Abstract
In this study, we focused to identify whether eupatilin (5,7-dihydroxy-3',4',6-trimethoxyflavone), an extract from Artemisia argyi folium, prevents H2O2-induced injury of cultured feline esophageal epithelial cells. Cell viability was measured by the conventional MTT reduction assay. Western blot analysis was performed to investigate the expression of 5-lipoxygenase by H2O2 treatment in the absence and presence of inhibitors. When cells were exposed to 600 µM H2O2 for 24 hours, cell viability was decreased to 40%. However, when cells were pretreated with 25~150 µM eupatilin for 12 hours, viability was significantly restored in a concentration-dependent manner. H2O2-treated cells were shown to express 5-lipoxygenase, whereas the cells pretreated with eupatilin exhibited reduction in the expression of 5-lipoxygenase. The H2O2-induced increase of 5-lipoxygenase expression was prevented by SB202190, SP600125, or NAC. We further demonstrated that the level of leukotriene B4 (LTB4) was also reduced by eupatilin, SB202190, SP600125, NAC, or nordihydroguaiaretic acid (a lipoxygenase inhibitor) pretreatment. H2O2 induced the activation of p38MAPK and JNK, this activation was inhibited by eupatilin. These results indicate that eupatilin may reduce H2O2-induced cytotoxicity, and 5-lipoxygenase expression and LTB4 production by controlling the p38 MAPK and JNK signaling pathways through antioxidative action in feline esophageal epithelial cells.
Reactive oxygen species (ROS) are forms of oxygen that are produced by the incomplete reduction of molecular oxygen [1]. ROS participate and regulate diverse downstream signaling pathways leading to specific cellular functions such as growth, metabolic rate, cell division, necrosis, apoptosis and the aging process [2,3]. Although low levels of ROS play an important role in physiological functions, several studies have reported that high concentrations of ROS result in oxidative damage and induce cytotoxic effects in cells [4,5]. Since hydrogen peroxide, one type of ROS, has remarkable membrane permeability [6], exposure of cells to exogenous hydrogen peroxide (H2O2) can induce detrimental effects [7]. From these processes, further oxidative stimulation will be propagated, damaging cellular molecules, and thus contributing to inflammation, aging, and cancer [8-10]. Increased mucosal concentrations of ROS have been implicated in the generation of gastrointestinal diseases, including acid-related peptic diseases and inflammatory disorders [11]. However, cell damage caused by free radicals in gastric or esophageal mucosa can be prevented by the administration of free radical scavengers [12,13].
As seen in one study, an ischemic-like injury by oxygen-glucose deprivation activated 5-lipoxygenase mediated by oxidative stress through the p38 mitogen-activated protein kinase (MAPK) pathway in PC12 cells [14]. 5-Lipoxygenase (5-LOX) is a key enzyme within the arachidonic acid cascade [15]. 5-LOX catalyzes oxygenation of arachidonic acid, and produces 5-hydroperoxyeicosatetraenoic acid (5-HPETE) and leukotrienes [16]. Leukotrienes (LT) play important roles in the inflammatory pathophysiologic process. LTB4, a subtype of LT, is a potent chemoattractant for neutrophils, eosinophils and monocytes including adherence of phagocytes to vessel walls, neutrophil degranulation as well as the release of superoxide anions [17]. Considering the potent pro-inflammatory properties of LTB4, the modulation of 5-LOX and LTB4 is of interest in the treatment of numerous diseases such as inflammatory disorders [18,19].
Werz and Steinhilber [17] observed that p38 MAPK activation by cell stress is required for efficient leukotriene synthesis in B-lymphocytes. MAPK pathways are critical for converting diverse extracellular signals, including ROS, to biological responses. MAPKs modulate many cellular processes, such as gene induction, cell survival/apoptosis, as well as cellular stress and inflammatory responses [20]. ERK1/2 behave mainly as mitogen-activated proliferation/differentiation factors [21], whereas JNK and p38 MAP kinase are mainly stress-activated proteins related to apoptotic cell death [22]. Based on these evidences, in the present study, we tested the roles of 5-LOX and MAPKs in external H2O2 stimulation with esophageal epithelial cells.
On the other hand, flavonoids, which are secondary metabolites in plants, are considered relatively non-toxic bioactive substances and have diverse biological effects, such as anti-inflammatory, anti-oxidant, anti-allergic, hepatoprotective, anti-thrombotic, anti-viral, and anti-carcinogenic activities [23,24]. Thus, these activities may explain the beneficial effects of flavonoid intake in different human pathologies, such as hypertension, inflammatory conditions, and even cancer [25]. In the present study, we used eupatilin (5,7-dihydroxy-3'4',6-trimethoxyflavone) being one of the pharmacologically active flavonoid components of Stillen to test the protective potential of flavonoids. Stillen (Artemisia herba extract) is a quality-controlled compound extracted from Artemisiae argyi folium, a traditional Korean herbal medicine for the treatment of abdominal tenderness, bloody diarrhea, and gynecological disorders. It shows muco-protective activity against noxious agents and exhibits favorable effects in experimental models of gastrointestinal disease such as gastritis, peptic ulcer, inflammatory bowel disease, and pancreatitis. Eupatilin has a potent antigastritic effect [26]. Eupatilin is reported to exert strong anti-inflammatory, and anti-oxidative activity as well as cytoprotective effects against experimentally induced gastrointestinal, hepatic, and pancreatic damage in vivo and in vitro [27-30].
