated the signal couplings of LPA on BKCa channel activation by using a Xenopus oocyte gene expression system. Our results revealed three major findings. First, we observed that LPA treatment induced BKCa channel activation in concentration- and voltage-dependent manners via LPA receptor activation, while repeated treatments with LPA induced rapid desensitization. Second, the presence of PLC inhibitor, IP3 receptor antagonist, intracellular Ca2+ chelator, or PKC inhibitor greatly attenuated the LPA actions. Third, mutations in amino acid residues in the Ca2+ bowl and RCK domain greatly attenuated LPA-mediated enhancement of the BKCa channel currents. Thus, since BKCa channels play an important role in presynaptic nerve terminals and blood vessel smooth muscle cells, the findings in the present study suggest the possibility that LPA may be a novel BKCa channel regulator in the nervous and vascular systems via PLC-IP3-Ca2+ signal transduction pathways.
To confirm that the LPA-mediated [Ca2+]i transient is coupled to the activation of BKCa channels, we first treated oocytes with BAPTA-AM, a membrane permeable Ca2+ chelator. As shown in Fig. 4C, BAPTA-AM treatment abolished to 98.5% of the control (p<0.001, compared to BAPTA un-treatment). In addition, we constructed mutant channels in the Ca2+ bowl and RCK domain. As shown in Fig. 5B, mutations of several amino acid residues in the Ca2+ bowl and RCK domain greatly attenuated LPA-mediated BKCa channel activation, indicating that extracellular LPA treatment induces the mobilization of cytosolic Ca2+ from the endoplasmic reticulum; the increased Ca2+ ions bind the Ca2+ bowl and RCK domain in order to activate the BKCa channels.
BKCa channels are ideal pharmacological targets, since the channel opening increases the attenuation of neuron excitability and smooth muscle relaxation in an existing physiological system. The in vitro LPA enhancement of brain BKCa channel currents also has in vivo pharmacological effects. The enhancing effects of LPA on the channel currents may contribute to repolarization of the action potential neurons and vascular smooth muscle cells, while shortening the action potential duration. Thus, activating BKCa channels by LPA might be used to reduce over-excitability of the nervous system or to down-regulate hyperactivity of vascular smooth muscle cells. This study also raises the possibility that LPA participates in the regulation of synaptic transmission in nerve terminals and vascular systems.
On the other hand, recent report showed that LPA is also related with pain induction in spinal cord, since LPA synthesized in the spinal dorsal horn after nerve injury [24]. LPA-mediated intracellular [Ca2+]i increase not only stimulates microglia to induce the BDNF synthesis through activation of P2X4 receptors, but also activates activation of microglial BKCa channels [25]. BDNF secreted from microglia in turn further enhance the activities of BK channels [19,26]. Thus, it seems that the physiological or pharmacological roles of LPA-mediated BK channel activations might be dependent on cell types or organs. Further investigations into the potential clinical applications of LPA on the nervous and vascular systems are required.
In conclusion, we found that activation of Gαq/11 protein-coupled LPA receptor by LPA is coupled to BKCa channel activation and that Ca2+ bowl and RCK domain of BKCa channel also play important roles in LPA-mediated BKCa channel activation using site-directed mutagenesis method. The present findings may provide a molecular basis for LPA effects on the nervous and vascular systems.
ACKNOWLEDGEMENTS
This paper was supported by the SMART Research Professor Program of Konkuk University.
References
1. Ghatta S, Nimmagadda D, Xu X, O'Rourke ST. Large-conductance, calcium-activated potassium channels: structural and functional implications. Pharmacol Ther. 2006; 110:103–116. PMID: 16356551.


2. Salkoff L, Butler A, Ferreira G, Santi C, Wei A. High-conductance potassium channels of the SLO family. Nat Rev Neurosci. 2006; 7:921–931. PMID: 17115074.


