Abstract
B13, a ceramide analogue, is a ceramidase inhibitor and induces apoptosis to give potent anticancer activity. A series of thiourea B13 analogues was evaluated for their in vitro cytotoxic activities against human renal cancer Caki-2 and leukemic cancer HL-60 in the MTT assay. Some compounds (12, 15, and 16) showed stronger cytotoxicity than B13 and C6-ceramide against both tumor cell lines, and compound (12) gave the most potent activity with IC50 values of 36 and 9 μM, respectively. Molecular modeling of thiourea B13 analogues was carried out by comparative molecular field analysis (CoMFA) and comparative molecular similarity indices analysis (CoMSIA). We obtained highly reliable and predictive CoMSIA models with cross-validated q2 values of 0.707 and 0.753 and CoMSIA contour maps to show the structural requirements for potent activity. These data suggest that the amide group of B13 could be replaced by thiourea, that the stereochemistry of 1,3-propandiol may not be essential for activity and that long alkyl chains increase cytotoxicity.
References
1. Kolesnick RN, Goni FM, Alonso A. Compartmentalization of ceramide signaling: physical foundations and biological effects. J Cell Physiol. 2000; 184:285–300.


2. Merrill AH Jr, Sullards MC, Allegood JC, Kelly S, Wang E. Sphingolipidomics: high-throughput, structure-specific, and quantitative analysis of sphingolipids by liquid chromatography tandem mass spectrometry. Methods. 2005; 36:207–224.
3. Merrill AH Jr. De novo sphingolipid biosynthesis: a necessary, but dangerous, pathway. J Biol Chem. 2002; 277:25843–25846.


4. Hannun YA, Obeid LM. The ceramide-centric universe of lipid-mediated cell regulation: stress encounters of the lipid kind. J Biol Chem. 2002; 277:25847–25850.


5. Kolesnick RN. The therapeutic potential of modulating the ceramide sphingomyelin pathway. J Clin Invest. 2002; 110:3–8.
6. Hanada K, Kumagai K, Yasuda S, Miura Y, Kawano M, Fukasawa M, Nishijima M. Molecular machinery for non-vesicular trafficking of ceramide. Nature. 2003; 426:803–809.


7. Lee DW, Park SY, Ryu JS, Kim SH, Im C, Choi SH, Lee SE, Ko SK, Sohn UD. Relaxation effect of synthetic ceramide analogues in cat esophageal smooth muscle cells. Korean J Physiol Pharmacol. 2008; 12:137–142.


8. Kanto T, Kalinski P, Hunter OC, Lotze MT, Amoscatto AA. Ceramide mediates tumor-induced dendritic cell apoptosis. J Immunol. 2001; 167:3773–3784.


9. Noda S, Yoshimura S, Sawada M, Naganawa T, Iwama T, Nakashima S, Sakai N. Role of ceramide during cisplatin-induced apoptosis in C6 glioma cells. J Neurooncol. 2001; 52:11–21.
10. Selzner M, Bielawska A, Morse MA, Rudiger HA, Sindram D, Hannun YA, Clavien PA. Induction of apoptotic cell death and prevention of tumor growth by ceramide analogues in metastatic human colon cancer. Cancer Res. 2001; 61:1233–1240.
11. Zheng W, Kollmeyer J, Symolon H, Momin A, Munter E, Wang E, Kelly S, Allegood JC, Liu Y, Peng Q. Ceramides and other bioactive sphingolipid backbones in health and disease: lipidomic analysis, metabolism and roles in membrane structure, dynamics, signaling and autophagy. Biochim Biophys Acta. 2006; 1758:1864–1884.


12. Liu X, Elojeimy S, El-Zawahry AM, Holman DH, Bielawska A, Bielawski J, Rubinchik S, Guo GW, Dong JY, Keane T, Hannun YA, Tavassoli M, Norris JS. Modulation of ceramide metabolism enhances viral protein apoptin's cytotoxicity in prostate cancer. Mol Ther. 2006; 14:637–646.


