Abstract
Interstitial cells of Cajal (ICCs) are the pacemaker cells in the gastrointestinal tract, and histamine is known to regulate neuronal activity, control vascular tone, alter endothelial permeability, and modulate gastric acid secretion. However, the action mechanisms of histamine in mouse small intestinal ICCs have not been previously investigated, and thus, in the present study, we investigated the effects of histamine on mouse small intestinal ICCs, and sought to identify the receptors involved. Enzymatic digestions were used to dissociate ICCs from small intestines, and the whole-cell patch-clamp configuration was used to record potentials (in current clamp mode) from cultured ICCs. Histamine was found to depolarize resting membrane potentials concentration dependently, and whereas 2-PEA (a selective H1 receptor agonist) induced membrane depolarizations, Dimaprit (a selective H2-agonist), R-alpha-methylhistamine (R-alpha-MeHa; a selective H3-agonist), and 4-methylhistamine (4-MH; a selective H4-agonist) did not. Pretreatment with Ca2+-free solution or thapsigargin (a Ca2+-ATPase inhibitor in endoplasmic reticulum) abolished the generation of pacemaker potentials and suppressed histamine-induced membrane depolarization. Furthermore, treatments with U-73122 (a phospholipase C inhibitor) or 5-fluoro-2-indolyl des-chlorohalopemide (FIPI; a phospholipase D inhibitor) blocked histamine-induced membrane depolarizations in ICCs. On the other hand, KT5720 (a protein kinase A inhibitor) did not block histamine-induced membrane depolarization. These results suggest that histamine modulates pacemaker potentials through H1 receptor-mediated pathways via external Ca2+ influx and Ca2+ release from internal stores in a PLC and PLD dependent manner.
Histamine is a biogenic amine and is synthesized from the basic amino acid histidine. Histamine is a major mediator of inflammatory and allergic reactions [1] and plays important roles in various physiological processes, for example, it regulates neuronal activity, controls vascular tone, alters endothelial permeability, modulates gastric acid secretion, and participates in inflammatory responses [2-5]. There are four histamine receptors, H1 [6], H2 [7], H3 [8], and H4 [9], through which histamine can exert its effects. H1 receptors are widely expressed in enterocytes, muscle layer, blood vessels, immune cells and ganglion cells [10]. H2 receptors are located on parietal cells in fundic mucosa, intestinal epithelium, immune cells, and myenteric ganglia [10]. H3 receptors are presumably located on submucosal neurons from human small and large intestine [11], whereas H4 receptor is mainly expressed in leucocytes in mucosa and is colonic submucosal blood vessels [9,10].
Many regions of the tunica muscularis of the gastrointestinal (GI) tract display spontaneous contractions, which are mediated by the periodic generation of electrical slow waves [12]. Furthermore, recent studies have shown that interstitial cells of Cajal (ICCs) act as the pacemakers and conductors of electrical slow waves in GI smooth muscles [13-17].
The histaminergic control of GI motility is very complex and consists of neurally mediated as well as direct effects on the smooth muscle contractility. Despite the multiplicity of studies carried out to characterize histamine receptors in GI tissues, there are few studies of the importance of these receptors in the control of physiologic GI motility is still not well established. Results obtained have sometimes been contradictory [18], suggesting the existence of species differences in the distribution [19,20] and functional role of histamine receptors [21]. Also, a central inhibitory control of intestinal propulsion by histamine receptors was demonstrated in the rat [22]. Oishi et al. [23] studied the effect of histamine receptor ligands on the intestinal transit of a charcoal meal in mice, but did not observe any H receptor mediated effect in response to the histamine-receptor agonist. Recently, Kim et al. [24] investigated the expression of histamine receptors and responses to histamine in colonic contractility in mouse and monkey and they suggested that histamine receptor expression in simian colon is closer to that reported in human. However, the effects of histamine on mouse small intestinal ICCs have not been previously investigated, and thus, we conducted this study to investigate the effects of histamine in mouse small intestinal ICCs.
All animals used were treated ethically according to the 'Guidelines for the Care and Use of Animals' issued by Pusan National University. Balb/c mice (8-13-days-old) of either sex were anaesthetized with ether and sacrificed by cervical dislocation. Small intestines were removed from 1 cm below the pyloric ring to the cecum and opened along the mesenteric border. Luminal contents were washed out with Krebs-Ringer bicarbonate solution, tissues were pinned to the base of a Sylgard dish, and mucosa was removed by sharp dissection. Small strips of intestinal muscle (consisting of both circular and longitudinal muscles) were then equilibrated in Ca2+ free Hank's solution containing 5.36 mM KCl, 125 mM NaCl, 0.34 mM NaOH, 0.44 mM Na2HCO3, 10 mM glucose, 2.9 mM sucrose, and 11 mM N-(2-hydroxyethyl)piperazine-N-2-ethanesulfonic acid (HEPES) for 30 min. Cells were then dispersed in a solution containing 1.3 mg/ml collagenase (Worthington Biochemical, Lakewood, NJ, USA), 2 mg/ml bovine serum albumin (Sigma-Aldrich, St. Louis, MO, USA), 2 mg/ml trypsin inhibitor (Sigma-Aldrich) and 0.27 mg/ml ATP, plated onto sterile glass coverslips coated with 2.5 mg/ml murine collagen (Falcon/BD, USA) in 35 mm-diameter culture dishes, and cultured at 37℃ in a 95% O2-5% CO2 incubator in smooth muscle growth medium (SMGM; Clonetics, San Diego, CA) supplemented with 2% antibiotics/antimycotics (Gibco, Franklin Lakes, NJ, USA) and 5 ng/ml murine stem cell factor (SCF; Sigma-Aldrich). ICCs were identified immunologically by incubation with anti-c-kit antibody [phycoerythrin (PE)-conjugated rat anti-mouse c-kit monoclonal antibody; eBioscience, San Diego, CA] at a dilution of 1:50 for 20 min. Because ICCs differed morphologically from other cell types in cultures, they were identified by phase contrast microscopy after incubation with anti-c-kit antibody.
The physiological salt solution used to bathe cells (Na+-Tyrode) contained; 5 mM KCl, 135 mM NaCl, 2 mM CaCl2, 10 mM glucose, 1.2 mM MgCl2, and 10 mM HEPES, adjusted to pH 7.4 with NaOH. The pipette solution contained 140 mM KCl, 5 mM MgCl2, 2.7 mM K2ATP, 0.1 mM NaGTP, 2.5 mM creatine phosphate disodium, 5 mM HEPES, and 0.1 mM ethylene glycol bis(2-aminoethyl ether)-N,N,N',N'-tetraacetic acid (EGTA), adjusted to pH 7.2 with KOH. Single ICCs used in patch clamp experiments were bathed in a solution containing 2.8 mM KCl, 145 mM NaCl, 2 mM CaCl2, 10 mM glucose, 1.2 mM MgCl2 and 10 mM HEPES, adjusted to pH 7.4 with NaOH. The pipette solution contained 145 mM Cs-glutamate, 8 mM NaCl, 10 mM Cs-2-bis(2-aminophenoxy)-ethane-N,N,N',N'-tetraacetic acid, and 10 mM HEPES-CsOH, adjusted to pH 7.2 with CsOH. The whole-cell configuration patch-clamp technique was used to record membrane potentials (current clamp) of cultured ICC, and an Axopatch I-D (Axon Instruments, Aberdeen, UK) was used to amplify membrane currents and potentials. Command pulses were applied using an IBM-compatible personal computer and pClamp software (version 6.1; Axon Instruments). Data were filtered at 5 kHz, displayed on an oscilloscope and a computer monitor, printed using a Gould 2200 pen recorder (Gould, Valley View, OH, USA), and analyzed using pClamp and Origin (version 6.0) software. All experiments were performed at 30℃.
