Abstract
Black ginseng (BG) has been widely used as herbal treatment for improving physiological function. In order to investigate the neuroprotective action of this herbal medicine, we examined the influence of BG on the learning and memory of rats using the Morris water maze, and we studied the effects of BG on the central cholinergic system and neural nitric oxide synthesis in the hippocampus of rats with neuronal and cognitive impairment. After middle cerebral artery occlusion was applied for 2h, the rats were administered BG (100 or 400 mgkg-1, p.o.) daily for 2 weeks, followed by training and performance of the Morris water maze test. The rats with ischemic insults showed impaired learning and memory on the tasks. Treatment with BG produced improvement in the escape latency to find the platform. Further, the BG groups showed a reduced loss of cholinergic immunoreactivity and nicotinamide adenine dinucleotide phosphate-diaphorase (NADPH-d)-positive neurons in the hippocampus compared to that of the ISC group. These results demonstrated that BG has a protective effect against ischemia-induced neuronal and cognitive impairment. Our results suggest that BG might be useful for the treatment of vascular dementia.
Acute ischemic stroke is one of the leading causes of adult disability and death in the world, and it affects up to 0.2% of the population every year [1]. Neuronal cell injury, including apoptosis, is the major event in the acute and sub-acute phases of cerebral ischemia [2,3]. Although the exact mechanism of the neurological destruction of the brain caused by cerebral ischemia has not yet been determined, several studies have suggested that oxidative stress, inflammatory cytokines and excitotoxicity may play a role in etiology of this disease [4,5].
Ginseng (Panax ginseng C.A. meyer, Araliaceae) is a traditional medicinal herb, and it is considered to be an adaptogen that improves physiological function [6,7]. In Asia there are a variety of commercial ginseng products, including white, red and black ginseng. White ginseng is produced as a result of dehydration by the sun, while red ginseng is produced by steaming at 95~100℃ for 2~3 h. Black ginseng is produced from red ginseng by 9 cycles of steam treatment, at which point it becomes black in color [8,9]. The pharmacological and biological activities of steam-processed ginseng are greater than that of non-steamed ginseng. During the steaming process, the ratio of major components, including ginsenosides, saponin, phenolics and proteins, is altered as newly produced active components [10,11]. It has been suggested that the steamed ginseng has a greater spectrum of pharmacological effects than that of white ginseng [12-14], including anti-inflammatory, anti-carcinogenesis, anti-stress and antioxidant effects in humans and animals [8,14-16]. Song et al. and Sun et al., reported that the data of HPLC analysis of BG [9,17]. However, few papers on BG products efficacy have been published.
The present study was undertaken to evaluate the neuroprotective effect of BG on the ischemia-induced learning and memory deficits in rats and to elucidate the cholinergic and neural nitric oxide synthesis mechanism underlying these protective effects in rats.
Male Sprague-Dawley rats weighting 250~280 g each were purchased from Samtaco Animal Corp. (Kyungki-do, Korea). The animals were allowed to acclimatize themselves for at least 7 days prior to the experimentation. The animals were housed in individual cages under light-controlled conditions (12/12-hr light/dark cycle) and at a 23℃ room temperature. Food and water were available ad libitum. All the experiments were approved by the Kyung Hee University Institutional animal care and use committee. The rats were allowed at least 1week to adapt to their environment before the experiments.
All the 6-year Korean white and red ginseng roots (P. ginseng C.A. Meyer) were purchased from the largest Korean ginseng market of Geumsan country (Chungcheong Province, South Korea). BG was manufactured by repeatedly steaming WG at 98℃ for 3 h in a pottery apparatus and drying it at 60℃ for 15 h. The dried BG samples (40 g) were immersed in a 200 ml of dH2O and added 2 L of 80% ethanol and then mixed 500 ml of dH2O. For the extraction, diethyl ether was used three times.