13. Michael JM, Lavin MF, Watters DJ. Resistance to radiation-induced apoptosis in burkitt's lymphoma cells is associated with defective ceramide signaling. Cancer Res. 1997; 57:3600–3605.
14. Norris JS, Bielawska A, Day T, El-Zawahri A, ElOjeimy S, Hannun Y, Holman D, Hyer M, Landon C, Lowe S, Dong JY, McKillop J, Norris K, Obeid L, Rubinchik S, Tavassoli M, Tomlinson S, Voelkel-Johnson C, Liu X. Combined therapeutic use of AdGFPFasL and small molecule inhibitors of ceramide metabolism in prostate and head and neck cancers: a status report. Cancer Gene Ther. 2006; 13:1045–1051.


15. Senchenkov A, Litvak DA, Cabot MC. Targeting ceramide metabolism a strategy for overcoming drug resistance. J Natl Cancer Inst. 2001; 93:347–357.
16. Mathias S, Pena LA, Kolensnick RA. Signal transduction of stress via ceramide. Biochem J. 1998; 335:465–480.


17. Eto M, Bennouna J, Hunter OC. C16-ceramide accumulates following androgen ablation in LNCaP prostate cancer cells. Prostate. 2003; 57:66–79.
18. Pettus BJ, Chalfant CE, Hannun YA. Ceramide in apoptosis: an overview and current perspectives. Biochim Biophys Acta. 2002; 1585:114–125.


19. Bose R, Verheij M, Haimovitz FA, Scotto K, Fuks Z, Kolesnick R. Ceramide synthase mediates daunorubicin-induced apoptosis: an alternative mechanism for generating death signals. Cell. 1995; 82:405–414.


20. Hannun YA, Luberto C. Ceramide in the eukaryotic stress response. Trends Cell Biol. 2000; 10:73–80.


21. Nam EJ, Lee HS, Lee YJ, Joo WS, Maeng S, Im HI, Park CW, Kim YS. Ceramide is involved in MPP+ -induced cytotoxicity in human neuroblastoma cells. Korean J Physiol Pharmacol. 2002; 6:281–286.
22. Bieberich E, Hu B, Silva J, Mackinnon S, Yu RK, Fillmore H, Broaddus WC, Ottenbrite RM. Synthesis and characterization of novel ceramide analogs for induction of apoptosis in human cancer cells. Cancer Letters. 2002; 181:55–64.


23. Radin NS. Killing cancer cells by poly-drug elevation of ceramide levels: a hypothesis whose time has come? Eur J Biochem. 2001; 268:193–204.
24. Bielawska A, Greenberg MS, Perry D, Jayadev S, Shayman JA, McKay C, Hannun YA. (1S,2R)-D-erythro-2-(N-myristoylamino)-1-phenyl-1-propanol as an inhibitor of ceramidase. J Biol Chem. 1996; 271:12646–12654.


25. Raisova M, Goltz G, Bektas M, Bielawska A, Riebeling C, Hossini AM, Eberle J, Hannun YA, Orfanos CE, Geilen CC. Bcl-2 overexpression prevents apoptosis induced by ceramidase inhibitors in malignant melanoma and hacat keratinocytes. FEBS Lett. 2002; 516:47–52.


26. Samsel L, Zaidel G, Drumgoole HM, Jelovac D, Drachenberg C, Rhee JG, Brodie AM, Bielawska A, Smyth MJ. The ceramide analog, B13, induces apoptosis in prostate cancer cell lines and inhibits tumor growth in prostate cancer xenografts. Prostate. 2004; 58:382–393.


27. Holman DH, Turner LS, El-Zawahry A, Elojeimy S, Liu X, Bielawski J, Szulc ZM, Norris K, Zeidan YH, Hannun YA, Bielawska A, Norris JS. Lysosomotropic acid ceramidase inhibitor induces apoptosis in prostate cancer cells. Cancer Chemother Pharmacol. 2008; 61:231–242.


28. Kwon OH. Synthesis and anticancer activity of Aryl-1,3-propanediol thiourea derivatives. Chung-Ang Graduate School Master Thesis. 2002.
29. Park JG, Kramer BS, Steinberg SM, Carmichael J, Collins JM, Minna JD, Gazdar AF. Chemosensitivity testing of human colorectal carcinoma cell lines using a tetrazolium-based colorimetric assay. Cancer Research. 1987; 47:5875–5879.
30. Manthey JA, Guthrie N. Antiproliferative activities of citrus flavonoids against six human cancer cell lines. J Agric Food Chem. 2002; 50:5837–5843.