The drugs used in the experiments, namely, histamine, tetrodotoxin (TTX), thapsigargin, U-73122, 5-fluoro-2-indolyl des-chlorohalopemide (FIPI), and KT5720, were purchased from Sigma-Aldrich. 2-Pyridylethylamine (2-PEA), Dimaprit, R-alpha-methylhistamine (R-alpha-MeHa), 4-methylhistamine (4-MH), cetirizine and other drugs were purchased from TOCRIS (Bristol, UK). Appropriate solvents (DMSO or distilled water) were used to dissolve drugs and prepare stock solutions (50 or 100 mM), which were stored in aliquots at the designated temperatures. Required concentrations of drugs were prepared during experiments and added to bath solutions. All drugs were applied to whole cell preparations by superfusion. The final concentration of DMSO in all drug preparations was <0.1% and at this level DMSO did not affect recorded traces.
The patch-clamp technique was applied to ICCs that formed network-like structures in culture (2~4 days), as these ICCs displayed more robust electrical rhythms. Spontaneous rhythms were routinely recorded under current and voltage-clamp conditions. Tissue-like spontaneous slow waves have been previously recorded from these cells [25]. To understand the effect of histamine on the pacemaker activities of ICCs, we examined its effects on pacemaker potentials. Recordings from cultured ICCs in current clamp mode (I=0) showed spontaneous pacemaker potentials. The resting membrane potential was -51±2.4 mV and its amplitude was 21.3±2.2 mV. In the presence of histamine (10~100µM), membrane potentials were depolarized to 5.1±0.8 mV at 10µM (n=5), 21.4±2.1 mV at 50µM (n=4), and 37.2±2.1 mV at 100µM (n=5) (Fig. 1A~C), and amplitudes decreased to 20.1±2.4 mV at 10µM (n=5), 10.1±2.3 mV at 50µM (n=4), and 5.2±2.3 mV at 100µM (n=5) (Fig. 1A~C). Summarized values and a bar graph of the depolarizing effects of histamine on pacemaker potentials are provided in Fig. 1D. Also, to identify the involvement of neuronal mechanisms, tetrodotoxin (TTX, 1µM), a voltage-dependent Na+ channel blocker, was tested. TTX had no effects on the pacemaker potentials (n=4; data not shown).
In this experiment, a high concentration of histamine (50µM) was used to induce more depolarizations, which allows for easier detection of the reduction of depolarizations by histamine treatment. To identify the receptor subtypes of histamine involved in cultured ICCs, cells were treated with various histamine receptor agonists. 2-Pyridylethylamine (2-PEA) (a selective H1-agonist) [26], Dimaprit (a selective H2-agonist) [24], R-alpha-methylhistamine (R-alpha-MeHa) (a selective H3-agonist) [24], and 4-methylhistamine (4-MH) (a selective H4-agonist) [27] were all administered at a concentration of 50µM. 2-PEA depolarized pacemaker potentials, but others histamine receptor agonists had no effects on pacemaker potentials (n=5, respectively; Fig. 2A). To confirm the involvement of H1 receptor, ICCs were pretreated with cetirizine (a selective H1-antagonist) [28] and then treated with histamine. After pre-treatment with cetirizine, membrane depolarization by histamine was blocked (n=4; Fig. 2E); membrane depolarization produced in the presence of cetirizine by histamine was 1.2±1.3 mV (Fig. 2F). On the other hand, pretreatment with the selective H2-, H3-, H4-antagonists did not block membrane depolarization by histamine (data not shown). Summarized values and a bar graph of the effects of histamine receptor agonists on pacemaker potentials are provided in Fig. 2F.
Ca2+ influx is required for GI smooth muscle contractions and for generating pacemaker potentials in ICCs. Furthermore, the generation of pacemaker potentials is dependent on intracellular Ca2+ oscillations [17]. To investigate the effects on external and internal Ca2+ on histamine-induced responses, histamine was tested under external norminal Ca2+-free conditions and in the presence of thapsigargin (a Ca2+-ATPase inhibitor in endoplasmic reticulum) [29]. Pacemaker potentials were completely abolished by an external norminal Ca2+-free solution, and under this condition, histamine did not induce membrane depolarization (1.5±0.28 mV) (n=3; Fig. 3A). Furthermore, membrane depolarization by histamine (50µM) was significantly less than that caused by histamine in normal Ca2+ containing solution (2 mM CaCl2; 21.3±0.8 mV) (n=5; Fig. 3C). In addition, histamine-induced membrane depolarizations were inhibited by thapsigargin pretreatment (1.25±0.3 mV; n=4; Fig. 3B); in the presence of thapsigargin (5µM), membrane depolarization by histamine was significantly smaller than that induced by histamine in the absence of thapsigargin (21.3±0.8 mV) (n=5, Fig. 3B~C).
Stimulation of histamine H1 receptors activates PLC, which leads to the increased production of inositol 1,4,5-triphosphate (InsP3) [30] and subsequent Ca2+ mobilization from InsP3-sensitive Ca2+ stores [31], as demonstrated in many different tissues, such as, brain, heart, intestine, vascular tissues, tracheal epithelium, and airway smooth muscle [32-34]. Thus, we examined whether or not PLC, PLD, and PKA are involved in these effects of histamine using U-73122 (a PLC inhibitor), 5-Fluoro-2-indolyl des-chlorohalopemide (FIPI) (a PLD inhibitor), or KT5720 (a PKA inhibitor). In the presence of U-73122, histamine did not cause membrane depolarization (n=6; Fig. 4A), indicating that PLC plays a role in histamine-induced membrane depolarization. In the presence of FIPI, the membrane potentials was a little hyperpolarized and histamine did not cause membrane depolarization (n=6; Fig. 4B), indicating that PLD plays a role in histamine-induced membrane depolarization. However, the PKA inhibitor KT5720 did not block membrane depolarization by histamine (n=5; Fig. 4C). Summarized values and a bar graph of the effects of histamine on pacemaker potentials are provided in Fig. 4D.
It has been reported that histamine actives transient receptor potential canonical 3 (TRPC3) channels [35], and thus, we investigated whether TRPC3 channels are involved in the membrane depolarization caused by histamine in cultured ICCs. In the presence of pyr3 (selective TRPC3 channel inhibitor [36]), the membrane potentials was not changed and histamine did not produce membrane depolarization (n=4; Fig. 5A), indicating that TRPC3 channels play a role in histamine-induced membrane depolarization. Summarized values and a bar graph of the effects of histamine on pacemaker potentials are provided in Fig. 5B.
ICCs generate spontaneous pacemaker potentials that depolarize their membranes and spread to smooth muscle cells via gap junctions to depolarize smooth muscle cell membranes. Furthermore, it is known that pacemaker potentials are initiated by the release of Ca2+ from the endoplasmic reticulum. Cyclopiazonic acid (a Ca2+ ATPase inhibitor in endoplasmic reticulum) or xestospongin C (an inhibitor of inositol (1,4,5)-triphosphate receptor in endoplasmic reticulum) abolished the generation of pacemaker potentials, suggesting that inositol (1,4,5)-triphosphate-mediated Ca2+ release from the endoplasmic reticulum is essentially required for pacemaker current generation. Because of the central role played by ICCs in GI motility, loss of these cells would be extremely detrimental. Thus, research into the biology of ICCs provides exciting new opportunities to understand the etiology of diseases that have long eluded comprehension.