Focal cerebral ischemia was induced using the intraluminal filament technique. Anesthesia was administered with 3% isoflurane in 30% O2/70% N2O, and it was maintained throughout the operation with 0.5~0.6% isoflurane delivered via a nose mask. The right common carotid artery was exposed through a midline cervical incision. A heparinized intraluminal filament (φ0.28 mm, rounded tip) was introduced via the external carotid artery. The rectal temperature was monitored and maintained at 37℃ using a heating pad (Harvard Homeothermic Blanket Control Unit, 50-7061). After 120 min of occlusion, the filament was gently pulled out and the external carotid artery was permanently closed by cauterization. In the sham-operated rats, the right common carotid artery was exposed and the external carotid artery was opened without introducing the filament into the internal carotid artery. After the operation, the animals were allowed to wake up in the incubator (30℃) and they were then moved to their home cages. The rats were divided into four groups. The experimental group was treated with BG100 [100 mgkg-1, p.o., BG100+ISCH group (n=6)] and BG400 [400 mgkg-1, p.o., BG400+ISCH group (n=6)] for 2 weeks after the induction of ischemia. The control group was treated with saline [0.1 mg kg-1, p.o., SAL+ISCH group (n=6)] for 2 weeks after the induction of ischemia. The black ginseng and saline were administered orally everyday in the morning. The naïve normal group (Normal, n=6) was not treated with any drug and operation. The water maze tests were performed the last (second) week.
The swimming pool of the Morris water maze was a circular water tank 200 cm in diameter and 35 cm deep. It was filled to a depth of 21 cm with water at 23±2℃. A platform 15 cm in diameter and 20 cm in height was placed inside the tank with its top surface being 1.5 cm below the surface of the water. The pool was surrounded by many cues that were external to the maze [18]. A CCD camera was equipped with a personal computer for the behavioral analysis. Each rat was received four daily trials. For 6 consecutive days, the rats were tested with three acquisition tests. For the acquisition test, the rat was allowed to search for the hidden platform for 180 s and the latency to escape onto the platform was recorded. The animals were trained to find the platform that was in a fixed position during 6 days for the acquisition test. The inter-trial interval time was 1 min. The performance of the test animals in each water maze trial was assessed by a personal computer for the behavioral analysis (S-mart program, Spain).
Briefly, the rats were anesthetized (sodium pentobarbital, 100 mg/kg, ip), they were perfused transcardially with heparinized phosphate-buffered saline (PBS; pH 7.4) for 30 s and this was followed by 4% paraformaldehyde in 0.1 M phosphate buffer (pH 7.4) for 10~15 min. The brains were postfixed in the same fixative overnight, cryoprotected in 30% sucrose solution in PBS, embedded and serially sectioned on a cryostat (Leica, Germany) at 30µm thickness in the coronal plane and then they were collected in PBS. One set of sections that represented different region of the cortex was dehydrated, rehydrated and stained with cresyl violet (ICN Biomedicals, Aurora, USA) to assess the cell loss and the morphology of the hippocampal area.
The sections were stained for NADPH-d activity as previously described [19,20]. In brief, free-floating sections were incubated at 37℃ for 30 min in 0.05 M PBS containing 0.3% Triton X-100, 0.1 mg/ml nitroblue tetrazolium (Sigma, MO, USA) and 0.1 mg/ml β-NADPH (Sigma). The sections were washed three times with 0.05 M PBS and then they were mounted on gelatin-coated slides. The slides were air-dried overnight at room temperature, rinsed twice with distilled water and dried again. Coverslips were mounted using permount solution. The brain sections were analyzed using the atlas by Paxinos and Watson. The staining intensities of the sections that stained specifically for NADPH-d were quantitatively assessed according to a microdensitometrical method based on optical density and using an image analyzer (Multiscan, Fullerton, CA, USA) [21].