31. SYBYL Molecular Modeling Software. Tripos Inc.;St. Louis, USA: 2010.
Fig. 2.
Plot of the actual versus predicted activities against tumor cell lines. (A) Renal cancer Caki-2 cells. (B) Leukemic cancer HL-60 cells. The IC50 values were converted into pIC50 (–log IC50) values (: training set molecules, ▴: test set molecules).
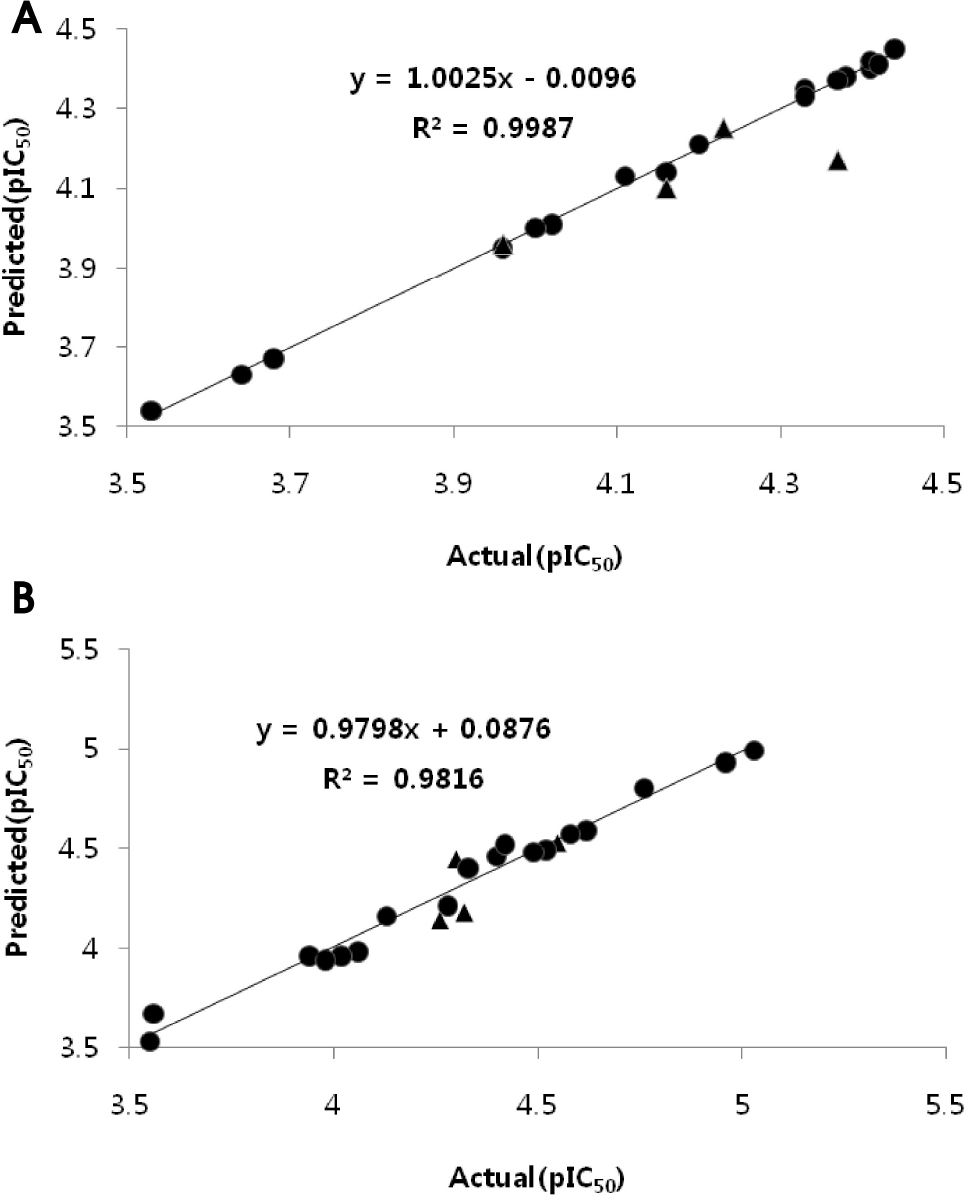
Fig. 3.
3D-contour maps of CoMSIA models. (A) CoMSIA model with steric and hydrogen bond donor fields for renal cancer Caki-2 cells. (B) CoMSIA model with steric and electrostatic fields for leukemic cancer HL-60 cells. Compound 12 is shown inside fields (green: sterically favorable; yellow: sterically unfavorable; cyan: hydrogen bond donor favorable; purple: hydrogen bond donor unfavorable; blue: positive charge favorable; red: negative charge favorable).
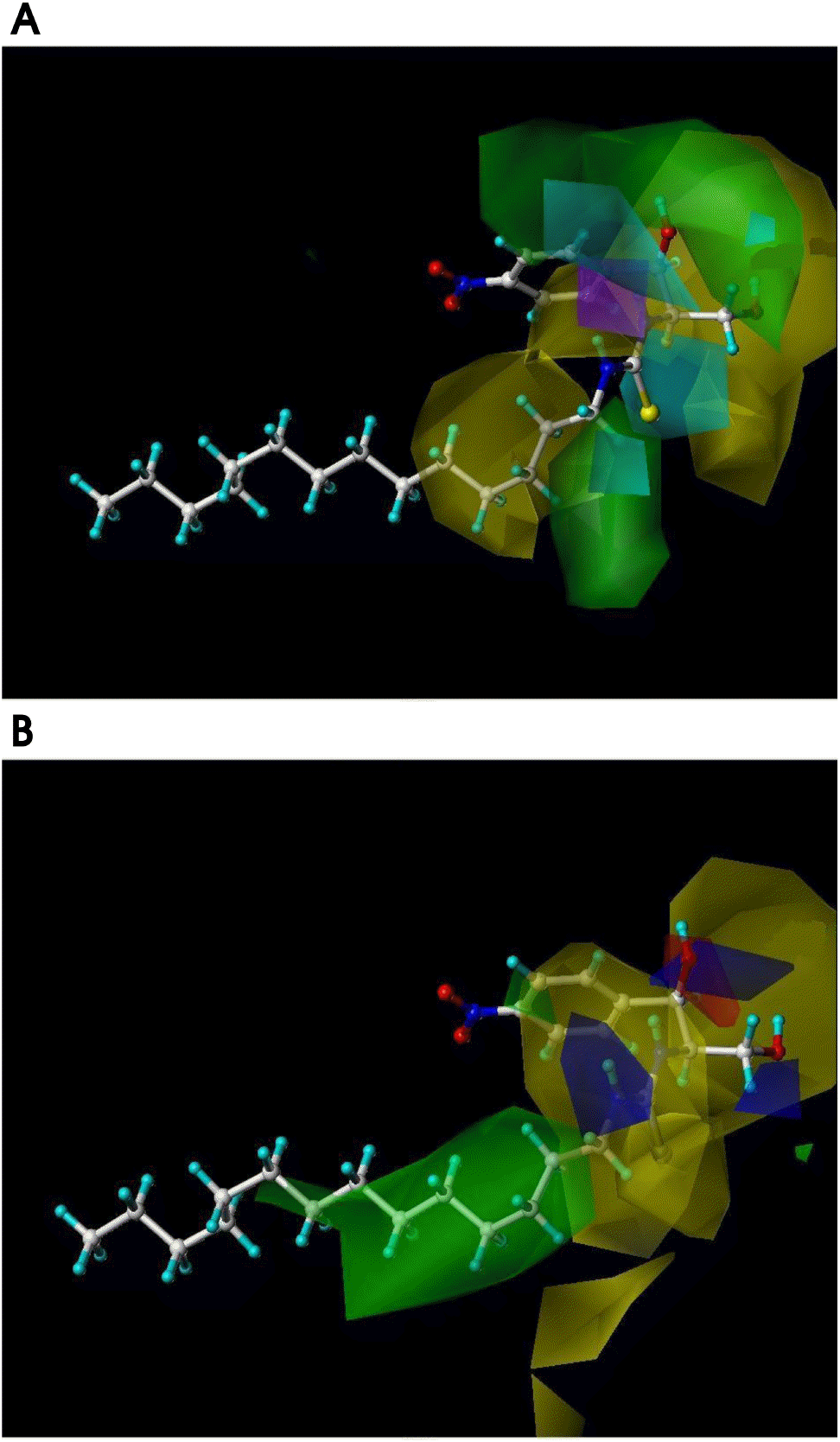
Table 2.
Statistical data for CoMFA and CoMSIA models
Fielda | q2b | Nc | SEPd | r2ncve | SEEf | Fg | r2predh | Contributionsa | |||
---|---|---|---|---|---|---|---|---|---|---|---|
S | E | D | A | ||||||||
Renal cancer Caki-2 cells | |||||||||||
CoMFA | |||||||||||