Therefore, the purpose of this study is to investigate the anti-inflammatory potential and the cytoprotective mechanism of eupatilin on the H2O2-activated 5-LOX and LTB4 production in feline esophageal epithelial cells.
Eupatilin was graciously provided by Dong-A Pharmaceutical Co., Ltd. (Yong-In, Korea) and dissolved in 0.1% dimethyl sulfoxide (DMSO). H2O2, bovine serum albumin (BSA), 4-(2-hydroxyethyle)-1-piperazine-N'-2-ethane sulfonic acid (HEPES), leupeptin, aprotinin, β-mercaptoethanol, ethylene glycol-bis-(β-aminoethylether)-N,N,N',N'-tetraacetic acid (EGTA), ethylenediamine tetra acetic acid (EDTA), phenylmethyl-sulfonylfluoride (PMSF), thiazolyl blue tetrazolium bromide, Hank's Balanced Salt Solution-Modified (HBSS), NAC and NDGA were purchased from Sigma Chemical Co. (St. Louis, MO, USA); Fetal bovine serum (FBS), Antibiotic-Antimycotic (penicillin, streptomycin, amphotericin B), and trypsin-EDTA from Invitrogen (Grand Island, NY, USA); Dulbecco's modified Eagle's medium (DMEM), and phosphate-buffered saline (PBS) from Welgene Inc. (Daegu, South Korea); SB202190 and SP600125 from Calbiochem (San Diego, CA, USA); 5-LOX, phospho-SAPK/JNK, SAPK/JNK, phospho-p38 MAPK, and p38 MAPK antibodies from Cell Signaling Technology (Beverly, MA, USA); Actin antibody from Santa Cruz Biotechnology (Santa Cruz, CA, USA); goat anti-rabbit IgG-HRP, goat anti-mouse IgG-HRP, and rabbit anti-goat IgG-HRP from Zymed Laboratories Inc. (Eccles Avenue, CA, USA); Rainbow prestained molecular weight marker from Amersham (Arlington Heights, IL, USA); Enhanced Chemiluminescence (ECL) agents from PerkinElmer Life Sciences (Boston, MA, USA); Ammonium persulfate, N,N,N',N'-tetramethylethylene diamine (TEMED), nitrocellulose (NC) membrane, Tris/Glycine/SDS buffer, Tris/Glycine buffer, and 30% acrylamide/bis solution from BioRad (Richmond, CA, USA); RestoreTM Western Blot Stripping Buffer from Pierce (Rockford, IL, USA); LTB4 EIA kit from Cayman Chemical Company (Ann Arbor, MI, USA).
All animal experiments were approved by the Institutional Animal Care and Use Committee of Chung-Ang University, in accordance with the guide regarding the Care and Use of Laboratory Animals in Seoul, Korea. Adult cats of either sex weighing between 2.5 and 3.5 kg were anesthetized with Zoletil 50 (12.5 mg/0.25 ml/kg), which was composed of tiletamine and zolazepam, and euthanized with an overdose of 25% urethane (Aldrich, St. Louis, MO, USA). After the abdomen was opened with a midline incision, the esophagus was excised, cleaned and maintained in Krebs buffer composed of 116.6 mM NaCl, 21.9 mM NaHCO3, 1.2 mM NaH2PO4, 3.4 mM KCl, 2.5 mM CaCl2, 5.4 mM glucose and 1.2 mM MgCl2. The esophagus was opened along the lesser curvature. The location of the squamocolumnar junction was identified and the mucosa was then peeled off. The submucosal connective tissues were then removed by microspring scissors. The mucosa from esophagus was sliced into 0.5-mm-thick sections with a Stadie Riggs tissue slicer (Thomas Scientific Apparatus, Philadelphia, PA, USA). The last slices were cut into 2×2 mm tissue squares using scissors.
The sliced tissue was placed into DMEM supplemented with 10% FBS containing 100 U/ml penicillin, 0.1 mg/ml streptomycin, and 0.25 µg/ml amphotericin B and incubated in a humidified atmosphere of 5% CO2 and 95% air at 37℃ [31]. After 10 days, the medium was exchanged with fresh DMEM containing 10% FBS. After reaching confluence, the cells were detached using 1% trypsin in HBSS with bicarbonate. Afterwards, the cells were then counted, seeded at 2×105 cells/ml on 100-mm culture dishes and maintained in DMEM containing 10% FBS. The medium was changed every 48 hours until the cells reached confluence. Experiments were performed on cells at passage 3 or 4.
