Abstract
The receptor activator of NF-κB ligand (RANKL) signal is an activator of tumor necrosis factor receptor-associated factor 6 (TRAF6), which leads to the activation of NF-κB and other signal transduction pathways essential for osteoclastogenesis, such as Ca2+ signaling. However, the intracellular levels of inositol 1,4,5-trisphosphate (IP3) and IP3-mediated cellular function of RANKL during osteoclastogenesis are not known. In the present study, we determined the levels of IP3 and evaluated IP3-mediated osteoclast differentiation and osteoclast activity by RANKL treatment of mouse leukemic macrophage cells (RAW 264.7) and mouse bone marrow-derived monocyte/macrophage precursor cells (BMMs). During osteoclastogenesis, the expression levels of Ca2+ signaling proteins such as IP3 receptors (IP3Rs), plasma membrane Ca2+ ATPase, and sarco/endoplasmic reticulum Ca2+ ATPase type2 did not change by RANKL treatment for up to 6 days in both cell types. At 24 h after RANKL treatment, a higher steady-state level of IP3 was observed in RAW264.7 cells transfected with green fluorescent protein (GFP)-tagged pleckstrin homology (PH) domains of phospholipase C (PLC) δ, a probe specifically detecting intracellular IP3 levels. In BMMs, the inhibition of PLC with U73122 [a specific inhibitor of phospholipase C (PLC)] and of IP3Rs with 2-aminoethoxydiphenyl borate (2APB; a non-specific inhibitor of IP3Rs) inhibited the generation of RANKL-induced multinucleated cells and decreased the bone-resorption rate in dentin slice, respectively. These results suggest that intracellular IP3 levels and the IP3-mediated signaling pathway play an important role in RANKL-induced osteoclastogenesis.
Bone is a connective tissue that constructs the skeleton and is composed of the intracellular matrix proteoglycans, osteoblasts, osteoclasts, and osteocytes. It is a dynamic tissue that is constantly renewed by bone remodeling. Bone remodeling is an active process and is regulated by the activity of bone-forming osteoblasts and bone-resorbing osteoclasts [1,2]. In fact, an imbalance in bone remodeling can cause pathological conditions such as rheumatoid arthritis, osteoporosis, and osteopetrosis.
Cells grow and differentiate through cell-to-cell interactions, and extracellular signals lead to specific cell reactions through signal transduction. Cell-to-cell signaling of mesenchymal cells, including osteoblasts, induces the differentiation of osteoclast precursor cells into mature osteoclasts [3,4]. Receptor activator of nuclear factor kappa B (NF-κB) ligand (RANKL) is expressed on the surfaces of osteoclasts, and an essential factor for osteoclast differentiation. RANKL, a member of the tumor necrosis factor (TNF) family, is expressed in the bone, bone marrow, and lymphoid tissue during osteoclastogenesis. RANKL binds to RANK and transmits a differentiation signal [5]. It is known that RANKL stimulation initially activates tumor necrosis factor receptor-associated factor 6 (TRAF6), and then sequentially activates NF-κB, c-fos, JNK, ERK, and PI3K in osteoclast precursor cells [6,7]. Recent studies of osteoclastogenesis have focused on TRAF6, JNK, and NF-κB signaling pathways triggered by the binding of RANKL to RANK on osteoclast precursors. During osteoclastogenesis, auto-amplification of NFATc1 induces osteoclast-specific genes including AP-1, tartrate-resistant acid phosphatase (TRAP), calcitonin receptor, and cathepsin K [6]. Recently, Ca2+ signaling has been recognized as an essential pathway in the differentiation of osteoclasts. In particular, a previous study has shown that treatment with a Ca2+ chelator inhibits osteoclastogenesis in osteoclast precursor cells [8], suggesting that Ca2+ signaling is important in osteoclastogenesis.