The primary antibodies against the following specific antigen were used: cholineacetyl transferase (sheep polyclonal ChAT, concentration 1:2,000; Cambridge Research Biochemicals, Wilmington, D.E.). The primary antibody was prepared at a dilution in 0.3% PBST, 2% blocking serum and 0.001% kehole limpit hemocyanin (Sigma, USA). The sections were incubated in the primary antiserum for 72 h at 4℃. After three more rinses in PBST, the sections were placed in Vectastain Elite ABC reagent (Vector laboratories, Burlingame, CA) for 2 h at room temperature. Following a further rinsing in PBS, the tissue was developed using diaminobenzadine (Sigma, USA) as the chromogen. Images were captured using a DP2-BSW imaging system (Olympus, CA, USA) and they were processed using Adobe Photoshop. For measuring cells that were immunoreactive for ChAT, a grid was placed on the CA1 and CA3 areas of the hippocampus according to the method of Paxinos et al. [21]. The number of cells was counted at 100× magnification using a microscope rectangle grid that measured 200×200 mm2. The cells were counted in three sections per rat within the hippocampal CA1 and CA3 areas.
Statistical comparisons were done for the behavioral and histochemical studies using one-way ANOVA and repeated measures of ANOVA, respectively, and Tukey post hoc was done. All of the results are presented as means±S.E.M., and we used SPSS 15.0 for Windows for analysis of the statistics. The level of significance was set at p<0.05.
Effect of BG on the performance in the water maze test The effect of BG (100 or 400 mg/kg) on spatial learning was evaluated in the Morris water maze test. As shown in Fig. 1, the escape latency of the SAL+ISCH group was longer due to memory impairment than that of the normal group during all the trial sessions [p<0.05]. Data shows the mean group latencies to reach to hidden platform in the MWM for all the groups for 4 days. The escape latency differed among the groups when the results were averaged over all the sessions. The SAL+ISCH group showed a worse performance than did the normal group (at the 2nd day (p=0.004), 3rd day (p=0.022) and 4th day (p=0.05)). However, BG treatment had no significant interaction effect on the distance traveled to reach the platform from the 6th day.
The results of the evaluations of the cresyl violet positive neurons per section, from different hippocampal formations, are shown in Fig. 2. Post-hoc comparisons indicated that the cresyl violet activity in the hippocampus of the SAL+ISCH group was significantly lower than that of the normal group (p<0.05). In particular, there were significant differences in both the CA1 (F3,37=17.2, p=0.001) and CA3 (F3,37=10.6, p=0.001) areas. However, the number of cresyl violet positive neurons in the BG100+ISCH group was higher than that of the SAL+ISCH group, and particularly in the CA1 (p=0.001) and CA3 (p=0.001) areas. However, the number of cresyl violet positive neurons in the hippocampus of the BG100+ISCH group was not significantly different compared to that of the SAL+ISCH group, but there was a slight trend for a significant interaction effect on the expression of the cresyl violet positive neurons in the hippocampus. We also found that the tissue damage of the cortex was significantly decreased in the BG treated groups compared to that of the SAL+ISCH group.
The results of the evaluations of the NADPH-d-positive neurons per section, from different hippocampal formations, are shown in Fig. 3. Post-hoc comparisons indicated that the number of NADPH-d-positive neurons in the hippocampus of the SAL+ISCH group were noticeably less than that of the normal group (p<0.001). In particular, there were significant differences in both the CA3 area (F3,19=13.6, p=0.001) and the dentate gyrus (F3,19=7.1, p=0.003). However, the number of NADPH-d-positive neurons of the BG100+ISCH group was higher than that of the SAL+ISCH group, both in the CA3 area (p=0.001) and the DG (p=0.001).
The results of the evaluations of the ChAT immunoreactive cells per section, from different hippocampal formations, are shown in Fig. 4. Post-hoc comparisons indicated that the ChAT activity in the hippocampus of the SAL+ISCH group was significantly lower than that of the normal group (p<0.05). In particular, there were significant differences in both the CA1 (F3,16=4.9, p=0.02) and CA3 (F3,16=9.3, p=0.001) areas. However, the ChAT reactivity in the BG treated groups was higher than that of the SAL+ISCH group, and particularly in the CA3 area (BG 100, p=0.05; BG 400, p=0.014). However, the ChAT reactivity in the hippocampal CA1 area showed no significant differences among the groups.
In this study, we investigated whether treatment with BG can improve impairment of memory and affect the cholinergic cells in the hippocampus of ischemia-induced memory deficit rats. The results showed that BG ameliorated the measures of stress-induced deficits of memory on the MWM, and the BG treated rats displayed an increased number of cholinergic positive neurons in the hippocampal CA1 and CA3 areas of the rats.