The cell viability was determined by the conventional 3-(4,5-dimethylthiazol-2-yl)-2,5-diphenyl-tetrazolium bromide (MTT) reduction assay using the method previously described [32]. Briefly, cells were made quiescent at confluence by incubation in serum-free DMEM for 24 hours to arrest cell growth and silence gene activity, followed by treatment with each indicated agent for the designated time periods. After incubation, the cells were rapidly washed twice with ice-cold PBS and incubated with MTT solution (final concentration, 5 mg/ml) for 4 hours at 37℃. Then, the supernatant was removed and the formazan crystals were dissolved with DMSO. Absorbance at 570 nm was measured with a microplate reader (Molecular Devices, Sunnyvale, CA, USA), and ECC Cell image were observed and acquired with Leica DM IL LED fluorescence microscopy (Leica Microsystems, Wetzlar, Germany).
When the cells reached confluence, they were serum starved by incubation in serum-free DMEM for 24 hours. The cells were then stimulated with each compound for the indicated time periods or at the specified concentrations. After incubation, the cells were rapidly washed twice with ice-cold PBS and lysed with an ice-cold lysis buffer (20 mM Tris-HCl (pH 7.4), 0.5 mM EDTA, 0.5 mM EGTA, 1% (w/v) Triton X-100, 0.01% (w/v) SDS, 10 µg/ml leupeptin, 10 µg/ml aprotinin, 1 mM PMSF, and 0.7 µg/ml β-mercaptoethanol) for 5 min. The lysates were scraped with a cell scraper and collected in Eppendorf tubes. They were then sonicated (6 seconds, 3×) and centrifuged for 10 min at 13,000 rpm at 4℃ to remove cellular debris; the supernatants were collected and stored at -70℃ for protein assay and Western blot analysis.
Equal amounts of protein from each sample were subjected to electrophoresis on a 10% SDS-polyacrylamide gel and transferred to a NC membrane using the Power Pac 1,000 (Bio-Rad, Melville, NY, USA) power supply. To block any nonspecific binding, the NC membrane was incubated in 5% nonfat dry milk in PBS for 60 min followed by three rinses in milk-free PBS. The membranes were incubated overnight at 4℃ with primary antibodies raised against 5-LOX, phospho-SAPK/JNK, or phospho-p38 MAP kinase followed by three washes with PBS containing 0.05% Tween 20. This was followed by 60 min incubation in a horseradish peroxidase-conjugated secondary antibody. Immunoreactive proteins were detected with an ECL agent. Molecular masses were estimated by comparison with a prestained molecular mass marker. To confirm the uniformity of protein loading, the same blots were subsequently stripped with Western blot stripping buffer and reprobed with actin, SAPK/JNK, and p38 MAPK antibodies [33]. The results were analyzed by Quantity One analysis software (Bio-Rad Chemical Division, Richmond, CA, USA). The percentage of p38 MAPK, SAPK/JNK activation or the 5-LOX expression was calculated as the ratio of phosphorylated p38 MAPK to total p38 MAPK, phosphorylated SAPK/JNK to total SAPK/JNK or 5-LOX to Actin, respectively.
Cells were pretreated with each indicated agent for the designated time periods. EECs were then stimulated with H2O2. Regarding experiments designed to measure the production of LTB4, the medium was collected, centrifuged, and stored at -70℃ until assayed [34]. The level of LTB4 released into the culture medium was quantified using a LTB4 EIA kit. Assays were then performed according to the manufacturer's instructions.
To investigate the cytotoxic effects concerning the external addition of H2O2, we performed MTT assays in cultured EECs. Cells were incubated with H2O2 at the indicated concentration for 24 hours, and then cell viability was measured using the MTT assay (Fig. 1A). As a result, cell viability was significantly decreased by greater than 300 µM H2O2 in a concentration-dependent manner. Moreover, cell viability after exposure to 600 µM H2O2 was reduced to 40% of the control. In addition, morphologic observation of EECs treated with H2O2 was performed to identify the H2O2-induced morphologic change (Fig. 1B). After H2O2 treatment, the number of cells was reduced and a high fraction of cells exhibited cytoplasmic condensation.
To study the cytotoxic effect of eupatilin, we employed the MTT assay in EECs (Fig. 1C). We treated EECs with various concentrations of eupatilin for 24 hours. The cell viability did not show significant changes until 200 µM of eupatilin was used.