Few studies have addressed the effect of histamine on GI motility. In one study, it was found histamine induced a dominant contractile response in human colon tissue strips, and that the effects of histamine were not dependent upon nervous activity [37]. Bolton et al. [38] showed that histamine depolarized longitudinal smooth muscle cells and increased action potential discharge in guinea-pig ileum. Recently, Kim et al. [24] investigated that H1, H2 and H4 receptor transcripts were expressed at similar levels in simian colonic tissue whereas only the H2 receptor transcript was detected in murine colonic tissue. Stimulation of simian colonic muscles with histamine caused depolarization and contraction in the presence of TTX. Histamine activated non-selective cation channels in simian smooth muscle cells. In contrast, histamine caused hyperpolarization and inhibited contractions of murine colon. The hyperpolarization was inhibited by the KATP channel blocker, glibenclamide. Histamine-activated K+ currents were inhibited by glibenclamide in murine colonic smooth muscle cells. Therefore, they suggested that since histamine receptor expression in simian colon is closer to that reported in human, they suggested that it is a more suitable model to predict the effects of the inflammatory mediator, histamine, on colonic motility in humans [24]. However, previous studies on the effects of histamine on GI motility studies were usually performed on smooth muscles, and no investigation has been conducted on the effects of histamine on ICCs, the pacemaker cells in the GI tract.
Here we evaluated the effects of histamine in ICCs and examined responses to a variety of histamine receptor-specific agonists. Histamine has diverse effects on tissues due to expressions of specific H receptors and the activations of distinct intracellular pathways [39]. In particular, H1 receptors are coupled to Gq/11 proteins, which activate PLC and the phosphatidylinositol 4,5-bisphosphate signaling pathway [40,41]. In addition, in equine tracheal smooth muscle cells, intracellular Ca2+ release, due to stimulation of the H1/Gq/11 pathway, was found to be necessary for the histamine-induced activation of nonselective cation channels (NSCC) [41]. Subsequently, Kwan et al. [35] suggested that TRPC3 channels serve as molecular identifiers of NSCCs by histamine. In addition, in the present study, we identified the involvement of TRPC3 channels in histamine-induced membrane depolarizations in murine small intestine ICCs. In ICCs, there were many types of TRP channels. In cultured ICC clusters, TRPM2, TRPM3, TRPM7, and TRPM8 were expressed in murine small intestine [42]. Also, TRPC4 was expressed in ICCs and smooth muscles [43,44]. In murine stomach ICCs, TRP mRNA except TRPC5 were detected [45]. The implication of TRP channels in physiological and pathological processes of ICCs within the GI tract have raised enormous interest in the therapeutic exploitation. Therefore, these TRP channels seem to play many important physiological roles within the GI system.
Kim et al. [24] suggested that only the H2 receptor transcript was detected in murine colonic tissue. These results are different from our this study. They used the longitudinal smooth muscle cells in colon and found the H2 transcript by RT-PCR, but we used the cultured ICCs in small intestine. Also when we cultured the colonic ICCs and measured membrane potentials, the membrane potentials were irregular types. However, in small intestine, the membrane potentials were regular types. Therefore, we think that the mechanisms of GI motility is different between small intestine and colon.
Generally speaking, histamine works from 1µM to 10µM [24]. However, in this study, histamine worked at high concentrations (>10µM). Maybe, we think that it might be damage or reduce sensitivity of receptors during cultures. Histamine (high concentrations)-induced depolarizations decayed spontaneously during prolonged stimulation of H1 receptors in ICCs (desensitization). This is true especially in carbachol [46] and substance P [47]. The spontaneous decrease of the responses to sustained receptor stimulation has been explained by various mechanisms that include a decrease in receptor number [48] and autoinhibitory feedback to signal-transducing molecules (e.g. G-proteinor) or effector molecules (e.g. ion channel) [49,50]. Also, it was explained by Ca2+ dependent-PKC mediated inhibition [51]. Desensitization of histamine-induced depolarizations may be an example of a negative feedback mechanism in GI motility, and may prevent prolonged depolarization. Without such negative feedback, recycling histamine-induced depolarizations may induce excessive Ca2+ influx and contraction.
ICCs are the pacemakers and the conductors of the electrical slow waves in GI smooth muscles [13-17]. Moreover there is evidence that endogenous agents such as neurotransmitters, hormones and paracrine substances modulate gastrointestinal tract motility by influencing the ICCs [52-54]. Consequently, ICCs are involved not only in physiological GI motility, but also in many bowel disorders, including inflammatory bowel disease, chronic idiopathic intestinal pseudo-obstruction, intestinal obstruction with hypertrophy, achalasia, Hirschsprung disease, juvenile pyloric stenosis, juvenile intestinal obstruction, and anorectal malformation. Thus, the study of endogenous agents such as neurotransmitters, hormones and paracrine substances (ex. histamine) is very important to understand the GI motility and the target for pharmacological treatment of GI motility disorders.
In the present study, histamine produced membrane depolarization in current-clamp mode, and pretreatment with a Ca2+-free solution or with thapsigargin (a Ca2+-ATPase inhibitor in endoplasmic reticulum) abolished the generation of pacemaker potentials. Under Ca2+-free conditions or in the presence of thapsigargin, histamine did not induce membrane depolarization. Furthermore, 2-PEA (a selective H1-receptor agonist) induced membrane depolarizations, whereas Dimaprit (a selective H2-agonist), R-alpha-methylhistamine (R-alpha-MeHa) (a selective H3-agonist), and 4-methylhistamine (4-MH) (a selective H4-agonist) did not. In addition, pacemaker membrane depolarization by histamine was inhibited by U-73122 or FIPI, but not by KT5720, and pyr3 blocked histamine-induced membrane depolarization. These results suggest that histamine affects GI motility by modulating pacemaker activity in ICCs, and that this activation is associated with TRPC3 channels via PLC and PLD activation and Ca2+ release from internal stores or occurs in an external Ca2+-dependent manner.
ACKNOWLEDGEMENTS
This research was supported by the Basic Science Research Program through the Korean National Research Foundation (NRF) funded by the Ministry of Education, Science and Technology (Grant no. 2010-0021347).
References
1. Dy M, Schneider E. Histamine-cytokine connection in immunity and hematopoiesis. Cytokine Growth Factor Rev. 2004; 15:393–410. PMID: 15450254.