3. Yuan P, Leonetti MD, Pico AR, Hsiung Y, MacKinnon R. Structure of the human BK channel Ca2+-activation apparatus at 3.0 A resolution. Science. 2010; 329:182–186. PMID: 20508092.
4. Weiger TM, Hermann A, Levitan IB. Modulation of calciumactivated potassium channels. J Comp Physiol A Neuroethol Sens Neural Behav Physiol. 2002; 188:79–87. PMID: 11919690.


5. Fahanik-Babaei J, Eliassi A, Saghiri R. How many types of large conductance Ca2+-activated potassium channels exist in brain mitochondrial inner membrane: evidence for a new mitochondrial large conductance Ca2+-activated potassium channel in brain mitochondria. Neuroscience. 2011; 199:125–132. PMID: 21996476.
6. Jiang Y, Pico A, Cadene M, Chait BT, MacKinnon R. Structure of the RCK domain from the E. coli K+ channel and demonstration of its presence in the human BK channel. Neuron. 2001; 29:593–601. PMID: 11301020.
7. Lee US, Cui J. BK channel activation: structural and functional insights. Trends Neurosci. 2010; 33:415–423. PMID: 20663573.


8. Niu X, Qian X, Magleby KL. Linker-gating ring complex as passive spring and Ca2+-dependent machine for a voltage- and Ca2+-activated potassium channel. Neuron. 2004; 42:745–756. PMID: 15182715.
9. Tigyi G. Aiming drug discovery at lysophosphatidic acid targets. Br J Pharmacol. 2010; 161:241–270. PMID: 20735414.


10. Contos JJ, Ishii I, Chun J. Lysophosphatidic acid receptors. Mol Pharmacol. 2000; 58:1188–1196. PMID: 11093753.


11. Moolenaar WH, Kranenburg O, Postma FR, Zondag GC. Lysophosphatidic acid: G-protein signalling and cellular responses. Curr Opin Cell Biol. 1997; 9:168–173. PMID: 9069262.


12. Dyer D, Tigyi G, Miledi R. The effect of active serum albumin on PC12 cells: I Neurite retraction and activation of the phosphoinositide second messenger system. Brain Res Mol Brain Res. 1992; 14:293–301. PMID: 1326692.


13. Chettibi S, Lawrence AJ, Stevenson RD, Young JD. Effect of lysophosphatidic acid on motility, polarisation and metabolic burst of human neutrophils. FEMS Immunol Med Microbiol. 1994; 8:271–281. PMID: 8004064.


14. Ha TS, Lim HH, Lee GE, Kim YC, Park CS. Electrophysiological characterization of benzofuroindole-induced potentiation of large-conductance Ca2+-activated K+ channels. Mol Pharmacol. 2006; 69:1007–1014. PMID: 16332986.
15. Choi S, Rho SH, Jung SY, Kim SC, Park CS, Nah SY. A novel activation of Ca2+-activated Cl- channel in Xenopus oocytes by Ginseng saponins: evidence for the involvement of phospholipase C and intracellular Ca2+ mobilization. Br J Pharmacol. 2001; 132:641–648. PMID: 11159716.
16. Lee JH, Lee BH, Choi SH, Yoon IS, Pyo MK, Shin TJ, Choi WS, Lim Y, Rhim H, Won KH, Lim YW, Choe H, Kim DH, Kim YI, Nah SY. Ginsenoside Rg3 inhibits human Kv14 channel currents by interacting with the Lys531 residue. Mol Pharmacol. 2008; 73:619–626. PMID: 17959711.


17. Lu L, Montrose-Rafizadeh M, Guggino WB. Ca2+-activated K+ channels from rabbit kidney medullary thick ascending limb cells expressed in Xenopus oocytes. J Biol Chem. 1990; 265:16190–16194. PMID: 1697853.
18. Candia S, Garcia ML, Latorre R. Mode of action of iberiotoxin, a potent blocker of the large conductance Ca2+-activated K+ channel. Biophys J. 1992; 63:583–590. PMID: 1384740.
19. Langer P, Gründer S, Rüsch A. Expression of Ca2+-activated BK channel mRNA and its splice variants in the rat cochlea. J Comp Neurol. 2003; 455:198–209. PMID: 12454985.
20. Kaczorowski GJ, Knaus HG, Leonard RJ, McManus OB, Garcia ML. High-conductance calcium-activated potassium channels; structure, pharmacology, and function. J Bioenerg Biomembr. 1996; 28:255–267. PMID: 8807400.