To study the cytoprotective effect of eupatilin against H2O2-induced cell death, cells were pre-incubated with 25~150 µM eupatilin for 12 hours and then exposed to 600 µM H2O2 for 24 hours (Fig. 1D). H2O2 treatment alone significantly decreased cell viability to about 40%. However, when cells were pretreated with 25~150 µM eupatilin for 12 hours, the cell viability was restored to roughly 65% of the control at a concentration of 150 µM. Morphologic observation of EECs treated with H2O2 in the absence or presence of eupatilin was also performed. (Fig. 1E). H2O2 induced cytoplasmic condensation of EECs, whereas the morphology of cells incubated with H2O2 in the presence of 150 µM eupatilin was shown to maintain similar to control.
To examine whether H2O2 causes 5-LOX expression in cultured EECs, the cells were exposed to H2O2 at the indicated concentrations, and then 5-LOX expression was measured by western blotting analysis. When the cells were treated with 100~400 µM H2O2 for 24 hours, 5-LOX expression peaked at 300 µM H2O2 (Fig. 2A). Next, to assess whether eupatilin affects H2O2-induced 5-LOX expression in EECs, western blotting analysis was performed (Fig. 2B). After pre-treatment with the indicated concentration of eupatilin for 12 hours, EECs were further exposed to 300 µM H2O2 in the presence of eupatilin for 24 hours. Moreover, pretreatment with 100~150 µM eupatilin significantly reduced the H2O2-induced 5-LOX protein expression. 5-Lox expression by H2O2 was reduced 10% by eupatillin.
Serum-starved EECs were treated with or without 150 µM eupatilin for 12 hours, 5 mM NAC, 30 µM SB202190 or 30 µM SP600125 for 1 hours prior to 300 µM H2O2 stimulation for 24 hours. As shown in Fig. 3A, pretreatment of the cells with SB202190, SP600125 or NAC significantly reduced H2O2-induced the 5-LOX expression. These results indicated that p38 MAPK, JNK and ROS scavenging action may mediate the inhibitory effect of eupatilin on the 5-LOX expression by H2O2. In parallel experiments, the inhibitory effect of eupatilin on H2O2-induced LTB4 production was determined using LTB4 EIA kit (Fig. 3B). Fig. 3B showed that treatment of cultured EECs with H2O2 caused a significant increase in the production of LTB4. However, when EECs were treated with eupatilin, SB202190, SP600125, NAC or NDGA (a lipoxygenase inhibitor), the levels of LTB4 production was significantly reduced by all of them. Eupatilin and inhibitors reduced 10~12 pg/ml of LTB4 production. These data were similar to the results of the 5-LOX expression by H2O2 with or without inhibitors.
To determine the effect of H2O2 on activation of MAPKs, the phosphorylation of p38 MAPK and JNK was investigated. The concentration-dependence of p38 MAPK and JNK phosphorylation was investigated by Western blot analysis (Fig. 4). p38 MAPK and JNK phosphorylation levels were significantly increased by 300 µM H2O2. P38 MAPK expression after exposure to 300 µM H2O2, in Fig. 4A, was increased to 40% of the control and JNK activation after exposure to 300 µM H2O2, in Fig. 4B, was increased to 30% of the control.
Serum-starved EECs were treated in the presence or absence of 150 µM eupatilin for 12 hr and with NAC, SB202190 or SP600125 for 1 hr prior to 300 µM H2O2 treatment for 24 hr. p38 MAPK and JNK phosphorylation levels in EECs were estimated by Western blot analysis (Fig. 5). Pretreatment with eupatilin, NAC, SB202190, or SP600125 inhibited the expression of H2O2-induced p38 MAPK and JNK phosphorylation.
The group with H2O2 treatment as well as Eupatilin, in Fig. 5A, decreased 21% when compared to that of the H2O2 treatment alone. The ROS scavengers presented similar effect to Eupatilin, and MAPK inhibitors showed further decrease down to 30%, similar to that of the non-treated group. In Fig. 5B, the effect of drugs and reagents on P-JNK/T-JNK cell were shown. Eupatilin, MAPK inhibitors, and ROS scavenger treated groups showed diminishment as well.