2. Hofstra CL, Desai PJ, Thurmond RL, Fung-Leung WP. Histamine H4 receptor mediates chemotaxis and calcium mobilization of mast cells. J Pharmacol Exp Ther. 2003; 305:1212–1221. PMID: 12626656.
3. Niimi N, Noso N, Yamamoto S. The effect of histamine on cultured endothelial cells. A study of the mechanism of increased vascular permeability. Eur J Pharmacol. 1992; 221:325–331. PMID: 1426009.
4. Schaefer U, Schneider A, Rixen D, Neugebauer E. Neutrophil adhesion to histamine stimulated cultured endothelial cells is primarily mediated via activation of phospholipase C and nitric oxide synthase isozymes. Inflamm Res. 1998; 47:256–264. PMID: 9683033.


5. Yanai K, Son LZ, Endou M, Sakurai E, Nakagawasai O, Tadano T, Kisara K, Inoue I, Watanabe T, Watanabe T. Behavioural characterization and amounts of brain monoamines and their metabolites in mice lacking histamine H1 receptors. Neuroscience. 1998; 87:479–487. PMID: 9740406.


6. De Backer MD, Gommeren W, Moereels H, Nobels G, Van Gompel P, Leysen JE, Luyten WH. Genomic cloning, heterologous expression and pharmacological characterization of a human histamine H1 receptor. Biochem Biophys Res Commun. 1993; 197:1601–1608. PMID: 8280179.
7. Gantz I, Munzert G, Tashiro T, Schäffer M, Wang L, DelValle J, Yamada T. Molecular cloning of the human histamine H2 receptor. Biochem Biophys Res Commun. 1991; 178:1386–1392. PMID: 1714721.