21. Kimura Y, Schmitt A, Fukushima N, Ishii I, Kimura H, Nebreda AR, Chun J. Two novel Xenopus homologs of mammalian LP(A1)/EDG-2 function as lysophosphatidic acid receptors in Xenopus oocytes and mammalian cells. J Biol Chem. 2001; 276:15208–15215. PMID: 11278944.
22. Yusifov T, Savalli N, Gandhi CS, Ottolia M, Olcese R. The RCK2 domain of the human BKCa channel is a calcium sensor. Proc Natl Acad Sci U S A. 2008; 105:376–381. PMID: 18162557.


23. Schreiber M, Salkoff L. A novel calcium-sensing domain in the BK channel. Biophys J. 1997; 73:1355–1363. PMID: 9284303.


24. Ma L, Uchida H, Nagai J, Inoue M, Aoki J, Ueda H. Evidence for de novo synthesis of lysophosphatidic acid in the spinal cord through phospholipase A2 and autotaxin in nerve injuryinduced neuropathic pain. J Pharmacol Exp Ther. 2010; 333:540–546. PMID: 20123931.


25. Hayashi Y, Kawaji K, Sun L, Zhang X, Koyano K, Yokoyama T, Kohsaka S, Inoue K, Nakanishi H. Microglial Ca2+-activated K+ channels are possible molecular targets for the analgesic effects of S-ketamine on neuropathic pain. J Neurosci. 2011; 31:17370–17382. PMID: 22131399.
26. Gao Y, Yang Y, Guan Q, Pang X, Zhang H, Zeng D. IL-1beta modulate the Ca2+-activated big-conductance K channels (BK) via reactive oxygen species in cultured rat aorta smooth muscle cells. Mol Cell Biochem. 2010; 338:59–68. PMID: 19949838.
Fig. 1
