Abstract
Hypoxic pulmonary vasoconstriction (HPV) is physiologically important response for preventing mismatching between ventilation and perfusion in lungs. The HPV of isolated pulmonary arteries (HPV-PA) usually require a partial pretone by thromboxane agonist (U46619). Because the HPV of ventilated/perfused lungs (HPV-lung) can be triggered without pretone conditioning, we suspected that a putative tissue factor might be responsible for the pretone of HPV. Here we investigated whether HPV can be also observed in precision-cut lung slices (PCLS) from rats. The HPV in PCLS also required partial contraction by U46619. In addition, K+ channel blockers (4AP and TEA) required U46619-pretone to induce significant contraction of PA in PCLS. In contrast, the airways in PCLS showed reversible contraction in response to the K+ channel blockers without pretone conditioning. Also, the airways showed no hypoxic constriction but a relaxation under the partial pretone by U46619. The airways in PCLS showed reliable, concentration-dependent contraction by metacholine (EC50, ~210 nM). In summary, the HPV in PCLS is more similar to isolated PA than V/P lungs. The metacholine-induced constriction of bronchioles suggested that the PLCS might be also useful for studying airway physiology in situ.
Different from vasodilatory responses of systemic arteries (e.g. coronary and renal arteries) to hypoxia, pulmonary arteries constrict during alveolar hypoxia in a response known as hypoxic pulmonary vasoconstriction (HPV). HPV directs blood flow from hypoxic region toward well-ventilated areas of the lung, thereby optimizing gas exchange. HPV responses have been measured in whole lung (pulmonary arterial pressure increase by hypoxic ventilation), isolated pulmonary artery (PA, isometric contraction by hypoxia), and isolated pulmonary artery smooth muscle cells (PASMCs, changes in ion channel activity and [Ca2+]c by hypoxia), indicating that the O2-sensing machinery is intrinsic to PASMCs. However, the mechanism by which PASMCs detect hypoxia and transduce this into contraction is not fully understood.
Various electrophysiological models and signaling pathways have been suggested to explain the underlying mechanisms for HPV. Among them, hypoxic inhibition of K+ channels and subsequent depolarization-induced activation of L-type Ca2+ channel has been a major hypothesis. However, some previous studies including our recent one showed that a pharmacological inhibition of K+ channel alone could not induce the isometric contraction in isolated pulmonary arteries [1-3]. In the isolated PA, a partial pretone induced by vasoactive agent (e.g. thromboxane A2 or PGF2α) is required to show contractile responses to hypoxia [4-6]. Our previous study also showed that the HPV of isolated PA (HPV-PA) requires pretreatment with U46619, a TXA2 analogue. Also, an application of K+ channel blocker did induce strong contraction of PA under the pretone induced by U46619 [3].
Different from HPV-PA, the hypoxia-induced increase in pulmonary arterial pressure (PAP) of ventilated/perfused (V/P) lungs does not require a pretreatment with TXA2 or other agonists. Although addition of angiotensin II (Ang II) or red blood cells in the perfusate augment or stabilize the HPV response of V/P lungs, the increase in PAP by hypoxia per se was consistently observed. Furthermore, in isolated PAs, the pretreatment with Ang-II was not an effective pretone condition for the HPV-PA. Such differential responses between PA and V/P lungs indicate that some diffusible factors from lung parenchyma might affect the HPV. To address this issue, different type of experimental model is requested.
Viable tissue slices of uniform thickness, precision-cut tissue slices, can be taken into biomedical experiments. The precision-cut slices of liver and brain have been widely used in toxicology and neurophysiology, respectively. For the relatively soft tissue like lung, an agarose gel instilling is necessary to provide appropriate solidity of tissue cores [7]. The precision-cut lung slices (PCLS) can be cultured for 48 h [8-10], and have been successfully utilized in pharmacological studies of airway contractility [11-15]. However, there are only a few trials of applying PCLS technique to investigate the physiological responses of PA, such as HPV [10,16,17].