8. Lovenberg TW, Roland BL, Wilson SJ, Jiang X, Pyati J, Huvar A, Jackson MR, Erlander MG. Cloning and functional expression of the human histamine H3 receptor. Mol Pharmacol. 1999; 55:1101–1107. PMID: 10347254.
9. Oda T, Morikawa N, Saito Y, Masuho Y, Matsumoto S. Molecular cloning and characterization of a novel type of histamine receptor preferentially expressed in leukocytes. J Biol Chem. 2000; 275:36781–36786. PMID: 10973974.


10. Sander LE, Lorentz A, Sellge G, Coëffier M, Neipp M, Veres T, Frieling T, Meier PN, Manns MP, Bischoff SC. Selective expression of histamine receptors H1R, H2R, and H4R, but not H3R, in the human intestinal tract. Gut. 2006; 55:498–504. PMID: 16299042.


11. Breunig E, Michel K, Zeller F, Seidl S, Weyhern CW, Schemann M. Histamine excites neurones in the human submucous plexus through activation of H1, H2, H3 and H4 receptors. J Physiol. 2007; 583:731–742. PMID: 17627982.
12. Szurszewsik JH. Johnson LR, editor. Electrical basis for gastrointestinal motility. Prostaglandins and the Gastrointestinal Tract. 1987. New York: Raven Press;p. 383.
13. Huizinga JD, Thuneberg L, Klüppel M, Malysz J, Mikkelsen HB, Bernstein A. W/kit gene required for interstitial cells of Cajal and for intestinal pacemaker activity. Nature. 1995; 373:347–349. PMID: 7530333.


14. Lee HW, Baak CH, Lee MY, Kim YC. Spontaneous contractions augmented by cholinergic and adrenergic systems in the human ureter. Korean J Physiol Pharmacol. 2011; 15:37–41. PMID: 21461239.


15. Ordög T, Ward SM, Sanders KM. Interstitial cells of cajal generate electrical slow waves in the murine stomach. J Physiol. 1999; 518:257–269. PMID: 10373707.
16. Sanders KM. A case for interstitial cells of Cajal as pacemakers and mediators of neurotransmission in the gastrointestinal tract. Gastroenterology. 1996; 111:492–515. PMID: 8690216.


17. Ward SM, Ordog T, Koh SD, Baker SA, Jun JY, Amberg G, Monaghan K, Sanders KM. Pacemaking in interstitial cells of Cajal depends upon calcium handling by endoplasmic reticulum and mitochondria. J Physiol. 2000; 525:355–361. PMID: 10835039.


18. Poli E, Pozzoli C. Histamine H3 receptors do not modulate reflex-evoked peristaltic motility in the isolated guinea-pig ileum. Eur J Pharmacol. 1997; 327:49–56. PMID: 9185835.


19. Korte A, Myers J, Shih NY, Egan RW, Clark MA. Characterization and tissue distribution of H3 histamine receptors in guinea pigs by N alpha-methylhistamine. Biochem Biophys Res Commun. 1990; 168:979–986. PMID: 2161226.
20. Lovenberg TW, Roland BL, Wilson SJ, Jiang X, Pyati J, Huvar A, Jackson MR, Erlander MG. Cloning and functional expression of the human histamine H3 receptor. Mol Pharmacol. 1999; 55:1101–1107. PMID: 10347254.
21. Pozzoli C, Poli E, Costa A, De Ponti F. Absence of histamine H3-receptors in the rabbit colon: species difference. Gen Pharmacol. 1997; 29:217–221. PMID: 9251902.


22. Fargeas MJ, Fioramonti J, Bueno L. Involvement of different receptors in the central and peripheral effects of histamine on intestinal motility in the rat. J Pharm Pharmacol. 1989; 41:534–540. PMID: 2571697.


23. Oishi R, Adachi N, Saeki K. N alpha-methylhistamine inhibits intestinal transit in mice by central histamine H1 receptor activation. Eur J Pharmacol. 1993; 237:155–159. PMID: 8103457.
24. Kim H, Dwyer L, Song JH, Martin-Cano FE, Bahney J, Peri L, Britton FC, Sanders KM, Koh SD. Identification of histamine receptors and effects of histamine on murine and simian colonic excitability. Neurogastroenterol Motil. 2011; 23:949–e409. PMID: 21806740.


25. Koh SD, Sanders KM, Ward SM. Spontaneous electrical rhythmicity in cultured interstitial cells of cajal from the murine small intestine. J Physiol. 1998; 513:203–213. PMID: 9782170.


26. Muller MJ, Prior T, Hunt RH, Rangachari PK. H1 contractile and H2 relaxant receptors in canine gastric muscularis mucosae. Life Sci. 1993; 52:PL49–PL53. PMID: 8429755.
27. Lim HD, van Rijn RM, Ling P, Bakker RA, Thurmond RL, Leurs R. Evaluation of histamine H1-, H2-, and H3-receptor ligands at the human histamine H4 receptor: identification of 4-methylhistamine as the first potent and selective H4 receptor agonist. J Pharmacol Exp Ther. 2005; 314:1310–1321. PMID: 15947036.