Hormone and neurotransmitter-induced increases in intracellular Ca2+ concentration ([Ca2+]i) regulate gene expression, growth, differentiation, muscle contraction, memory, and learning [9]. Particularly, in osteoclast differentiation, Ca2+ was reported to play an important role by sequentially activating calcineurin and NFATc1 [5,8,10]. In this study, Ca2+ signals were shown to exist in a unique form, such as Ca2+ oscillations, which are a recurring phenomena of increases and decreases in [Ca2+]i. Generally, in non-excitable cells, the increase in [Ca2+]i occurs through the activation of Ca2+ channels or Ca2+ release from the ER into the cytoplasm, and is then followed by activation of the sarco/endoplasmic reticulum Ca2+ ATPase (SERCA) and the plasma membrane Ca2+ ATPase (PMCA) that remove Ca2+ from the cytosol [11,12]. In most cells, extracellular signals such as hormones and neurotransmitters stimulate phospholipase C (PLC) and then hydrolyze a plasma membrane lipid phosphatidyl-inositol bisphosphate (PIP2) to generate inositol 1,4,5-trisphosphate (IP3) in the cytosol. Increased levels of IP3 promote Ca2+ release into the cytosol by changing Ca2+ permeability of the IP3Rs in the ER, which is the intracellular Ca2+ store. Ca2+ release from the ER can change the conductance of Ca2+ release-activated Ca2+ channels. It induces Ca2+ influx from an environment of high concentration of extracellular Ca2+ [12]. An induced increase in [Ca2+]i is removed by the Ca2+ pump. Nevertheless, there are no reports on the intracellular levels of IP3 in osteoclast differentiation. In the present study, we assessed the levels of IP3 and the associated IP3-induced osteoclast differentiation and activity during RANKL-mediated osteoclastogenesis in osteoclast precursor cells.
The mouse monocyte cell line RAW264.7 (Korean Cell Line Bank, South Korea) and primary cultured, bone marrow-derived monocyte/macrophage precursor cells (BMMs) were respectively cultured in Dulbecco's modified eagle medium (DMEM, Invitrogen, Carlsbad, CA, USA) and α-minimum essential medium (α-MEM, Invitrogen), supplemented with 10% fetal bovine serum (FBS) and 1% antibiotics, in a 37℃ incubator (5% CO2). To maintain BMMs, α-MEM was supplemented with 50 ng/ml macrophage colony-stimulating factor (M-CSF). RANKL and M-CSF were purchased from Koma Biotech, Inc. (Seoul, Korea). U73122, U73343 and 2-aminoethoxydiphenyl borate (2-APB) were from Sigma-Aldrich (St. Louis, MO, USA). Polyclonal antibodies for IP3R1, IP3R2, and IP3R3 were generous gifts from Dr. Akihiko Tanimura (Hokkaido University, Japan), and the monoclonal antibody for PMCA (5F10) was purchased from Santa Cruz Biotechnology (Santa Cruz, CA, USA). The vector containing the PLC δ-PH-GFP construct was a generous gift from Dr. Shmuel Muallem (NIH/NIDCR, USA).
Four-week-old male mice (Koatec, Pyeongtaek, Korea) were sacrificed, and the femur and tibia were separated. Bone marrow cells derived from the femur and tibia were collected and cultured in α-MEM containing 10% FBS and 10 ng/ml M-CSF. The next day, non-adherent cells in the media were collected and seeded on adequate number of plates with 50 ng/ml M-CSF. After 2 days, non-adherent cells were washed out with fresh media, and the adherent cells were used as BMMs.
Whole cell proteins were prepared in lysis buffer [20 mM Tris (pH 7.4), 250 mM NaCl, 2 mM EDTA (pH 8.0), 0.1% Triton X-100, 0.01 mg/ml aprotinin, 0.005 mg/ml leupeptin, 0.4 mM PMSF, and 4 mM NaVO4), and the extracts were centrifuged at 1,100×g for 10 min to remove insoluble material. Cleared extracts (50~100µg of protein/well) were subjected to 6~12 % SDS-PAGE, and the proteins were electrotransferred to a nitrocellulose membrane, blocked with 6% skimmed milk, and probed with antibodies against IP3R1 (1:1,000), IP3R2 (1:5,000), IP3R3 (1:1,000), PMCA (1:5,000), SERCA2b (1:5,000). The blots were washed, exposed to horseradish peroxidase-conjugated secondary antibodies for 1 h, and detected by chemiluminescence (ECL, Amersham Pharmacia Biotech, Schenectady, NY, USA).
RAW264.7 cells were transiently transfected with a green fluorescent protein (GFP)-tagged high affinity (R441Q) or low affinity (K508A) IP3-sequestering sponge [13], or were transfected with PLC-δ1-PH-GFP construct provided by Dr. Shmuel Muallem (NIH/NIDCR, USA) using Lipofectamine 2000 reagent (invitrogen), according to the manufacturer's instructions. Cells were incubated for 48 h at 37℃, in a 5% CO2 atmosphere with saturated humidity to allow expression of each protein was confirmed by GFP fluorescence.