Although the V/P lung model is a physiologically more relevant than isolated PA, the flexibility of experimental condition is limited. In the lung slices, it is suggested that the influence from lung parenchyma might be preserved while allowing the solution exchange and variety of experimental protocols (e.g. tissue culture). Therefore, to overcome the drawbacks of the above experimental methods, we aimed to test the feasibility of PCLS technique for studying HPV in rat and mouse lungs. More specifically, we investigated whether the partial pretone by U46619 (TXA2 agonist) is also requested for the HPV of PA in the lung slices. In addition, it was aimed to compare the effects of agonists and K+ channel inhibitors between PAs and airways (AWs).
This investigation was performed in compliance with the Guide for the Care and Use of Laboratory Animals published by the US National Institutes of Health (NIH Publication NO. 85-23, Revised 1996) and was approved by the IACUC of the Seoul National University College of Medicine. After decapitation, the lungs were rapidly removed from the fully anesthesized Male Sprague-Dawley rats (250~300 g) and moved into normal tyrode (NT) solution. The second- and third-order branches of PAs (diameter: 200~300 µm) were isolated and cut into 2.0 mm-long ring without removing endothelium. Using two 25 µM tungsten wires, a segment of artery was mounted on a Mulvany-type myograph (Myo-Interface model 410A; DMT, Aarhus, Denmark), superfused with PSS solution and continuously gassed with 74% N2, 21% O2, and 5% CO2 at 37℃. A basal tension was set as 0.5 g and 80 mM KCl-PSS (80K contraction) was applied to evaluate the viability of PAs.
Male Sprague-Dawley rats (250~300 g) were anaesthetized with pentobarbital sodium (40 mg/kg, i.p.), and ventilated (Ventilator 683, Harvard Apparatus, USA) via cannulated trachea with normoxic gas (21% O2, 5% CO2, 74% N2). Stable ventilation (85 breaths min-1) with regular tidal volume (10 ml/kg) was maintained. After median sternotomy, a suture was placed around the ascending aorta and the main pulmonary artery. After injection of heparin (200 U/kg) into the right ventricle, cannulations were made into the pulmonary artery via right ventricle and into the left atrium via the left ventricle, and then perfused at a constant flow of 15 ml/min (Servo amplifier 2990, Harvard Apparatus, USA). The recycling perfusate consisted of 20 ml of whole blood and 30 ml of PSS. The PAP was measured at the inflow cannula and digitally stored using Powerlab/4ST and Chart5 (ADInstruments). The lungs were exposed to cycles of normoxia (5 min) and hypoxia (5 min) through ventilation with gas containing 21% or 3% O2 with balance made up by N2.
Lungs were taken from male Sprague-Dawley rats (250~300 g) under full anaesthesia (see above). After injection of pentobarbital (40 mg/kg), the trachea was cannulated and ventilation was started with a tidal volume of 10 ml/kg at 80 breaths/min. After laparotomy, the diaphragm was removed and heparin (200 IU) was injected into the right ventricle. The animals were exsanguinated by cutting the vena cava. Both lungs were perfused with NT solution via a catheter placed in the pulmonary artery until the lungs were free of blood. Then, the lungs were filled with a low melting-point agarose solution (1.5% in MEM, 44 ml/kg) and the heart and the lungs were rapidly removed en bloc. In order to solidify the agarose and harden them for cutting, the lungs were placed on ice for 10 min. Tissue slices (220 µm) were prepared using a Vibratome VT1200 (Leica Microsystems GmbH, Wetzlar, Germany). Then, the lung slices were incubated at 37℃ in a humid atmosphere in minimal essential medium (pH 7.2). The medium was changed every 30 min during the first 2 h followed by a change every 1 h for the next 2 h, in order to remove the agarose and cell debris from the tissue.
The prepared lung slice was transferred to PCLS chamber (Harvard Apparatus, Holliston, MA, USA) on the stage of an inverted microscope (IX70, Olympus, Japan) and warmed to 37℃. PAs and AWs were focused, imaged with Cascade 650 CCD camera (Roper Scientific, USA) controlled by Metafluor software (Universal Imaging, Downingtown PA, USA). After preincubation for 10 min, the first image was acquired. The PAs and AWs were imaged every 0.5 minute. The images were analysed by Metafluor software (Universal Imaging, Downingtown, PA, USA). The area of the AW and PA lumen was calculated, with respect to time, by pixel summing. Control luminal area was defined as 100%.