The extent of brain damage produced by MCAO is known to be dependent on the degree of the ischemic insult and its duration. One of the most critical factors is the period of occlusion. The damage produced by mild injury (30 min of MCAO) was confined to the striatum or cortex, whereas more than 2 h exposure to MCAO led to damage of the striatum, cortex and more remote areas, including the hippocampus [22]. In this study, the Morris water maze is well-established paradigm for evaluating deficits in hippocampal-dependent memory, and the MWM spatial learning task has been used for the validation of rodent models for neurocognitive disorders and for the evaluation of possible neurocognitive treatments [18,23-27]. In this study, the spatial memory tended to be improved in BG400 group during the training day (on the day 6), but not in the BG100 group. The data of the memory test demonstrated that BG protects the animals from the ischemia-induced decrease of the spatial learning and memory.
The neuroprotective effects of these natural drugs on the central acetylcholine system were also examined by the histochemistry of the hippocampal neurons. The degeneration of the cholinergic innervation from the basal forebrain to the hippocampal formation in the temporal lobe is thought to be one of the factors determining the progression of memory decay, both during normal aging and AD [28]. The best available marker for cholinergic neurons in the basal forebrain is ChAT activity. ChAT synthesizes the neurotransmitter acetylcholine in the basal forebrain and the cortex, hippocampus and amygdala. A significant reduction in ChAT activity in the postmortem brains of demented patients has been reported. In addition, there was a 20~50% decrease in ChAT activity in the hippocampus of the memory deficit rats. However, the present results show that BG exerts beneficial effects on cholinergic neurotransmission in the brain by increasing the hippocampal CA3 ChAT activities. However, our results showed that the levels of AchE in the hippocampus were not significantly different among all the groups.
NO-mediated mechanisms have been ascribed a role in cortical perfusion [29], learning and memory [30], as well as in neuronal plasticity [31,32]. Dysfunction of the neocortical and hippocampal cholinergic transmission has also been associated with reduced cortical cerebral blood flow and impaired learning and memory [33-35], suggesting a coupling of the basal forebrain cholinergic system. NADPH-diaphorase (NADPH-d) has been characterized biochemically and immunochemically as nitric oxide synthase [36-38]. Faber-Zuschratter et al. [39] demonstrated by ultrastructural studies that NADPH-d histochemical activity is located at both the pre- and postsynaptic membranes after long term potentiation (LTP). These mechanisms seem to be dependent on nitric oxide (NO) in the striatum, cerebellum and hippocampus [40-45]. Also, Chien et al. [46] reported that a NO synthesis inhibitor induced impaired learning and memory. Also, there is a specific decline of NOS-containing neurons in the hippocampal subregions CA1 and CA3 n brain form Alzheimer patients [47]. In agreement with the previous study, we found significant increased numbers of NADPH-d positive neurons in the hippocampal CA1 and CA3 areas and the dentate gyrus of the BG treated groups.
In summary, treatment with BG attenuated the ischemia-induced learning and memory deficits on the Morris water maze and treatment with BG had a protective effect against the ischemia-induced decreased number of cholinergic neurons. Also, treatment with BG increased NO synthesis in the hippocampus. BG is a good candidate for further investigations that may ultimately result in its clinical use. Further research that will examine the effects of BG activation on additional behavioral tasks will help to elucidate whether increasing the number of cholinergic neurons and nitric oxide synthesis may also facilitate other types of memory.
ACKNOWLEDGMENTS
This work was supported by Technology Development Program for Agriculture and Forestry (108071-03-3-HD120), Ministry for Food, Agriculture, Forestry and Fishenes, Republic of Korea.
References
1. Klijn CJ, Hankey GJ. American Stroke Association and European Stroke Initiative. Management of acute ischaemic stroke: new guidelines from the American Stroke Association and European Stroke Initiative. Lancet Neurol. 2003; 2:698–701. PMID: 14572738.