After RANKL treatment, GFP-positive cells were identified, and the GFP density in PLC δ-PH-GFP cDNA-transfected RAW264.7 cells was measured using a confocal laser scanning microscope (Leica, Buffalo, NY, USA) at an excitation wavelength of 488 nm and an emission wavelength of 525 nm.
BMMs were seeded in 48-well plates at a concentration of 1×104 cells per well and pretreated with indicated compounds. Cells were then stimulated with 50 ng/ml sRANKL. After 6 days, a TRAP stain assay was performed to confirm the cell differentiation rate. TRAP-positive cells were stained using a Leukocyte Acid Phosphate Assay Kit (Sigma-Aldrich) by following the manufacturer's procedure. TRAP-positive multinucleated cells (containing ≥3 nuclei) were then counted.
BMMs were collected from 4-week-old mice, as described previously [14]. Collected BMMs were seeded on 48-well bone-slice covered plates, coated with an osteoclast activity assay substrate (OAAS™; OCT, Cheonan, Korea). BMMs in bone-sliced plates were maintained with 50 ng/ml M-CSF and sRANKL for 15 days. After 15 days, cells were washed out with sodium hypochlorite solution for 1 h at room temperature. The bone slices were imaged, and the pits in the bone slices were identified based on pixel area calculations. The data were expressed relative to the pit areas in RANKL-stimulated BMMs by using the MetaMorph software (Molecular Devices).
To assess the effect of RANKL stimulation on the expression levels of Ca2+ signaling proteins such as IP3R, SERCA, and PMCA, RAW264.7 cells and BMMs were treated with RANKL for 24 h, 48 h, 72 h, and 6 days. There were no differences in the expression levels of these Ca2+ signaling proteins after RANKL treatment (Fig. 1, n=3).
Previous studies show that Ca2+ oscillations were generated by RANKL stimulation [15]. To confirm the generation of RANKL-induced Ca2+ oscillations, we treated RANKL for 48 h on BMMs. As a results, RANKL-induced Ca2+ oscillations were observed at 48 h (Fig. 2A, n=3). To determine the role of PLC/IP3-dependent pathway in RANKL-induced Ca2+ oscillations, cells were pretreated with 10 µM U73122, a specific blocker of PLC, or its inactive analog, U73343. U73122 prevented RANKL-induced Ca2+ oscillations, whereas 10 µM U73343 had no effect (Fig. 2B, n=3) and inhibition of IP3R with the IP3R inhibitor 75 µM 2APB inhibited RANKL-induced Ca2+ oscillations in BMMs (Fig. 2C, n=3). In addition, we also observed that expression of the IP3 sponge completely abrogated RANKL-induced Ca2+ oscillations although the expression of low affinity IP3 sponge did not prevent the RANKL-induced Ca2+ oscillations (Fig. 2D, n=3).
Because there were no differences in expression levels of Ca2+ signaling proteins after RANKL treatment (Fig. 1), we hypothesized that RANKL-mediated activation of PLC induced an increase in intracellular IP3 levels, and then sequentially generated Ca2+ oscillations. To test our hypothesis, we transfected RAW264.7 cells with the PLC δ-PH-GFP domain. The localization of PLC δ-PH-GFP domain was confirmed using confocal laser microscopy after 24 h of RANKL treatment. In the steady state, the PLC δ PH-domain was located in the cell membrane in the absence of RANKL treatment; however, after 24 h of RANKL treatment, the PH-domain bound to IP3 and then moved into the cytoplasm (Fig. 3, n=3).
Based on our finding that RANKL induced an increase in intracellular IP3 levels, we further investigated the role of PLC and IP3R activation on RANKL-mediated osteoclast differentiation and osteoclast activity using a TRAP staining assay and pit assay. The blockage 10 µM U73122, and 75 µM 2APB inhibited the generation of RANKL-induced multinucleated cells to 60% and 84.3% of the controls, respectively (Fig. 4A, n=3). In addition, we measured osteoclast activity in RAW264.7 cells and BMMs using dentin slices following U73122 and 2APB treatment. Pit areas in RANKL-induced osteoclastogenesis were decreased to 48.4% and 93.6% of the controls, respectively (Fig. 4B, n=3).