The NT solution consists of the following composition (in mM): NaCl 143, KCl 5.4, NaH2PO4 0.33, CaCl2 1.8, MgCl2 0.5, 4-(2-hydroxyethyl)-1-piperazineethanesulfonic acid (HEPES) 10, and glucose 10 (pH 7.4 with NaOH). The PSS for myograph experiment consisted of the following composition (in mM): NaCl 118, KCl 4, NaH2PO4 0.44, NaHCO3 24, CaCl2 1.8, MgSO4 1 and Glucose 5.6. The PSS for V/P lung model experiment consisted of the following composition (in mM): NaCl 131, KH2PO4 1.2, NaHCO3 22.6, CaCl2 3.2, MgSO4 1.2, Sodium meclofenamate monohydrate 0.0050 g/l, glucose 11 and BSA 30.0 g/l. Isotonic high K+ solutions (80 mM) were prepared by replacing NaCl with an equimolar amount of KCl in PSS. BSA was purchased from Tocris (Ellisville, MO, USA). The other drugs including tetraethylammonium (TEA) and 4-aminopyridine (4-AP) were obtained Sigma (St. Louis, MO, USA).
The data are presented as original recordings and bar graphs of mean±SEM. Statical analysis was performed by paired or unpaired Student's t-test or a one-way analysis of variance (ANOVA) and the Bonferroni multiple range tests as appropriate. p<0.05 was considered as a statistical significance.
Firstly, we confirmed the requirement of pretone agent for the HPV-PA. The isometric tension of rat PA was increased by hypoxia (3% PO2) under pretreatment with 5~10 nM U46619. In contrast, hypoxia alone had no effect on the basal tone of PA (Fig. 1A, C). Different from the isolated PA, hypoxic increase in the PAP of V/P lungs was observed without pretreatment with U46619 while the amplitude of HPV was augmented by pretreatment with angiotensin II (Ang II) or with U46619 (Fig. 1D, E, n=3, respectively). The same pattern of contrasting requirement for the pretone condition has been consistently observed in our previous studies [3,6].
Next we investigated the contractility of PAs and AWs in PCLS from rats. As shown in a representative image of PCLS (Fig. 2A), PA and AW usually located together, and could be discriminated from their morphological characteristics; the luminal area of AW was larger than the paired PA, and the wall of PA appeared to be thicker than that of AW (Fig. 2A). The membrane depolarization by raising extracellular [K+] (60K) commonly induced contraction of both PA and AW (Fig. 2B, n=8). The responses to muscarinic stimulation (ACh) provided reliable functional discrimination between PA and AW; on application of ACh, AWs showed reversible constriction whereas PAs did not show any response (Fig. 2B, upward arrow). Interestingly, responses to K+ channel blockers (4-AP and TEA) were also different between AW and PA. Both 4-AP and TEA induced significant contraction of AW whereas neither of them had effect on PA (Fig. 2B, n=5).
Next we compared the responses of AWs and PAs to hypoxia. PAs did not show contractile response to hypoxia alone. However, HPV was observed under a partial pretone induced by 5 nM U46619, a low concentration of TXA2 receptor agonist (Fig. 3, n=3). Interestingly, the contractile responses to the K+ channel blockers, 4-AP and TEA, were also found with U46619 pretreatment (Fig. 3B, C, n=3).
In AWs, the pretreatment with 5 nM U46619 also induced a partial contraction with oscillatory changes, which was not augmented by additional hypoxia. In some cases, oscillatory contractions were induced by 5 nM U46619, and the additional hypoxia abolished the oscillatory contractions (Fig. 4, n=3).
We also tested whether the PCLS can be also applied for investigating HPV in mouse lungs. For reasons not clear yet, the changes in the luminal area of PA of mouse PCLS were technically more difficult to visualize than those of rat PCLS. The mouse PAs usually showed collapsed contour with hardly discernable luminal space (Fig. 5A). In contrast, the AW of mouse PCLS was consistently observed with reversible contractions in response to muscarinic agonist. The contractility of AW to muscarinic agonists is the basis of 'metacholine (MCh) provocation test' by which the airway resistance of asthmatic patients is evaluated. Here we tested whether concentration-dependent contraction of AW can be reliably tested in PCLS. In the mouse PCLS, after confirming the contraction by 60K-induced depolarization, various concentrations of MCh were applied with 5 min of interval for washout (Fig. 5B). AW showed reversible contractions to MCh and the summarized results are shown (Fig. 5C, n=4).
In the present study, the usability of PCLS technique for studying HPV was investigated and compared with the hypoxic responses of isolated PA and V/P lungs. After simultaneous recordings of the serial images of PA and AW, their luminal areas could be compared for investigation of differential responses to various conditions. The contractions of PA and AW in PCLS were almost completely reversible by washout with control solution. Therefore, the PCLS technique could be applied to pharmacological studies repetitively testing the effects on the contractility of PA and AW.