Schematic transmembrane diagram of the BKCa channel in the plasma membrane and the LPA chemical structure. (A) There are 7 transmembrane domains along with the RCK1 and RCK2 domains. The voltage sensor and pore of the BKCa channel resembles the responding regions of other voltage-dependent K+ channels (Yuan et al., 2010). Amino acid residues underlined and marked (*) indicate mutation sites in this study. (B) The chemical structure of lysophosphatidic acid (LPA, C18:1).
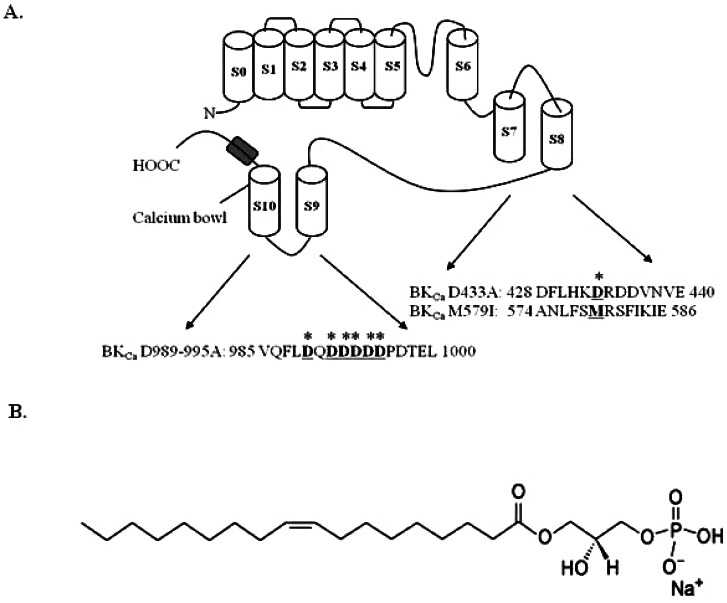
Fig. 2
Concentration- and voltage-dependent activation of the BKCa channel by LPA in Xenopus oocytes expressing rat brain BKCa channels. (A) The BKCa channel activation by LPA is concentration-dependent. (a) Representative traces of BKCa channel current enhancements using different concentrations of LPA (up to 1 µM). (b) The summary histogram shows the effects of various concentrations of LPA on the BKCa channels. (B) A representative control and LPA-mediated current in an I~V relationship. (a) Currents were recorded at a test potential from -70 to +60 mV, applied from a holding potential of -80 mV. (b) Current-voltage (I-V) relationship of the BKCa channel in the absence (●) or presence (○) of 100 nM LPA. Voltage pulses with 400-ms duration were applied in 10 mV increments at 3-s intervals from a holding potential of -80 mV. The current peaks, normalized to the peak current evoked by the voltage step to +60 mV in the absence of LPA, were used in the I-V plot. Data represent the mean±S.E.M. (n=4).
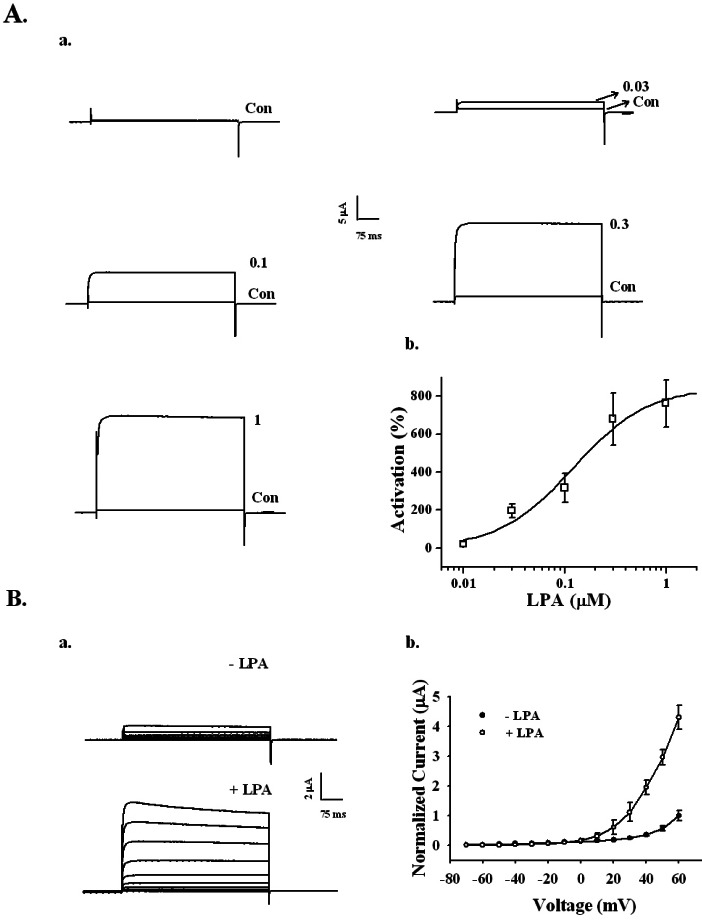
Fig. 3
Effects of repeated treatments of LPA and LPA1/3 receptor antagonist Ki16425 on LPA-mediated BKCa channel activation. (A-a) Repeated application of 100 nM LPA desensitized the BKCa channel activation with indicated time interval in representative oocyte (left