Osteoclastogenesis requires costimulatory receptor signaling mediated through adaptor proteins that contain immunoreceptor tyrosine-based activation motifs (ITAMs), such as the Fc receptor γ (FcR γ) and the 12-kDa DNAX-activating protein [5]. The active signal transmitted through adaptor proteins then induces Ca2+ signaling, which sequentially activates NFATc1 and induces osteoclast differentiation [5,10]. In addition, the signal via TRAF6 activates transcription factors such as c-fos and NF-κB [4] and the Ca2+ signal and activation of NFATc1 are transduction signals occurring after the middle stage of osteoclast differentiation in most cells. It is likely that activation of IP3Rs in the ER may induce Ca2+ oscillations. Because there was no way to directly measure the intracellular concentration of IP3, we transfected cells with a PLC δ-PH domain [16]. The transfected PLC δ-PH domain binds to PIP2 with high specificity and affinity during the resting state. However, it is translocated to the cytoplasm and binds to IP3 when IP3 accumulates via an external signal because the PLC δ-PH domain has a stronger affinity for IP3 than for PIP2 [16]. We demonstrated that RANKL induces an increase in IP3 levels at 24 h after RANKL treatment, after which IP3 binds to the PH-domain and translocates from the membrane to the cytosol. On the other hand, during osteoclastogenesis, PLCγ activation is important to generate Ca2+ signaling, and this has been confirmed by using the PLC inhibitor U73122 [15]. In our study, treatment of cells with U73122 inhibited the generation of RANKL-induced Ca2+ oscillations, multinucleated cells, and reduced the bone-resorption rate. In addition, we observed that treatment with 2APB, an IP3R inhibitor, inhibited the generation of RANKL-induced Ca2+ oscillations, multinucleated cells, and reduced bone-resorption rate. This result is consistent with a previous finding that, Ca2+ oscillations were not generated and in vitro osteoclastogenesis was impaired in IP3R2/3-deficient mice [17]. Therefore, IP3-mediated Ca2+ signaling is essential for osteoclastogenesis.
Previous studies showed that Ca2+ influx channels located on the plasma membrane play an important role in osteoclastogenesis [18,19]. Transient receptor potential cation channel subfamily V member 4 (TRPV4)-deficient mice led to an increase in bone mass; Ca2+ oscillations were normal in these cells at the early stage of osteoclastogenesis; however, they gradually disappeared at the later stages. These results suggest that Ca2+ influx via TRPV4 is necessary for Ca2+ signaling [19]. TRPV5-deficient mice also showed an increase in bone mass, although increased number and size of osteoclasts in vivo, suggesting the importance of Ca2+ influx for resorption activity in mature osteoclasts [18]. Therefore, it remains to be determined how Ca2+ oscillations and Ca2+ channel-mediated Ca2+ influx relate to osteoclastogenesis.
In summary, these results suggest that RANKL induces increases in intracellular IP3 and IP3 plays an important role in osteoclstogenesis. Therefore, manipulation of this signaling pathway may provide a target for treatment of bone-related disease.
ACKNOWLEDGEMENTS
This research was supported by Basic Science Research Program through the National Research Foundation of Korea (NRF) funded by the Ministry of Education, Science and Technology (2012-0000802, 2011-0025684).
References
1. Manolagas SC. Birth and death of bone cells: basic regulatory mechanisms and implications for the pathogenesis and treatment of osteoporosis. Endocr Rev. 2000; 21:115–137. PMID: 10782361.


3. Khosla S. Minireview: the OPG/RANKL/RANK system. Endocrinology. 2001; 142:5050–5055. PMID: 11713196.


4. Kobayashi N, Kadono Y, Naito A, Matsumoto K, Yamamoto T, Tanaka S, Inoue J. Segregation of TRAF6-mediated signaling pathways clarifies its role in osteoclastogenesis. EMBO J. 2001; 20:1271–1280. PMID: 11250893.


5. Takayanagi H. Mechanistic insight into osteoclast differentiation in osteoimmunology. J Mol Med (Berl). 2005; 83:170–179. PMID: 15776286.


6. Boyle WJ, Simonet WS, Lacey DL. Osteoclast differentiation and activation. Nature. 2003; 423:337–342. PMID: 12748652.


7. Ha H, Kwak HB, Lee SK, Na DS, Rudd CE, Lee ZH, Kim HH. Membrane rafts play a crucial role in receptor activator of nuclear factor kappaB signaling and osteoclast function. J Biol Chem. 2003; 278:18573–18580. PMID: 12637570.
8. Takayanagi H, Kim S, Koga T, Nishina H, Isshiki M, Yoshida H, Saiura A, Isobe M, Yokochi T, Inoue J, Wagner EF, Mak TW, Kodama T, Taniguchi T. Induction and activation of the transcription factor NFATc1 (NFAT2) integrate RANKL signaling in terminal differentiation of osteoclasts. Dev Cell. 2002; 3:889–901. PMID: 12479813.