S | 0.430 | 3 | 0.268 | 0.992 | 0.031 | 592.153 | 1 | ||||
E | 0.299 | 3 | 0.297 | 0.919 | 0.101 | 53.162 | 1 | ||||
SE | 0.556 | 2 | 0.228 | 0.958 | 0.070 | 170.692 | 0.550 | 0.450 | |||
CoMSIA | |||||||||||
S | 0.465 | 3 | 0.260 | 0.978 | 0.053 | 204.852 | 1 | ||||
E | 0.479 | 5 | 0.277 | 0.996 | 0.026 | 533.148 | 1 | ||||
D | 0.312 | 4 | 0.306 | 0.900 | 0.116 | 29.402 | 1 | ||||
A | -0.127 | 1 | 0.353 | 0.510 | 0.232 | 16.681 | 1 | ||||
SE | 0.522 | 2 | 0.237 | 0.963 | 0.066 | 197.916 | 0.444 | 0.556 | |||
SD | 0.707 | 4 | 0.200 | 0.999 | 0.014 | 2377.228 | 0.975 | 0.581 | 0.419 | ||
DA | 0.269 | 3 | 0.303 | 0.935 | 0.090 | 67.381 | 0.429 | 0.571 | |||
Leukemia HL-60 cells | |||||||||||
CoMFA | |||||||||||
S | 0.555 | 2 | 0.297 | 0.961 | 0.087 | 186.554 | 1 | ||||
E | 0.414 | 4 | 0.366 | 0.984 | 0.061 | 199.632 | 1 | ||||
SE | 0.602 | 3 | 0.291 | 0.990 | 0.047 | 452.730 | 0.505 | 0.495 | |||
CoMSIA | |||||||||||
S | 0.606 | 2 | 0.280 | 0.941 | 0.108 | 120.378 | 1 | ||||
E | 0.579 | 2 | 0.289 | 0.897 | 0.143 | 65.338 | 1 | ||||
D | 0.485 | 1 | 0.309 | 0.688 | 0.241 | 35.279 | 1 | ||||