panel). (A-b) The representative BKCa channel current traces by repeated LPA treatments were obtained from timecurrent relationship of "a" (right panel). Outward currents are caused by stepping from -80 mV to +40 mV for 400 ms. Data represent the mean±S.E.M. (n=5). (B-a) Representative traces are from the LPA channel activation in the absence (upper trace) or presence of 10 µM Ki16425 for 10 min (lower trace). (B-b) Summary histogram of the LPA effects on BKCa channel activation (right panel). Data represent the mean±S.E.M. (n=10~12; *p<0.01).
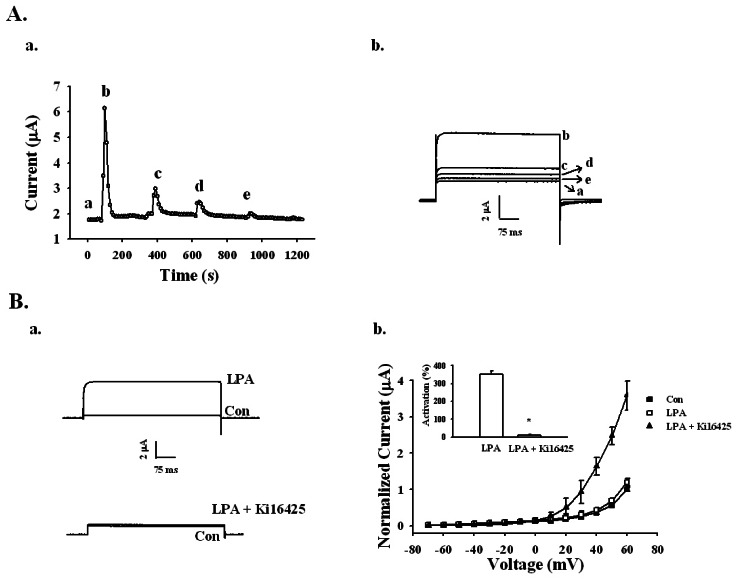
Fig. 4
Signaling pathway of LPA-mediated BKCa channel activation. (A) Effects of the PLC inhibitor on LPA-mediated BKCa channel activation. (a~c) A representative trace shows the activation of LPA-(100 nM) mediated currents in Xenopus oocytes expressing the BKCa channel in the absence or presence of inactive U73343 (1 µM) or active PLC inhibitor U73122 (1 µM). (d) Summary histograms of LPA-mediated activation on control, U-73122, or U-73343-treated groups (right lower panel). Data represent the mean±S.E.M. (n=10~11; *p<0.01). (B) Effect of IP3 receptor antagonist 2-APB on LPA-mediated (100 nM) BKCa channel activation. (a) Representative trace shows the activation of LPA-mediated (100 nM) currents in Xenopus oocytes expressing the BKCa channel in the absence or presence of 100 µM 2-APB for 20 min. (b) Summary histograms of LPA-mediated activation on control or 100 µM 2-APB (right lower panel). Data represent the mean±S.E.M. (n=12~14; *p<0.05). (C) Effect of intracellular Ca2+ chelator, BAPTA-AM, on