2. Fawcett JW, Asher RA. The glial scar and central nervous system repair. Brain Res Bull. 1999; 49:377–391. PMID: 10483914.


3. Silver J, Miller JH. Regeneration beyond the glial scar. Nat Rev Neurosci. 2004; 5:146–156. PMID: 14735117.


4. Wang Q, Sun AY, Simonyi A, Kalogeris TJ, Miller DK, Sun GY, Korthuis RJ. Ethanol preconditioning protects against ischemia/reperfusion-induced brain damage: role of NADPH oxidase-derived ROS. Free Radic Biol Med. 2007; 43:1048–1060. PMID: 17761301.


5. Mulvey JM, Dorsch NW, Mudaliar Y, Lang EW. Multimodality monitoring in severe traumatic brain injury: the role of brain tissue oxygenation monitoring. Neurocrit Care. 2004; 1:391–402. PMID: 16174941.


6. Gillis CN. Panax ginseng pharmacology: a nitric oxide link? Biochem Pharmacol. 1997; 54:1–8. PMID: 9296344.


7. O'Hara M, Kiefer D, Farrell K, Kemper K. A review of 12 commonly used medicinal herbs. Arch Fam Med. 1998; 7:523–536. PMID: 9821826.
8. Lee JH, Shen GN, Kim EK, Shin JH, Myung CS, Oh HJ, Kim DH, Roh SS, Cho W, Seo YB, Park YJ, Kang CW, Song GY. Preparation of black ginseng and its antitumor activity. Korean J Orient Physiol Pathol. 2006; 20:951–956.
9. Song GY, Oh HJ, Roh SS, Seo YB, Park YJ, Myung CS. Effect of black ginseng on body weight and lipid profiles in male rats fed normal diets. Korean J Pharmacogn. 2006; 50:381–385.
10. Yun TK. Experimental and epidemiological evidence on non-organ specific cancer preventive effect of Korean ginseng and identification of active compounds. Mutat Res. 2003; 523-524:63–74. PMID: 12628504.


11. Kim SN, Ha YW, Shin H, Son SH, Wu SJ, Kim YS. Simultaneous quantification of 14 ginsenosides in Panax ginseng C.A. Meyer (Korean red ginseng) by HPLC-ELSD and its application to quality control. J Pharm Biomed Anal. 2007; 45:164–170. PMID: 17560064.


12. Baek NI, Kim DS, Lee YH, Park JD, Lee CB, Kim SI. Ginsenoside Rh4, a genuine dammarane glycoside from Korean red ginseng. Planta Med. 1996; 62:86–87. PMID: 8720394.


13. Yun TK. Experimental and epidemiological evidence of the cancer-preventive effects of Panax ginseng C.A. Meyer. Nutr Rev. 1996; 54:S71–S81. PMID: 9110579.
14. Kim KT, Yoo KM, Lee JW, Eom SH, Hwang IK, Lee CY. Protective effect of steamed American ginseng (Panax quinquefolius L.) on V79-4 cells induced by oxidative stress. J Ethnopharmacol. 2007; 111:443–450. PMID: 17276636.


15. Kang KS, Kim HY, Yamabe N, Nagai R, Yokozawa T. Protective effect of sun ginseng against diabetic renal damage. Biol Pharm Bull. 2006; 29:1678–1684. PMID: 16880625.