28. Campoli-Richards DM, Buckley MM, Fitton A. Cetirizine. A review of its pharmacological properties and clinical potential in allergic rhinitis, pollen-induced asthma, and chronic urticaria. Drugs. 1990; 40:762–781. PMID: 1981354.
29. Koh SD, Jun JY, Kim TW, Sanders KM. A Ca2+-inhibited non-selective cation conductance contributes to pacemaker currents in mouse interstitial cell of Cajal. J Physiol. 2002; 540:803–814. PMID: 11986370.
30. Claro E, Garcia A, Picatoste F. Carbachol and histamine stimulation of guanine-nucleotide-dependent phosphoinositide hydrolysis in rat brain cortical membranes. Biochem J. 1989; 261:29–35. PMID: 2549964.


31. Repka-Ramirez MS. New concepts of histamine receptors and actions. Curr Allergy Asthma Rep. 2003; 3:227–231. PMID: 12662472.


32. Donaldson J, Hill SJ. Histamine-induced hydrolysis of polyphosphoinositides in guinea-pig ileum and brain. Eur J Pharmacol. 1986; 124:255–265. PMID: 3732381.


33. Sakuma I, Gross SS, Levi R. Positive inotropic effect of histamine on guinea pig left atrium: H1-receptor-induced stimulation of phosphoinositide turnover. J Pharmacol Exp Ther. 1988; 247:466–472. PMID: 2846821.
34. Schwartz JC, Arrang JM, Garbarg M, Pollard H, Ruat M. Histaminergic transmission in the mammalian brain. Physiol Rev. 1991; 71:1–51. PMID: 1846044.


35. Kwan HY, Wong CO, Chen ZY, Dominic Chan TW, Huang Y, Yao X. Stimulation of histamine H2 receptors activates TRPC3 channels through both phospholipase C and phospholipase D. Eur J Pharmacol. 2009; 602:181–187. PMID: 19032951.


36. Kiyonaka S, Kato K, Nishida M, Mio K, Numaga T, Sawaguchi Y, Yoshida T, Wakamori M, Mori E, Numata T, Ishii M, Takemoto H, Ojida A, Watanabe K, Uemura A, Kurose H, Morii T, Kobayashi T, Sato Y, Sato C, Hamachi I, Mori Y. Selective and direct inhibition of TRPC3 channels underlies biological activities of a pyrazole compound. Proc Natl Acad Sci U S A. 2009; 106:5400–5405. PMID: 19289841.


37. Bennett A, Whitney B. A pharmacological study of the motility of the human gastrointestinal tract. Gut. 1966; 7:307–316. PMID: 5917417.


38. Bolton TB, Clark JP, Kitamura K, Lang RJ. Evidence that histamine and carbachol may open the same ion channels in longitudinal smooth muscle of guinea-pig ileum. J Physiol. 1981; 320:363–379. PMID: 6275077.


39. Boer K, Helinger E, Helinger A, Pocza P, Pos Z, Demeter P, Baranyai Z, Dede K, Darvas Z, Falus A. Decreased expression of histamine H1 and H4 receptors suggests disturbance of local regulation in human colorectal tumours by histamine. Eur J Cell Biol. 2008; 87:227–236. PMID: 18258331.


40. Bakker RA, Schoonus SB, Smit MJ, Timmerman H, Leurs R. Histamine H(1)-receptor activation of nuclear factor-kappa B: roles for G beta gamma- and G alpha(q/11)-subunits in constitutive and agonist-mediated signaling. Mol Pharmacol. 2001; 60:1133–1142. PMID: 11641442.
41. Wang YX, Kotlikoff MI. Signalling pathway for histamine activation of non-selective cation channels in equine tracheal myocytes. J Physiol. 2000; 523:131–138. PMID: 10673549.


42. Kim BJ, Lim HH, Yang DK, Jun JY, Chang IY, Park CS, So I, Stanfield PR, Kim KW. Melastatin-type transient receptor potential channel 7 is required for intestinal pacemaking activity. Gastroenterology. 2005; 129:1504–1517. PMID: 16285951.


43. Torihashi S, Fujimoto T, Trost C, Nakayama S. Calcium oscillation linked to pacemaking of interstitial cells of Cajal: requirement of calcium influx and localization of TRP4 in caveolae. J Biol Chem. 2002; 277:19191–19197. PMID: 11897792.
44. Lee KP, Jun JY, Chang IY, Suh SH, So I, Kim KW. TRPC4 is an essential component of the nonselective cation channel activated by muscarinic stimulation in mouse visceral smooth muscle cells. Mol Cells. 2005; 20:435–441. PMID: 16404161.
45. Lee YM, Kim BJ, Kim HJ, Yang DK, Zhu MH, Lee KP, So I, Kim KW. TRPC5 as a candidate for the nonselective cation channel activated by muscarinic stimulation in murine stomach. Am J Physiol Gastrointest Liver Physiol. 2003; 284:G604–G616. PMID: 12631560.
46. So KY, Kim SH, Sohn HM, Choi SJ, Parajuli SP, Choi S, Yeum CH, Yoon PJ, Jun JY. Carbachol regulates pacemaker activities in cultured interstitial cells of Cajal from the mouse small intestine. Mol Cells. 2009; 27:525–531. PMID: 19466600.


47. Kim BJ, Chang IY, Choi S, Jun JY, Jeon JH, Xu WX, Kwon YK, Ren D, So I. Involvement of Na+-leak channel in substance P-induced depolarization of pacemaking activity in interstitial cells of Cajal. Cell Physiol Biochem. 2012; 29:501–510. PMID: 22508057.
48. Kurz JB, Perkins JP. Isoproterenol-initiated beta-adrenergic receptor diacytosis in cultured cells. Mol Pharmacol. 1992; 41:375–381. PMID: 1347147.
49. Lohse MJ. Molecular mechanisms of membrane receptor desensitization. Biochim Biophys Acta. 1993; 1179:171–188. PMID: 7692969.