9. Berridge MJ, Bootman MD, Roderick HL. Calcium signalling: dynamics, homeostasis and remodelling. Nat Rev Mol Cell Biol. 2003; 4:517–529. PMID: 12838335.


10. Koga T, Inui M, Inoue K, Kim S, Suematsu A, Kobayashi E, Iwata T, Ohnishi H, Matozaki T, Kodama T, Taniguchi T, Takayanagi H, Takai T. Costimulatory signals mediated by the ITAM motif cooperate with RANKL for bone homeostasis. Nature. 2004; 428:758–763. PMID: 15085135.


11. Shin DM, Dehoff M, Luo X, Kang SH, Tu J, Nayak SK, Ross EM, Worley PF, Muallem S. Homer 2 tunes G protein-coupled receptors stimulus intensity by regulating RGS proteins and PLCbeta GAP activities. J Cell Biol. 2003; 162:293–303. PMID: 12860966.
12. Kiselyov K, Shin DM, Muallem S. Signalling specificity in GPCR-dependent Ca2+ signalling. Cell Signal. 2003; 15:243–253. PMID: 12531423.
13. Uchiyama T, Yoshikawa F, Hishida A, Furuichi T, Mikoshiba K. A novel recombinant hyperaffinity inositol 1,4,5-trisphosphate (IP(3)) absorbent traps IP(3), resulting in specific inhibition of IP(3)-mediated calcium signaling. J Biol Chem. 2002; 277:8106–8113. PMID: 11741904.


14. Tian YS, Jeong HJ, Lee SD, Kong SH, Ohk SH, Yoo YJ, Seo JT, Shin DM, Sohn BW, Lee SI. Hyperosmotic Stimulus Down-regulates 1alpha, 25-dihydroxyvitamin D(3)-induced Osteoclastogenesis by Suppressing the RANKL Expression in a Co-culture System. Korean J Physiol Pharmacol. 2010; 14:169–176. PMID: 20631890.
15. Kim MS, Yang YM, Son A, Tian YS, Lee SI, Kang SW, Muallem S, Shin DM. RANKL-mediated reactive oxygen species pathway that induces long lasting Ca2+ oscillations essential for osteoclastogenesis. J Biol Chem. 2010; 285:6913–6921. PMID: 20048168.
16. Hirose K, Kadowaki S, Tanabe M, Takeshima H, Iino M. Spatiotemporal dynamics of inositol 1,4,5-trisphosphate that underlies complex Ca2+ mobilization patterns. Science. 1999; 284:1527–1530. PMID: 10348740.
17. Kuroda Y, Hisatsune C, Nakamura T, Matsuo K, Mikoshiba K. Osteoblasts induce Ca2+ oscillation-independent NFATc1 activation during osteoclastogenesis. Proc Natl Acad Sci USA. 2008; 105:8643–8648. PMID: 18552177.
18. van der Eerden BC, Hoenderop JG, de Vries TJ, Schoenmaker T, Buurman CJ, Uitterlinden AG, Pols HA, Bindels RJ, van Leeuwen JP. The epithelial Ca2+ channel TRPV5 is essential for proper osteoclastic bone resorption. Proc Natl Acad Sci USA. 2005; 102:17507–17512. PMID: 16291808.
19. Masuyama R, Vriens J, Voets T, Karashima Y, Owsianik G, Vennekens R, Lieben L, Torrekens S, Moermans K, Vanden Bosch A, Bouillon R, Nilius B, Carmeliet G. TRPV4-mediated calcium influx regulates terminal differentiation of osteoclasts. Cell Metab. 2008; 8:257–265. PMID: 18762026.


Fig. 1
Effect of receptor activator of NF-κB ligand (RANKL) on the expression of Ca2+ signaling proteins. RAW264.7 cells and mouse bone marrow derived monocyte/macrophage precursor cells (BMMs) were treated with RANKL (50 ng/ml). Levels of three types of inositol 1,4,5-trisphosphate receptors (IP3Rs), plasma membrane Ca2+ ATPase (PMCA), and sarco/endoplasmic reticulum Ca2+ ATPase (SERCA) 2b were measured at different time points from 24 h to 6 days after RANKL treatment.