16. Wargovich MJ. Colon cancer chemoprevention with ginseng and other botanicals. J Korean Med Sci. 2001; 16(Suppl):S81–S86. PMID: 11748382.


17. Sun BS, Gu LJ, Fang ZM, Wang CY, Wang Z, Lee MR, Li Z, Li JJ, Sung CK. Simultaneous quantification of 19 ginsenosides in black ginseng developed from Panax ginseng by HPLC-ELSD. J Pharm Biomed Anal. 2009; 50:15–22. PMID: 19394786.


18. D'Hooge R, De Deyn PP. Applications of the Morris water maze in the study of learning and memory. Brain Res Brain Res Rev. 2001; 36:60–90. PMID: 11516773.
19. Scherer-Singler U, Vincent SR, Kimura H, McGeer EG. Demonstration of a unique population of neurons with NADPH-diaphorase histochemistry. J Neurosci Methods. 1983; 9:229–234. PMID: 6363828.


20. Kim IB, Oh SJ, Chun MH. Neuronal nitric oxide synthase immunoreactive neurons in the mammalian retina. Microsc Res Tech. 2000; 50:112–123. PMID: 10891875.


21. Paxinos G, Watson C, Pennisi M, Topple A. Bregma, lambda and the interaural midpoint in stereotaxic surgery with rats of different sex, strain and weight. J Neurosci Methods. 1985; 13:139–143. PMID: 3889509.


22. Lee B, Choi Y, Kim H, Kim SY, Hahm DH, Lee HJ, Shim I. Protective effects of methanol extract of Acori graminei rhizoma and Uncariae Ramulus et Uncus on ischemia-induced neuronal death and cognitive impairments in the rat. Life Sci. 2003; 74:435–450. PMID: 14609722.


23. Torres JB, Assunção J, Farias JA, Kahwage R, Lins N, Passos A, Quintairos A, Trévia N, Diniz CW. NADPH-diaphorase histochemical changes in the hippocampus, cerebellum and striatum are correlated with different modalities of exercise and watermaze performances. Exp Brain Res. 2006; 175:292–304. PMID: 16763833.


24. Susswein AJ, Katzoff A, Miller N, Hurwitz I. Nitric oxide and memory. Neuroscientist. 2004; 10:153–162. PMID: 15070489.


25. Matsunaga K, Mukasa H. The effect of alcohol on the human memory. Arukoru Kenkyuto Yakubutsu Ison. 1986; 21:64–73. PMID: 3718332.
26. Luine VN, Jacome LF, Maclusky NJ. Rapid enhancement of visual and place memory by estrogens in rats. Endocrinology. 2003; 144:2836–2844. PMID: 12810538.


27. Sato T, Teramoto T, Tanaka K, Ohnishi Y, Irifune M, Nishikawa T. Effects of ovariectomy and calcium deficiency on learning and memory of eight-arm radial maze in middle-aged female rats. Behav Brain Res. 2003; 142:207–216. PMID: 12798282.


28. Du JY, Li XY, Zhuang Y, Wu XY, Wang T. Effects of acute mild and moderate hypoxia on human short memory. Space Med Med Eng (Beijing). 1999; 12:270–273. PMID: 11542709.
29. Iadecola C. Regulation of the cerebral microcirculation during neural activity: is nitric oxide the missing link? Trends Neurosci. 1993; 16:206–214. PMID: 7688160.


30. Medina JH, Izquierdo I. Retrograde messengers, long-term potentiation and memory. Brain Res Brain Res Rev. 1995; 21:185–194. PMID: 8866674.


31. Hawkins RD, Son H, Arancio O. Nitric oxide as a retrograde messenger during long-term potentiation in hippocampus. Prog Brain Res. 1998; 118:155–172. PMID: 9932440.
32. Zorumski CF, Izumi Y. Modulation of LTP induction by NMDA receptor activation and nitric oxide release. Prog Brain Res. 1998; 118:173–182. PMID: 9932441.
33. Bartus RT, Dean RL 3rd, Beer B, Lippa AS. The cholinergic hypothesis of geriatric memory dysfunction. Science. 1982; 217:408–414. PMID: 7046051.