In this study, the addition of external H2O2 to esophageal epithelial cells exhibited significant cytotoxicity. The cell viability was decreased and the shapes of cells were remarkably altered. However, eupatilin enhanced the reduction of cell viability by H2O2. Previously, we identified that the cytoprotective properties of eupatilin could be attributed to the induction of the antioxidant protein heme oxygenase-1 (HO-1) in ileal smooth muscle cells or esophageal epithelial cells [30,35]. We also confirmed that eupatilin induced HO-1 expression in esophageal epithelium of rats in vivo [35]. Cytoprotective roles for HO-1 have been demonstrated in many models, such as in hyperoxia-induced lung injury and reperfusion-induced injury of a transplanted liver [36,37]. It has been known that a variety of phytochemicals in medicinal herbs and dietary plants exert potent antioxidative and anti-inflammatory action via induction of HO-1 [38,39]. Eupatilin is also a flavonoid compound isolated from a traditional Korean herbal medicine, Artemisiae argyi folium. In the present study, although we did not test for the role of eupatilin-induced HO-1 in cell death by H2O2, we expect that the ability of eupatilin regarding HO-1 induction may be involved in cytoprotection against H2O2-induced cytotoxicity. In addition, the cytotoxicity of H2O2 could be associated with its ability to induce the expression of 5-LOX. As one study previously demonstrated, methyl jasmonate which is a plant stress hormone, induced apoptosis in human prostate carcinoma cells via 5-LOX dependent pathway [40]. In our study, co-treatment of eupatilin with H2O2 inhibited the increase of the H2O2-stimulated 5-LOX expression and LTB4 production. Therefore, it is possible that the cytoprotective effect of eupatilin could involve its ability to decrease the 5-LOX expression.
ROS act as second messengers to stimulate intracellular signaling pathways including MAPK [41]. Modulation of the MAPK signaling pathways by H2O2 is distinctive, depending on the cell type, concentration and duration of exposure. For example, exogenous H2O2 activates ERK and JNK but not p38 MAPK in human gastric epithelial cells [42], while endogenous H2O2 production by ethanol treatment in EECs activates ERK, but not JNK and p38 MAPK. As shown in our results, the H2O2-induced 5-LOX expression and LTB4 production were mediated by activation of p38 MAPK and JNK. Eupatilin inhibited H2O2-induced p38 MAPK and JNK activation. Considering the inhibitory effect of SB202190and SP600125on the 5-LOX expression, eupatilin may involve inhibition of the p38 MAPK and JNK pathways. In macrophages LTB4 or LTD4 have pro-proliferative effects through MAPK and phosphatidyl inositol 3-kinase pathways [43]. In addition, ERKs and p38 MAPK-regulated signaling can act stimulation of 5-LOX [44], and stress-induced nuclear export of 5-LOX is through activation of the p38 MAPK pathway [45]. Considering these observations, we suppose that MAPKs might participate in upstream or downstream of 5-LOX pathway as mediators.
The therapeutic approach and chemical design of anti-inflammatory agents has mainly targeted the development of selective cycloxygenase (COX) inhibitors. However, various leukotrienes, in particular LTB4, have emerged as new targets because of their contribution to the inflammatory process at the site of injury. For this reason, development of compounds that will inhibit COX and 5-LOX simultaneously could lead to enhanced anti-inflammatory effects and reduce undesirable side effects [46]. Eupatilin is already known as an effective COX inhibitor. For example, eupatilin remarkably inhibits LPS-induced expression of COX-2 in J774A.1 cells in a concentration-dependent manner [47]. In addition, eupatilin exhibits a down-regulatory effect on the COX-2 expression in carrageenan-induced inflammation within an air pouch on the backs of mice [48].
Considering the 5-LOX-inhibiting effect of eupatilin in the present study, eupatilin might act as a dual inhibitor with regards to COX and 5-LOX. Taken together, the present study provides evidence that eupatilin has a protective effect against H2O2-induced cell damage in cultured feline EEC. Eupatilin also inhibits the H2O2-induced 5-LOX expression and LTB4 production through the inactivation of p38 MAPK and JNK pathways.
Figures and Tables
Fig. 1
Effect of H2O2 on the cell viability of feline EECs and Effect of eupatilin on the H2O2-induced cell viability. Serum-starved EECs were incubated with H2O2 for 24 hours at the indicated concentration. (A) The cell viability was estimated using MTT assay. (B) The morphologic changes of EECs were observed (magnification: 100×). (C) Serumstarved EECs were incubated in the presence of eupatilin alone for 12 hours at the indicated concentration. (D) the cells were incubated in the 600 µM H2O2 with or without eupatilin 12 hours before and during 24 hours, and then their survival was estimated using the MTT assay and the morphologic changes of cells were observed (E) (magnification: 100×). Data are expressed as Means±S.E of four experiments (*; p<0.05 vs. control, **; p<0.01 vs. control, #; p< 0.05 vs. H2O2 alone, ##; p<0.01 vs. H2O2 alone).
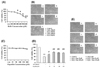
Fig. 2
Effects of eupatilin on the H2O2-induced 5-LOX expression. (A) Serum-starved EECs were treated with H2O2 for 24 hours at each dose. (B) Serum-starved cells were preincubated in the presence of eupatilin for 12 hours at the indicated concentration and then stimulated with 300 µM H2O2 for 24 hours. 5-LOX expression was estimated by Western blot. Data are expressed as Means±S.E of three experiments (*; p<0.05 vs. control, #; p<0.05 vs. H2O2 alone).