Because multiple slices could be obtained from a lung, this technique could also reduce the number of animals sacrificed for pharmacological studies. Between PA and AW in the same area of PCLS, the luminal area of AW was usually larger than that of PA, and therefore, the utility of PCLS for studying AW seems better for PA. Furthermore, in mouse PCLS, the luminal area of PA was usually too small for quantitative analysis, and were not available for studies. In contrast, the AW of mouse PCLS showed reliable concentration-dependent contractions to MCh (Fig. 5). This result clearly demonstrates the utility of PLCS for testing bronchodilatory agents or for investigating the pathophysiological agents in airway obstruction disease models of animal. The EC50 values of MCh for the contraction of mouse AW was between 200~250 nM irrespective of the criteria for the normalization (Fig. 5C), and these were smaller than the EC50 of Wistar rats PCLS [18].
The main question addressed in this study was whether the pretreatment with TXA2 agonist (U46619) is necessary for the HPV in PCLS. Different from the HPV of isolated PA, the HPV in perfused lungs was consistently induced without U46619 (Fig. 1), which suggested that a putative 'tissue factor' might substitute the effect of U46619. In the present study, we initially supposed that the PA in PCLS might behave like the PA in V/P lungs. However, the requirements of U46619 pretreatment for HPV and for the 4AP-induced contraction in PCLS were in common with the properties of isolated PA. These results suggested that the hypothetical tissue factor(s) in the V/P lungs, substituting the pretone effect of U46619 in the isolated PA study, might be insufficiently released or diluted in the experimental conditions for PCLS.
The critical role of TXA2 agonist for HPV has been investigated in our recent study, and the activation of nonselective cationic conductance was suggested as a permissive mechanism for inducing contraction of PA by the reduced K+ current under hypoxia [3]. It is widely accepted that the inhibition of K+ channel, especially voltage-gated K+ channel (Kv), is a crucial step mediating the PO2 change into electrophysiological excitation of PASMC. However, considering the threshold membrane potential for activating Kv, partial depolarization and subsequent activation of Kv would be a prerequisite to reveal the effect of K+ channel inhibition by hypoxia. Then, the activation of L-type Ca2+ channels would induce PA contraction. Such 'three-step hypothesis' for HPV seemed to be mimicked by applying 4AP, a Kv inhibitor, under the pretreatment with U46619 as shown in PCLS (Fig. 3).
In contrast to the lack of contractile response of PA to the K+ channel blocker alone, AW in the same PCLS showed consistent contractions by both 4AP and TEA. Such results indicate that the resting membrane potential of AW smooth muscle cells would be above the threshold voltage of Kv activation. It was notable that hypoxia induced a relaxing response (disappearance of oscillatory contractions) of U46619-pretreated AW, which was in opposite to the HPV response. While HPV is a physiological mechanism to prevent ventilation-perfusion mismatch, a hypoxic constriction of AW might aggravate and expand the unventilated area of lungs. In this respect, the hypoxic relaxation of AW could be a protective mechanism against focal atelectasis [19].
In summary, here we confirmed the feasibility of PCLS technique for the physiological and pharmacological investigation of PA and AW in rodents. The requirement of U46619-induced pretone for hypoxic contraction of PA in PCLS was in common with the property of isolated PA. Since PLCS can be kept in a tissue culture condition for up to 72 hours [18], this technique would be used to investigate the effects of sustained in vitro conditions on the contractility of PA and AW.
ACKNOWLEDGEMENTS
This research was supported by the Basic Science Research Program through the National Research Foundation of Korea (NRF) funded by the Ministry of Education, Science and Technology (NRF 2009-0066749, 2011-0017370 and 2011-0027739) and by Seoul National University Hospital (SNUH) Research Fund (No. 03-2011-0020).
References
1. Bonnet S, Dubuis E, Vandier C, Martin S, Marthan R, Savineau JP. Reversal of chronic hypoxia-induced alterations in pulmonary artery smooth muscle electromechanical coupling upon air breathing. Cardiovasc Res. 2002; 53:1019–1028. PMID: 11922912.