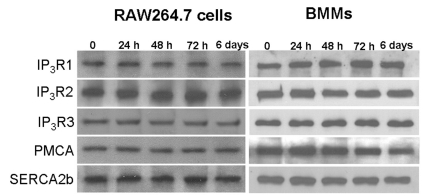
Fig. 2
Effect of PLC and IP3 inhibition on RANKL-induced [Ca2+]i oscillations in BMMs. (A) Generation of Ca2+ oscillations after 48 h with RANKL treatment. (B, C) Inhibitory effect of RANKL-induced [Ca2+]i oscillations by the PLC inhibitor, U73122 and IP3R inhibitor, 2APB. (D) Inhibition of RANKL-induced [Ca2+]i oscillations by elimination of IP3.
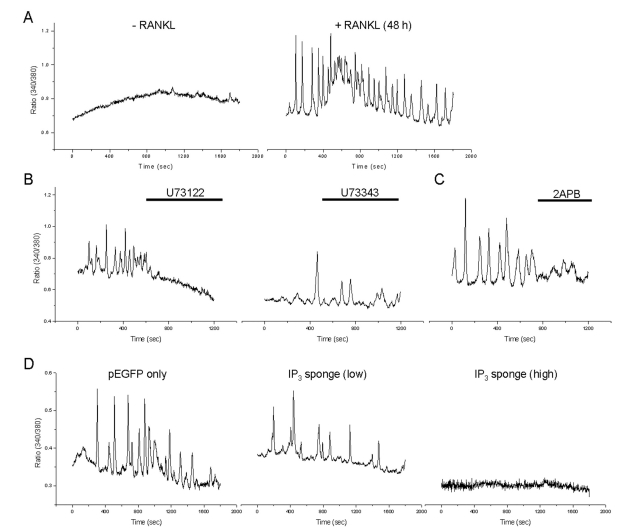
Fig. 3
Localization of phospholipase C (PLC) δ-PH-GFP in RAW264.7 cells after RANKL stimulation. RAW264.7 cells were treated with RANKL following their transfection with the PLC δ-PH-GFP domain. After 24 h with RANKL treatment, GFP intensity was measured using confocal laser microscopy. The graph shows the fluorescence intensity along the lines indicated in the images.
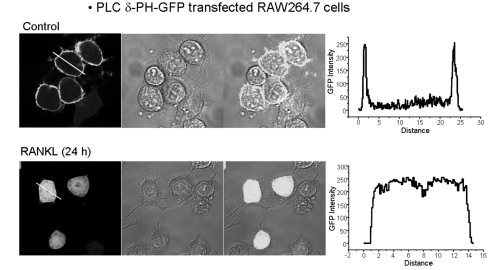
Fig. 4
Effects of IP3R inhibitor and PLC inhibitor on RANKL-induced multinucleated cell formation and pit formation in BMMs. (A) BMMs were seeded in 48-well plates and cultured for 6 days with RANKL and M-CSF. RANKL was simultaneously added to 2-aminoethoxydiphenyl borate (2APB)-, U73122-, and U73343-treated cells. Multinucleated cells (MNCs; ≥3 nuclei) in a well are presented as MNCs per well. Data from three independent experiments are represented as mean±SD (**p<0.005 compared with RANKL-induced MNC counts). (B) BMMs were seeded on OAAS plates, the bottom of which was covered with dentin slices, and cultured for 15 days with RANKL and M-CSF. RANKL was simultaneously added to 2APB- and U73122-treated cells. Data show the relative pit area compared to RANKL-treated BMMs and mean¡¾SD obtained from three independent experiments (**p<0.005 compared with RANKL-induced pit area).
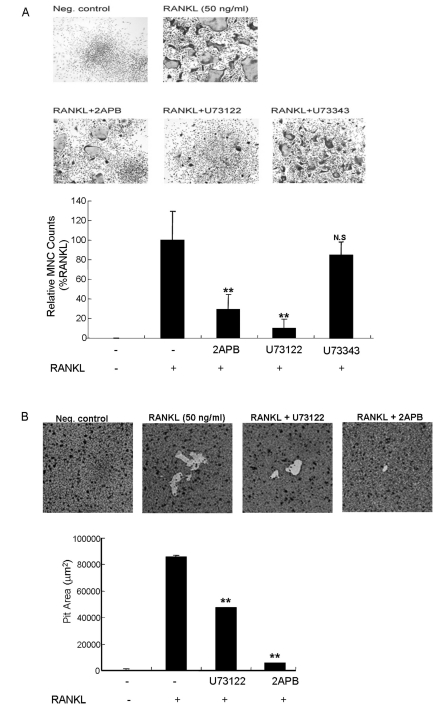