50. Zang WJ, Yu XJ, Honjo H, Kirby MS, Boyett MR. On the role of G protein activation and phosphorylation in desensitization to acetylcholine in guinea-pig atrial cells. J Physiol. 1993; 464:649–679. PMID: 8229823.


51. Kim YC, Kim SJ, Sim JH, Jun JY, Kang TM, Suh SH, So I, Kim KW. Protein kinase C mediates the desensitization of CCh-activated nonselective cationic current in guinea-pig gastric myocytes. Pflugers Arch. 1998; 436:1–8. PMID: 9560440.


52. Jun JY, Choi S, Yeum CH, Chang IY, Park CK, Kim MY, Kong ID, So I, Kim KW, You HJ. Noradrenaline inhibits pacemaker currents through stimulation of beta 1-adrenoceptors in cultured interstitial cells of Cajal from murine small intestine. Br J Pharmacol. 2004; 141:670–677. PMID: 14744802.
53. Jun JY, Choi S, Yeum CH, Chang IY, You HJ, Park CK, Kim MY, Kong ID, Kim MJ, Lee KP, So I, Kim KW. Substance P induces inward current and regulates pacemaker currents through tachykinin NK1 receptor in cultured interstitial cells of Cajal of murine small intestine. Eur J Pharmacol. 2004; 495:35–42. PMID: 15219818.


54. Shahi PK, Choi S, Zuo DC, Yeum CH, Yoon PJ, Lee J, Kim YD, Park CG, Kim MY, Shin HR, Oh HJ, Jun JY. 5-hydroxytryptamine generates tonic inward currents on pacemaker activity of interstitial cells of cajal from mouse small intestine. Korean J Physiol Pharmacol. 2011; 15:129–135. PMID: 21860590.