2. Osipenko ON, Alexander D, MacLean MR, Gurney AM. Influence of chronic hypoxia on the contributions of non-inactivating and delayed rectifier K currents to the resting potential and tone of rat pulmonary artery smooth muscle. Br J Pharmacol. 1998; 124:1335–1337. PMID: 9723941.


3. Yoo HY, Park SJ, Seo EY, Park KS, Han JA, Kim KS, Shin DH, Earm YE, Zhang YH, Kim SJ. Role of thromboxane A2-activated nonselective cation channels in hypoxic pulmonary vasoconstriction of rat. Am J Physiol Cell Physiol. 2012; 302:C307–C317. PMID: 21998141.


4. Leach RM, Robertson TP, Twort CH, Ward JP. Hypoxic vasoconstriction in rat pulmonary and mesenteric arteries. Am J Physiol. 1994; 266:L223–L231. PMID: 8166292.


5. Rodman DM, Yamaguchi T, O'Brien RF, McMurtry IF. Hypoxic contraction of isolated rat pulmonary artery. J Pharmacol Exp Ther. 1989; 248:952–959. PMID: 2467984.
6. Park SJ, Yoo HY, Earm YE, Kim SJ, Kim JK, Kim SD. Role of arachidonic acid-derived metabolites in the control of pulmonary arterial pressure and hypoxic pulmonary vasoconstriction in rats. Br J Anaesth. 2011; 106:31–37. PMID: 20935003.


7. Sanderson MJ. Exploring lung physiology in health and disease with lung slices. Pulm Pharmacol Ther. 2011; 24:452–465. PMID: 21600999.


8. Struckmann N, Schwering S, Wiegand S, Gschnell A, Yamada M, Kummer W, Wess J, Haberberger RV. Role of muscarinic receptor subtypes in the constriction of peripheral airways: studies on receptor-deficient mice. Mol Pharmacol. 2003; 64:1444–1451. PMID: 14645675.


9. Moreno L, Perez-Vizcaino F, Harrington L, Faro R, Sturton G, Barnes PJ, Mitchell JA. Pharmacology of airways and vessels in lung slices in situ: role of endogenous dilator hormones. Respir Res. 2006; 7:111. PMID: 16923180.


10. Paddenberg R, König P, Faulhammer P, Goldenberg A, Pfeil U, Kummer W. Hypoxic vasoconstriction of partial muscular intra-acinar pulmonary arteries in murine precision cut lung slices. Respir Res. 2006; 7:93. PMID: 16808843.


11. Bai Y, Sanderson MJ. Modulation of the Ca2+ sensitivity of airway smooth muscle cells in murine lung slices. Am J Physiol Lung Cell Mol Physiol. 2006; 291:L208–L221. PMID: 16461427.
12. Martin C, Uhlig S, Ullrich V. Cytokine-induced bronchoconstriction in precision-cut lung slices is dependent upon cyclooxygenase-2 and thromboxane receptor activation. Am J Respir Cell Mol Biol. 2001; 24:139–145. PMID: 11159047.