LPA-mediated (100 nM) BKCa channel activation. (a) A representative trace shows the activation of LPA-mediated (100 nM) currents in Xenopus oocytes expressing the BKCa channel in the absence or presence of BAPTA-AM for 3 h. (b) Summary histograms of LPA-mediated activation on control or 100 µM BAPTA-AM (right lower panel). Data represent the mean±S.E.M. (n=10~12; *p<0.01).
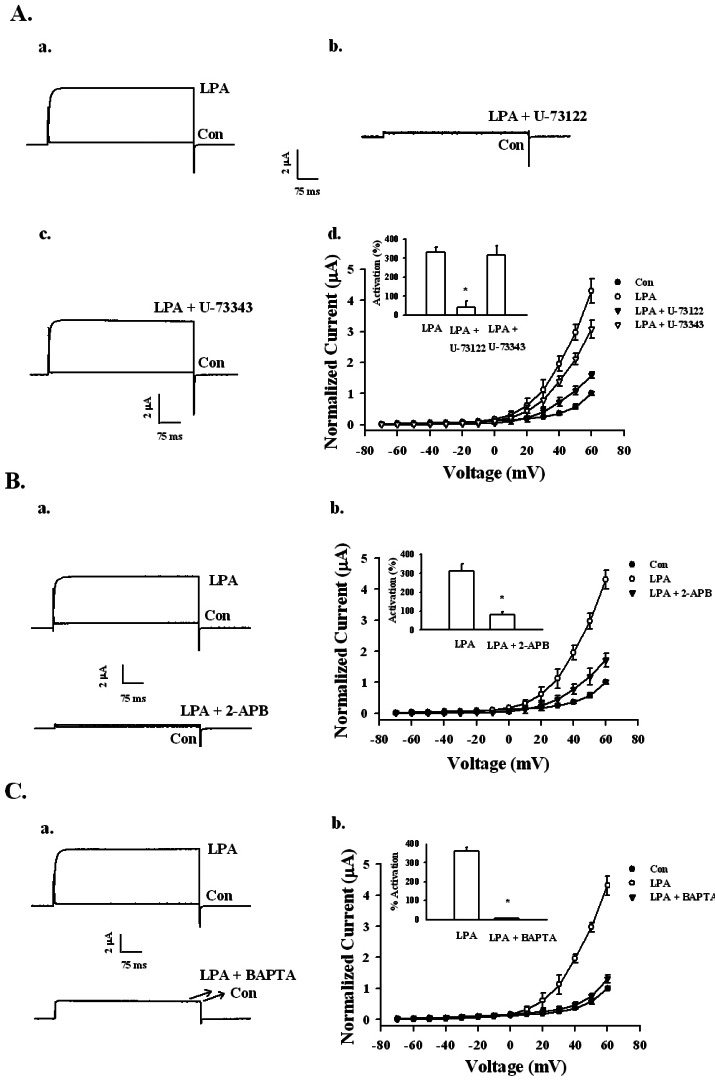
Fig. 5
Effects of PKC inhibitor and mutations in RCK1 and the Ca2+ bowl on LPA-mediated BKCa channel activation. (A-a) Effects of PKC inhibitor on LPA-mediated BKCa channel activation. A representative trace shows the activation of LPA-mediated (100 nM) currents in Xenopus oocytes expressing the BKCa channel in the absence or presence of 1.5 µM calphostin for 2 h. (A-b) Summary histograms of LPA-mediated BKCa channel activation in controls or with 1.5 µM calphostin (right lower panel). Data represent the mean±S.E.M. (n=10~12; *p<0.01). (B) Effect of mutations on the RCK1 and Ca2+ bowl domains in LPA-mediated BKCa channel activation. (B-a) The oocytes expressing wild-type or mutant BKCa channels in the RCK domain such as D433A or M579I were treated with LPA by a bathing application for 60 s. Mutations in the RCK domain attenuated the LPA concentration-responses (left panel). (B-b) The oocytes expressing wild-type or mutant BKCa channels in the Ca2+ bowl were treated with LPA by a bathing application for 60 s. Mutations in the Ca2+ bowl also attenuated LPA concentration-responses (right panel). Data represent the mean±S.E.M. (n=6).
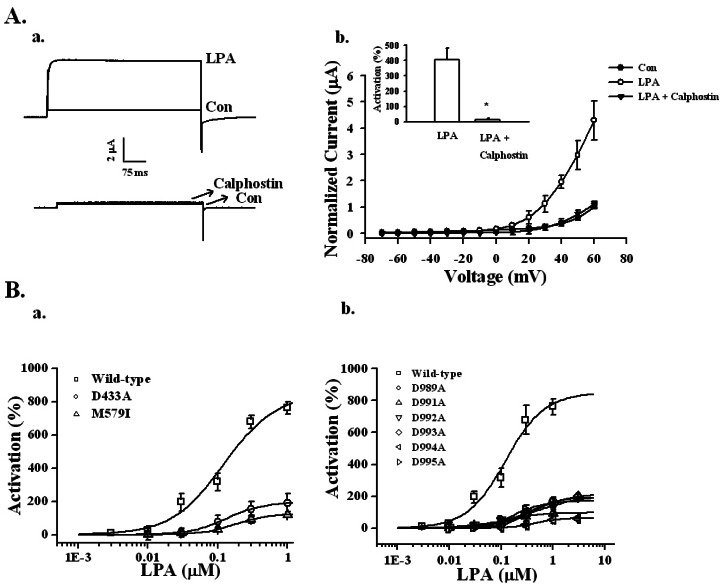