34. Coyle JT, Price DL, DeLong MR. Alzheimer's disease: a disorder of cortical cholinergic innervation. Science. 1983; 219:1184–1190. PMID: 6338589.


35. Waite JJ, Holschneider DP, Scremin OU. Selective immunotoxin-induced cholinergic deafferentation alters blood flow distribution in the cerebral cortex. Brain Res. 1999; 818:1–11. PMID: 9914432.


36. Bredt DS, Snyder SH. Nitric oxide mediates glutamate-linked enhancement of cGMP levels in the cerebellum. Proc Natl Acad Sci USA. 1989; 86:9030–9033. PMID: 2573074.


37. Dawson TM, Bredt DS, Fotuhi M, Hwang PM, Snyder SH. Nitric oxide synthase and neuronal NADPH diaphorase are identical in brain and peripheral tissues. Proc Natl Acad Sci USA. 1991; 88:7797–7801. PMID: 1715581.


38. Hope BT, Michael GJ, Knigge KM, Vincent SR. Neuronal NADPH diaphorase is a nitric oxide synthase. Proc Natl Acad Sci USA. 1991; 88:2811–2814. PMID: 1707173.


39. Faber-Zuschratter H, Seidenbecher T, Reymann K, Wolf G. Ultrastructural distribution of NADPH-diaphorase in the normal hippocampus and after long-term potentiation. J Neural Transm. 1996; 103:807–817. PMID: 8872865.


40. Zhuo M, Laitinen JT, Li XC, Hawkins RD. On the respective roles of nitric oxide and carbon monoxide in long-term potentiation in the hippocampus. Learn Mem. 1998; 5:467–480. PMID: 10489262.


41. Daniel H, Hemart N, Jaillard D, Crepel F. Long-term depression requires nitric oxide and guanosine 3':5' cyclic monophosphate production in rat cerebellar Purkinje cells. Eur J Neurosci. 1993; 5:1079–1082. PMID: 7506617.
42. Daniel H, Levenes C, Crépel F. Cellular mechanisms of cerebellar LTD. Trends Neurosci. 1998; 21:401–407. PMID: 9735948.


43. Calabresi P, Centonze D, Gubellini P, Marfia GA, Pisani A, Sancesario G, Bernardi G. Synaptic transmission in the striatum: from plasticity to neurodegeneration. Prog Neurobiol. 2000; 61:231–265. PMID: 10727775.


44. Bon CL, Garthwaite J. On the role of nitric oxide in hippocampal long-term potentiation. J Neurosci. 2003; 23:1941–1948. PMID: 12629199.


45. Susswein AJ, Katzoff A, Miller N, Hurwitz I. Nitric oxide and memory. Neuroscientist. 2004; 10:153–162. PMID: 15070489.


46. Chien WL, Liang KC, Teng CM, Kuo SC, Lee FY, Fu WM. Enhancement of learning behaviour by a potent nitric oxide-guanylate cyclase activator YC-1. Eur J Neurosci. 2005; 21:1679–1688. PMID: 15845095.


47. Thorns V, Hansen L, Masliah E. nNOS expressing neurons in the entorhinal cortex and hippocampus are affected in patients with Alzheimer's disease. Exp Neurol. 1998; 150:14–20. PMID: 9514829.