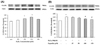
Fig. 3
The effect of eupatilin, SB202190, SP600125 or NAC on the H2O2-induced 5-LOX expression and LTB4 production. Serum-starved EECs were preincubated in the presence of eupatilin (150 µM, 12 hours), SB202190 (30 µM, 1 hours), SP600125 (30 µM, 1 hours), or NAC (5 mM, 1 hours). The cells were then stimulated with H2O2 (300 µM, 24 hours). (A) The change level of 5-LOX expression was estimated by Western blot analysis. (B) The production level of LTB4 was estimated by LTB4EIA kit. Data are expressed as Means±S.E of three experiments (*; p<0.05 vs. control, #; p<0.05, ##; p<0.01 vs. H2O2 alone).
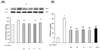
Fig. 4
p38 MAPK and JNK phosphorylation by H2O2. Serum-starved EECs were stimulated with H2O2 for 24 hr at each dose. (A) The change in the level of phosphorylated p38 MAPK was estimated by Western blot analysis. (B) The change of phosphorylated JNK level was estimated by Western blot analysis. Data are expressed as Means±S.E of three experiments (*; p<0.05 vs. control, **; p<0.001 vs. control).
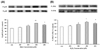
Fig. 5
The effect of eupatilin, SB202190, SP600125, NAC on p38 MAPK (A) and (B) JNK phosphorylation in EECS. Serum-starved EECs were preincubated in the presence of eupatilin (150 µM, 12 hr), SB202190 (p38 MAPK inhibitor, 30 µM, 1 hr), SP600125 (JNK inhibitor, 30 µM, 1hr), or NAC (N-acetyl-L-cysteine, ROS scavenger, 5 mM, 1 hr). EECs were then stimulated with H2O2 (300 µM, 24 hr). The change of phosphorylated p38MAPK and JNK was estimated by Western blot analysis. Data are expressed as Means±S.E of three experiments (#; p<0.05 vs. control, *; p<0.05 vs. H2O2 alone).
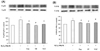
ACKNOWLEDGEMENTS
This research was supported by the Basic Science Research Program through the National Research Foundation of Korea (NRF) funded by the Ministry of Education, Science and Technology (2011-0012139).
References
1. Lee SH, Heo JS, Lee MY, Han HJ. Effect of dihydrotestosterone on hydrogen peroxide-induced apoptosis of mouse embryonic stem cells. J Cell Physiol. 2008. 216:269–275.
2. de Magalhães JP, Church GM. Cells discover fire: employing reactive oxygen species in development and consequences for aging. Exp Gerontol. 2006. 41:1–10.
3. Menon SG, Goswami PC. A redox cycle within the cell cycle: ring in the old with the new. Oncogene. 2007. 26:1101–1109.
4. Lee MN, Lee SH, Lee MY, Kim YH, Park JH, Ryu JM, Yun SP, Lee YJ, Kim MO, Park K, Han HJ. Effect of dihydrotestosterone on mouse embryonic stem cells exposed to H2O2-induced oxidative stress. J Vet Sci. 2008. 9:247–256.
5. Lee SH, Na SI, Heo JS, Kim MH, Kim YH, Lee MY, Kim SH, Lee YJ, Han HJ. Arachidonic acid release by H2O2 mediated proliferation of mouse embryonic stem cells: involvement of Ca2+/PKC and MAPKs-induced EGFR transactivation. J Cell Biochem. 2009. 106:787–797.
6. Fischer S, Wiesnet M, Renz D, Schaper W. H2O2 induces paracellular permeability of porcine brain-derived microvascular endothelial cells by activation of the p44/42 MAP kinase pathway. Eur J Cell Biol. 2005. 84:687–697.
7. Lee WC, Choi CH, Cha SH, Oh HL, Kim YK. Role of ERK in hydrogen peroxide-induced cell death of human glioma cells. Neurochem Res. 2005. 30:263–270.
8. Farinati F, Cardin R, Degan P, Rugge M, Mario FD, Bonvicini P, Naccarato R. Oxidative DNA damage accumulation in gastric carcinogenesis. Gut. 1998. 42:351–356.
9. Martindale JL, Holbrook NJ. Cellular response to oxidative stress: signaling for suicide and survival. J Cell Physiol. 2002. 192:1–15.
10. Kang KA, Lee KH, Zhang R, Piao MJ, Kang MY, Kwak YS, Yoo BS, You HJ, Hyun JW. Protective effects of Castanopsis cuspidate through activation of ERK and NF-kappaB on oxidative cell death induced by hydrogen peroxide. J Toxicol Environ Health A. 2007. 70:1319–1328.
11. Olyaee M, Sontag S, Salman W, Schnell T, Mobarhan S, Eiznhamer D, Keshavarzian A. Mucosal reactive oxygen species production in oesophagitis and Barrett's oesophagus. Gut. 1995. 37:168–173.