13. Bergner A, Sanderson MJ. Acetylcholine-induced calcium signaling and contraction of airway smooth muscle cells in lung slices. J Gen Physiol. 2002; 119:187–198. PMID: 11815668.


14. Brueggemann LI, Kakad PP, Love RB, Solway J, Dowell ML, Cribbs LL, Byron KL. Kv7 potassium channels in airway smooth muscle cells: signal transduction intermediates and pharmacological targets for bronchodilator therapy. Am J Physiol Lung Cell Mol Physiol. 2012; 302:L120–L132. PMID: 21964407.


15. Ressmeyer AR, Larsson AK, Vollmer E, Dahlèn SE, Uhlig S, Martin C. Characterisation of guinea pig precision-cut lung slices: comparison with human tissues. Eur Respir J. 2006; 28:603–611. PMID: 16737991.


16. Desireddi JR, Farrow KN, Marks JD, Waypa GB, Schumacker PT. Hypoxia increases ROS signaling and cytosolic Ca2+ in pulmonary artery smooth muscle cells of mouse lungs slices. Antioxid Redox Signal. 2010; 12:595–602. PMID: 19747064.
17. Held HD, Martin C, Uhlig S. Characterization of airway and vascular responses in murine lungs. Br J Pharmacol. 1999; 126:1191–1199. PMID: 10205008.


18. Martin C, Uhlig S, Ullrich V. Videomicroscopy of methacholine-induced contraction of individual airways in precision-cut lung slices. Eur Respir J. 1996; 9:2479–2487. PMID: 8980957.


19. Wetzel RC, Herold CJ, Zerhouni EA, Robotham JL. Hypoxic bronchodilation. J Appl Physiol. 1992; 73:1202–1206. PMID: 1400037.