Fig. 1
Effects of histamine on pacemaker potentials in cultured ICCs from murine small intestine. (A~C) show the pacemaker potentials of ICCs exposed to histamine (10~100µM) in current clamp mode (I=0). Responses to histamine are summarized in (D). Bars represent mean values±SEs. **: p<0.01. Significantly different from untreated controls. CTRL, control.
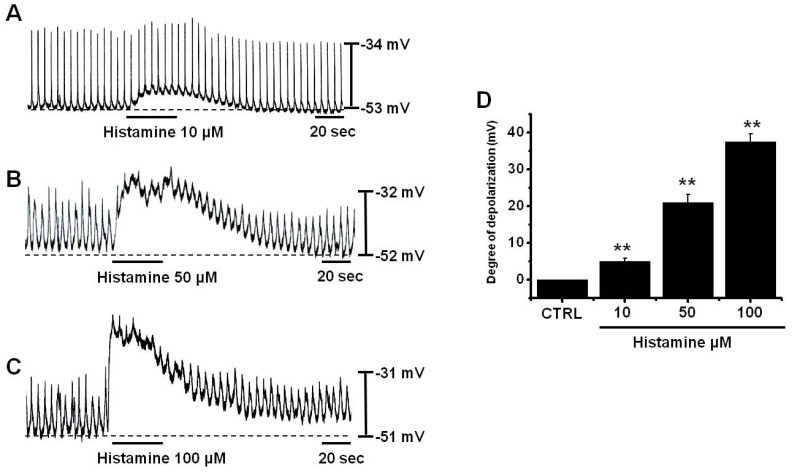
Fig. 2
Effects of histamine receptor subtype agonists and antagonists on histamine-induced pacemaker potential responses in cultured ICCs. (A) Pacemaker potentials of ICCs exposed to 2-PEA (50µM). (B~D) Pacemaker potentials of ICCs exposed to Dimaprit, R-alpha-MeHa, or 4-MH. (E) Pacemaker potentials of ICCs exposed to histamine in the presence of cetirizine (a H1 receptor antagonist). Responses to agonists or antagonists are summarized in (F). Bars represent mean values±SEs. **: p<0.01. Significantly different from untreated controls.
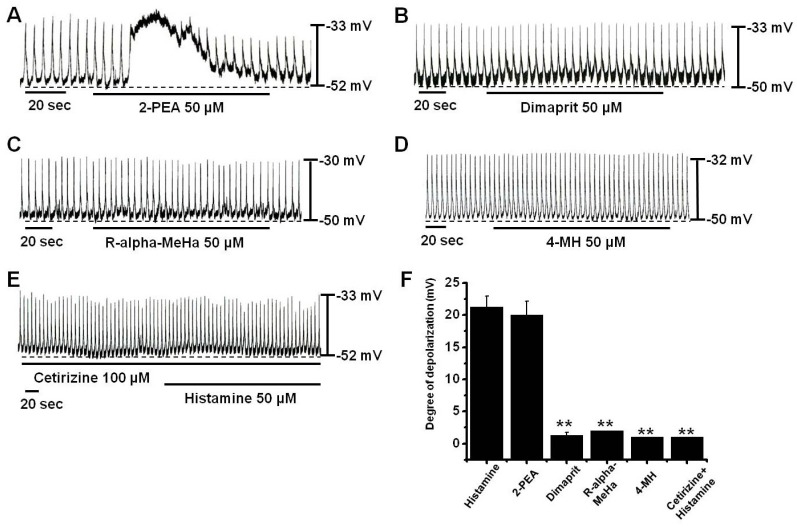
Fig. 3
Effects of an external Ca2+-free solution and of thapsigargin on histamine-induced pacemaker potential responses in cultured ICCs. (A) External Ca2+-free solution abolished the generation of pacemaker potentials, and blocked histamine-induced depolarizations. (B) Thapsigargin (5µM) abolished the generation of pacemaker potentials and also blocked histamine-induced depolarizations. Responses to histamine in external Ca2+-free solution and in the presence of thapsigargin are summarized in (C). Bars represent mean values±SEs. **: p<0.01. Significantly different from untreated controls. CTRL, control.
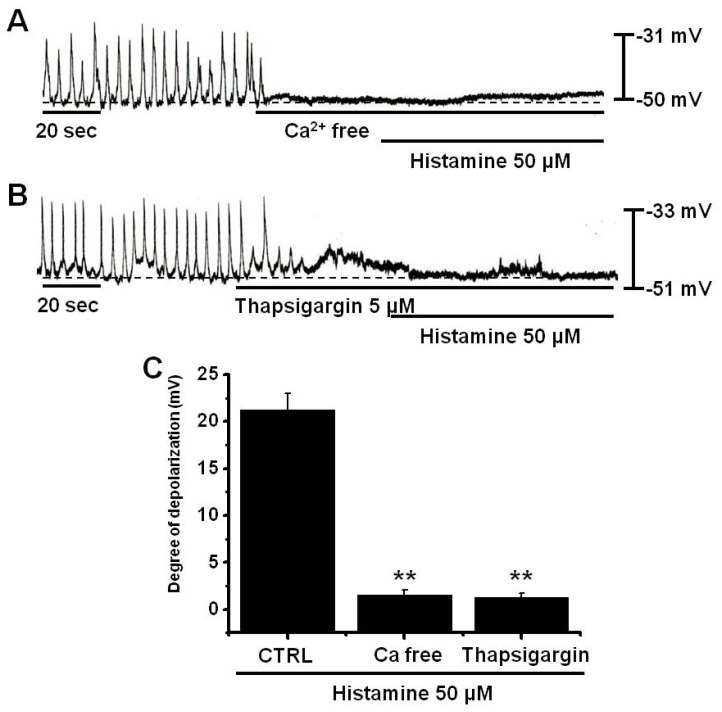
Fig. 4
Effects of PLC, PLD, and PKA inhibitors on histamine-induced pacemaker potential responses in cultured ICCs. (A) Pacemaker potentials of cultured ICCs exposed to histamine (50µM) in the presence of 5µM U-73122 (a PLC inhibitor). (B) Pacemaker potentials of cultured ICCs exposed to histamine in the presence of 1µM FIPI (a PLD inhibitor). (C) Pacemaker potentials of ICCs exposed to histamine in the presence of 1µM KT5720 (a PKA inhibitor). Responses to histamine in the presence of different inhibitors are summarized in (D). Bars represent mean values±SEs. **: p<0.01. Significantly different from untreated controls. CTRL, control.
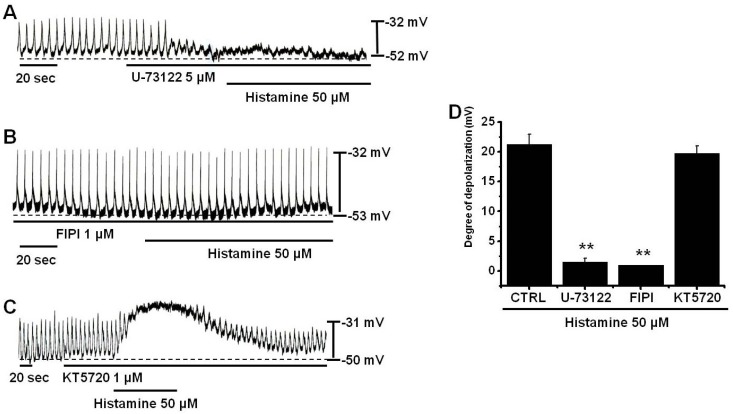
Fig. 5
Effects of specific TRPC3 inhibitors on histamine-induced pacemaker potential responses in cultured ICCs. (A) Pacemaker potentials of cultured ICCs exposed to histamine (50µM) in the presence of 2µM pyr3 (a specific TRPC3 inhibitor). Responses to histamine in the presence of pyr3 are summarized in (B). Bars represent mean values±SEs. **: p<0.01. Significantly different from untreated controls. CTRL, control.
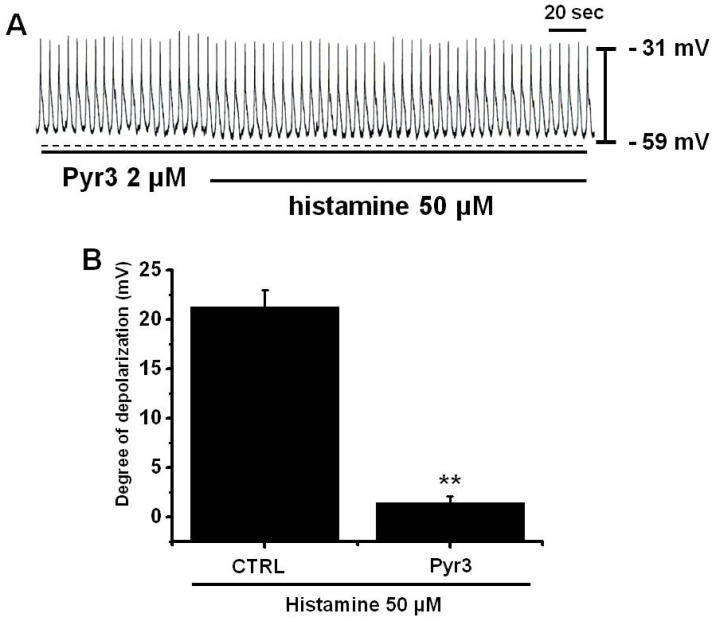