Fig. 1
The latency to escape onto the hidden platform during the Morris water maze. The task was performed with 3 trials per day during 6 days for the acquisition test. The values are presented as means±S.E.M. *p<0.05 vs. the Normal group and #p<0.05, ##p<0.01 vs. the SAL+ISCH group.
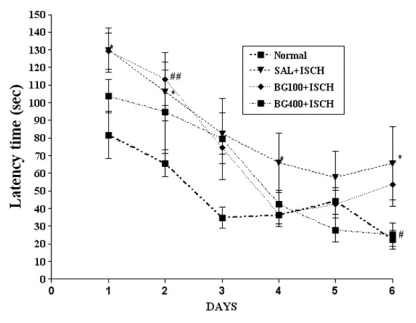
Fig. 2
(A) The number of cresyl violet stained neurons in the different hippocampal CA1,CA3 and DG areas of the experimental groups. Each value represents the mean±S.E.M. ***p<0.001 vs. the normal group and ##p<0.01 vs. the SAL+ISCH group. (B) Photographs showing the distribution of cresyl violet stained neurons in the hippocampus of the Normal (A), SAL+ISCH (B), BG100+ISCH (C) and BG400+ISCH (D). Sections were cut coronally at 30µm and the scale bar represents 200µm.
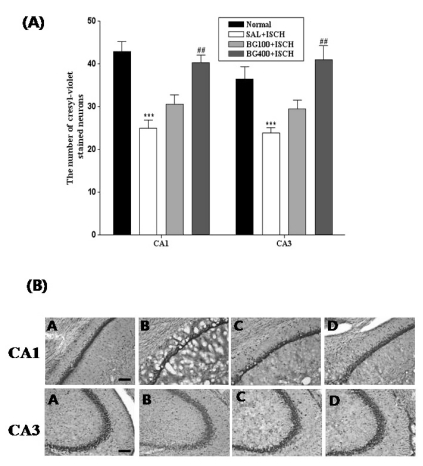
Fig. 3
(A) The number of choline acetyltransferase (ChAT) immunostained nuclei in the different hippocampal CA1 and CA3 areas of the experimental groups. Each value represents the mean±S.E.M. *p<0.05 vs. the normal group and #p<0.05 vs. the SAL+ISCH group. (B) Photographs showing the distribution of ChAT-immunoreactive cells in the hippocampus of the Normal (A), SAL+ISCH (B) and BG100+ISCH (C). Sections were cut coronally at 30µm and the scale bar represents 200µm.
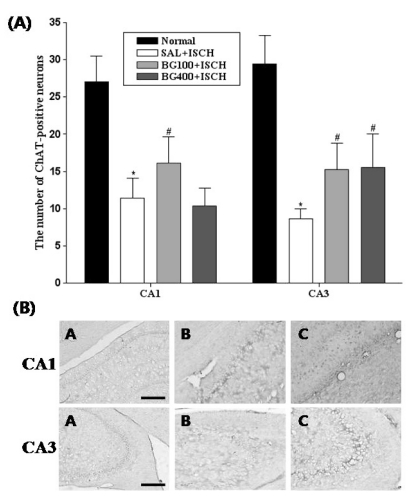
Fig. 4
(A) The number of NADPH-d positive neurons in the different hippocampal CA1 and CA3 areas of the experimental groups. Each value represents the mean±S.E.M. *p<0.05, ***p<0.001 vs. the normal group and #p<0.05, ##p<0.01 vs. the SAL+ISCH group. (B) Photographs showing the distribution of NADPH-d positive neurons in the hippocampus of the SHAM (A), SAL+ISCH (B), BG100+ISCH (C). Sections were cut coronally at 30µm and the scale bar represents 200µm.
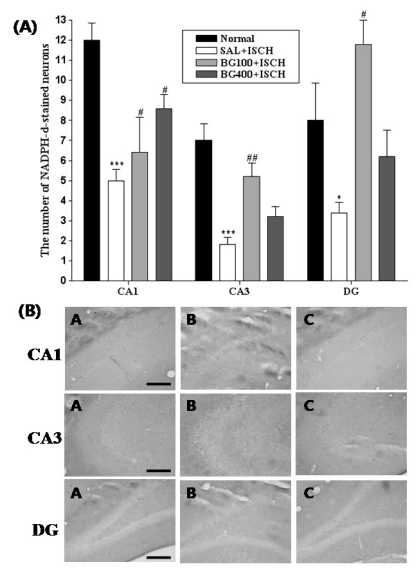