Fig. 1
Essential role of TXA2 on HPV in PAs. (A) Hypoxia (3% Po2) alone did not induce an increased tension of PAs. (B) In the presence of U46619 (10 nM), a strong contraction of PAs occurred in response to hypoxia. (C) Summaries of PAs tone normalized to 80K-induced contraction were shown as a bar graph (mean±SEM, n=7). *Indicates statistically significant difference from the control value (p<0.05). (D) Induction of HPV in V/P lungs. The increase in pulmonary arterial pressure (PAP, mmHg) was observed by hypoxic ventilation. In the same lung, Ang II induced a transient PAP increase, which augmented the HPV response. (E) A pretreatment with U46619 also induced a transient PAP increase, and HPV was also observed afterwards.
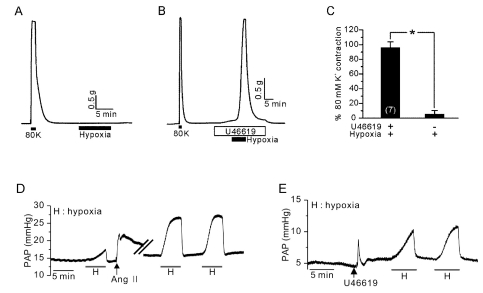
Fig. 2
Identification of PA and AW constrictions in rat PCLS. (A) A representative image of rat PCLS demonstrating the AW and PA. (B) Functional discrimination of PA and AW according to differential responses to ACh and K+ channel blockers. AW showed strong constriction to ACh (10 µM), tetraethylammonium (TEA, 2 mM) and 4-aminopyridine (4AP, 5 mM) while PA showed no response to the concomitant application of the above agents.
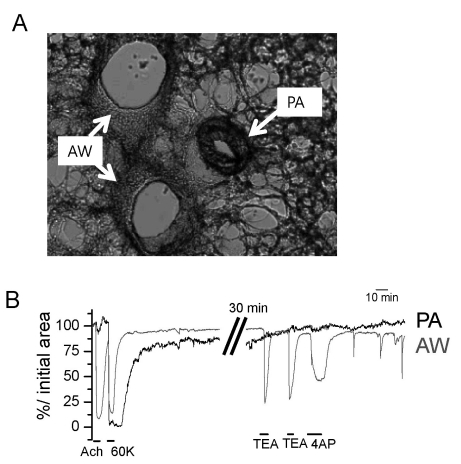
Fig. 3
Responses of PA to hypoxia and K+ channel blockers under pretone conditions in rat PCLS. Three representative cases of relative luminal area (% initial area) changes in PAs. In all cases (A~C), the K+ channel blockers (TEA and 4AP) induced reversible constriction only under the pretreatment with 5 nM U46619 while not with 100 nM Ang II (B). Also, the hypoxic constriction was observed under the pretreatment with 5 nM U46619 (A, C).
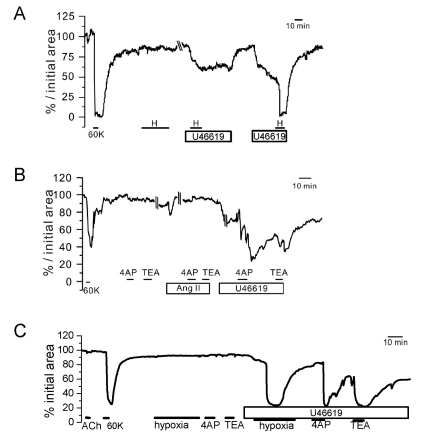
Fig. 4
Responses of AW to hypoxia and K+ channel blockers under pretone conditions in rat PCLS. Two representative cases of luminal area (% initial area) changes in AWs. The strong contractile response to 10 µM ACh was confirmed along with the response to 60 K. Hypoxia alone had no effect (A) or induced a slight dilation (B) of AW. Application of 5 nM U46619 induced oscillatory or tonic constrictions that were not affected (A) or partly inhibited by hypoxia (B). Different from PAs, both 4AP and TEA induced constriction of AW in the absence of U46619 pretreatment.
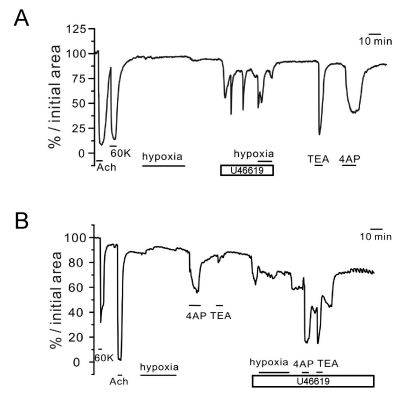
Fig. 5
Response of AW to metacholine (MCh) in mouse PCLS. (A) A representative image of rat PCLS demonstrating the AW and PA. Note the relatively narrow lumen of PA in the mouse PCLS. (B) Concentration-dependent reversible constrictions of mouse AW. The maximum constriction induced by 100 µM MCh was markedly larger (>five fold) than the 60K-constriction. (C) Normalized AW constrictions to different concentrations of MCh were averaged and fitted to logistic functions. Each panel was obtained by different type of normalization criteria; relative change from the maximum luminal area (left), normalized against 60K constriction (middle), and normalized to maximum constriction by 100 µM MCh (right). Regardless of the normalization criteria, the half-effective concentration (EC50) of MCh located at similar ranges.
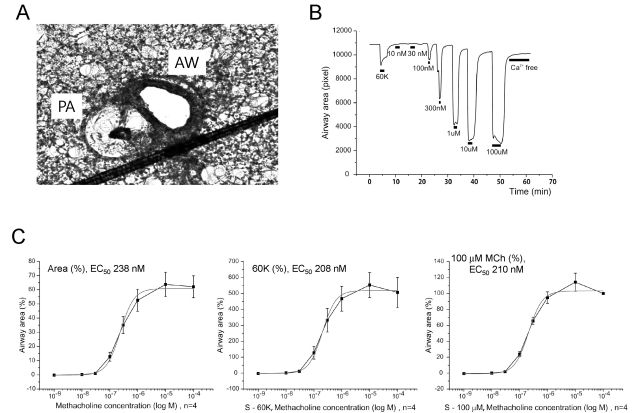