12. Stein HJ, Esplugues J, Whittle BJ, Bauerfeind P, Hinder RA, Blum AL. Direct cytotoxic effect of oxygen radicals on the gastric mucosa. Surgery. 1989. 106:318–323.
13. Stein HJ, Hinder RA, Oosthuizen MM. Gastric mucosal injury caused by hemorrhagic shock and reperfusion: protective role of the antioxidant glutathione. Surgery. 1990. 108:467–473.
14. Li CT, Zhang WP, Lu YB, Fang SH, Yuan YM, Qi LL, Zhang LH, Huang XJ, Zhang L, Chen Z, Wei EQ. Oxygen-glucose deprivation activates 5-lipoxygenase mediated by oxidative stress through the p38 mitogen-activated protein kinase pathway in PC12 cells. J Neurosci Res. 2009. 87:991–1001.
15. Funk CD. Prostaglandins and leukotrienes: advances in eicosanoid biology. Science. 2001. 294:1871–1875.
16. Koshihara Y, Neichi T, Murota S, Lao A, Fujimoto Y, Tatsuno T. Selective inhibition of 5-lipoxygenase by natural compounds isolated from Chinese plants, Artemisia rubripes Nakai. FEBS Lett. 1983. 158:41–44.
17. Werz O, Steinhilber D. Therapeutic options for 5-lipoxygenase inhibitors. Pharmacol Ther. 2006. 112:701–718.
18. Charlier C, Michaux C. Dual inhibition of cyclooxygenase-2 (COX-2) and 5-lipoxygenase (5-LOX) as a new strategy to provide safer non-steroidal anti-inflammatory drugs. Eur J Med Chem. 2003. 38:645–659.
19. Brain SD, Williams TJ. Leukotrienes and inflammation. Pharmacol Ther. 1990. 46:57–66.
20. Thalhamer T, McGrath MA, Harnett MM. MAPKs and their relevance to arthritis and inflammation. Rheumatology (Oxford). 2008. 47:409–414.
21. Tamura S, Hanada M, Ohnishi M, Katsura K, Sasaki M, Kobayashi T. Regulation of stress-activated protein kinase signaling pathways by protein phosphatases. Eur J Biochem. 2002. 269:1060–1066.
22. Ichijo H, Nishida E, Irie K, ten Dijke P, Saitoh M, Moriguchi T, Takagi M, Matsumoto K, Miyazono K, Gotoh Y. Induction of apoptosis by ASK1, a mammalian MAPKKK that activates SAPK/JNK and p38 signaling pathways. Science. 1997. 275:90–94.
23. Middleton E Jr. Effect of plant flavonoids on immune and inflammatory cell function. Adv Exp Med Biol. 1998. 439:175–182.
24. Moreira AJ, Fraga C, Alonso M, Collado PS, Zetller C, Marroni C, Marroni N, González-Gallego J. Quercetin prevents oxidative stress and NF-kappaB activation in gastric mucosa of portal hypertensive rats. Biochem Pharmacol. 2004. 68:1939–1946.
25. Middleton E Jr, Kandaswami C, Theoharides TC. The effects of plant flavonoids on mammalian cells: implications for inflammation, heart disease, and cancer. Pharmacol Rev. 2000. 52:673–751.
26. Ryu BK, Ahn BO, Oh TY, Kim SH, Kim WB, Lee EB. Studies on protective effect of DA-9601, Artemisia asiatica extract, on acetaminophen- and CCl4-induced liver damage in rats. Arch Pharm Res. 1998. 21:508–513.
27. Ahn BO, Ko KH, Oh TY, Cho H, Kim WB, Lee KJ, Cho SW, Hahm KB. Efficacy of use of colonoscopy in dextran sulfate sodium induced ulcerative colitis in rats: the evaluation of the effects of antioxidant by colonoscopy. Int J Colorectal Dis. 2001. 16:174–181.
28. Hahm KB, Kim JH, You BM, Kim YS, Cho SW, Yim H, Ahn BO, Kim WB. Induction of apoptosis with an extract of Artemisia asiatica attenuates the severity of cerulein-induced pancreatitis in rats. Pancreas. 1998. 17:153–157.
29. Oh TY, Lee JS, Ahn BO, Cho H, Kim WB, Kim YB, Surh YJ, Cho SW, Lee KM, Hahm KB. Oxidative stress is more important than acid in the pathogenesis of reflux oesophagitis in rats. Gut. 2001. 49:364–371.
30. Song HJ, Shin CY, Oh TY, Sohn UD. The protective effect of eupatilin on indomethacin-induced cell damage in cultured feline ileal smooth muscle cells: involvement of HO-1 and ERK. J Ethnopharmacol. 2008. 118:94–101.
31. Kim do Y, Song HJ, Jeong JH, Suh JS, Sohn UD. Regulation of lysophosphatidic acid-induced COX-2 expression by ERK1/2 activation in cultured feline esophageal epithelial cells. Arch Pharm Res. 2008. 31:1331–1338.
32. Park SY, Sohn UD. Inhibitory effect of rosiglitazone on the acid-induced intracellular generation of hydrogen peroxide in cultured feline esophageal epithelial cells. Naunyn Schmiedebergs Arch Pharmacol. 2011. 383:191–201.
33. Kim JS, Song HJ, Ko SK, Whang WK, Sohn UD. Quercetin-3-O-beta-d-glucuronopyranoside (QGC)-induced HO-1 expression through ERK and PI3K activation in cultured feline esophageal epithelial cells. Fitoterapia. 2010. 81:85–92.
34. Kim N, Cao W, Song IS, Kim CY, Sohn UD, Harnett KM, Biancani P. Leukotriene D4-induced contraction of cat esophageal and lower esophageal sphincter circular smooth muscle. Gastroenterology. 1998. 115:919–928.
35. Song HJ, Shin CY, Oh TY, Min YS, Park ES, Sohn UD. Eupatilin with heme oxygenase-1-inducing ability protects cultured feline esophageal epithelial cells from cell damage caused by indomethacin. Biol Pharm Bull. 2009. 32:589–596.
36. Amersi F, Buelow R, Kato H, Ke B, Coito AJ, Shen XD, Zhao D, Zaky J, Melinek J, Lassman CR, Kolls JK, Alam J, Ritter T, Volk HD, Farmer DG, Ghobrial RM, Busuttil RW, Kupiec-Weglinski JW. Upregulation of heme oxygenase-1 protects genetically fat Zucker rat livers from ischemia/reperfusion injury. J Clin Invest. 1999. 104:1631–1639.
37. Otterbein LE, Kolls JK, Mantell LL, Cook JL, Alam J, Choi AM. Exogenous administration of heme oxygenase-1 by gene transfer provides protection against hyperoxia-induced lung injury. J Clin Invest. 1999. 103:1047–1054.
38. Wung BS, Hsu MC, Wu CC, Hsieh CW. Piceatannol upregulates endothelial heme oxygenase-1 expression via novel protein kinase C and tyrosine kinase pathways. Pharmacol Res. 2006. 53:113–122.
39. Chen CY, Jang JH, Li MH, Surh YJ. Resveratrol upregulates heme oxygenase-1 expression via activation of NF-E2-related factor 2 in PC12 cells. Biochem Biophys Res Commun. 2005. 331:993–1000.
40. Ezekwudo DE, Wang RC, Elegbede JA. Methyl jasmonate induced apoptosis in human prostate carcinoma cells via 5-lipoxygenase dependent pathway. J Exp Ther Oncol. 2007. 6:267–277.
41. Li SY, Li Q, Shen JJ, Dong F, Sigmon VK, Liu Y, Ren J. Attenuation of acetaldehyde-induced cell injury by overexpression of aldehyde dehydrogenase-2 (ALDH2) transgene in human cardiac myocytes: role of MAP kinase signaling. J Mol Cell Cardiol. 2006. 40:283–294.
42. Lee S, Lee M, Kim SH. Eupatilin inhibits H(2)O(2)-induced apoptotic cell death through inhibition of mitogen-activated protein kinases and nuclear factor-kappaB. Food Chem Toxicol. 2008. 46:2865–2870.
43. Nieves D, Moreno JJ. Role of 5-lipoxygenase pathway in the regulation of RAW 264.7 macrophage proliferation. Biochem Pharmacol. 2006. 72:1022–1030.
44. Werz O, Bürkert E, Fischer L, Szellas D, Dishart D, Samuelsson B, Rådmark O, Steinhilber D. Extracellular signal-regulated kinases phosphorylate 5-lipoxygenase and stimulate 5-lipoxygenase product formation in leukocytes. FASEB J. 2002. 16:1441–1443.
45. Hanaka H, Shimizu T, Izumi T. Stress-induced nuclear export of 5-lipoxygenase. Biochem Biophys Res Commun. 2005. 338:111–116.
46. Fiorucci S, Meli R, Bucci M, Cirino G. Dual inhibitors of cyclooxygenase and 5-lipoxygenase. A new avenue in anti-inflammatory therapy? Biochem Pharmacol. 2001. 62:1433–1438.
47. Choi EJ, Lee S, Chae JR, Lee HS, Jun CD, Kim SH. Eupatilin inhibits lipopolysaccharide-induced expression of inflammatory mediators in macrophages. Life Sci. 2011. 88:1121–1126.
48. Min SW, Kim NJ, Baek NI, Kim DH. Inhibitory effect of eupatilin and jaceosidin isolated from Artemisia princeps on carrageenan-induced inflammation in mice. J Ethnopharmacol. 2009. 125:497–500.