A | 0.232 | 1 | 0.378 | 0.619 | 0.266 | 25.978 | |||||
SE | 0.753 | 2 | 0.221 | 0.982 | 0.060 | 411.615 | 0.965 | 0.395 | 0.605 | ||
SD | 0.562 | 1 | 0.285 | 0.787 | 0.199 | 59.254 | 0.430 | 0.570 | |||
DA | 0.466 | 1 | 0.315 | 0.729 | 0.224 | 43.026 | 0.487 | 0.513 |
Table 3.
Actual and predicted activities (pIC50) of the training set
Compounds | Caki-2 cells | HL-60 cells | ||||
---|---|---|---|---|---|---|
Actuala | Predictedb | Residualc | Actuala | Predictedd | Residualc | |
1 | 4.02 | 4.01 | 0.01 | 4.06 | 3.98 | 0.08 |
2 | 4.33 | 4.35 | -0.02 | 4.13 | 4.16 | –0.03 |
3 | 3.53 | 3.54 | -0.01 | 3.55 | 3.53 | 0.02 |
4 | 4.00 | 4.00 | 0 | 4.02 | 3.96 | 0.06 |
5 | 4.11 | 4.13 | -0.02 | 4.28 | 4.21 | 0.07 |
6 | 4.41 | 4.40 | 0.01 | 4.52 | 4.49 | 0.03 |
7 | 4.38 | 4.38 | 0 | 4.49 | 4.48 | 0.01 |
8 | 3.53 | 3.54 | -0.01 | 3.56 | 3.67 | -0.11 |
9 | 3.96 | 3.95 | 0.01 | 4.40 | 4.46 | -0.06 |
10 | 4.33 | 4.33 | 0 | 4.33 | 4.40 | -0.07 |
11 | 4.20 | 4.21 | -0.01 | 4.62 | 4.59 | 0.03 |
12 | 4.44 | 4.45 | -0.01 | 5.03 | 4.99 | 0.04 |
13 | 3.68 | 3.67 | 0.01 | 3.94 | 3.96 | -0.02 |
14 | 4.16 | 4.14 | 0.02 | 4.42 | 4.52 | -0.1 |
15 | 4.41 | 4.42 | -0.01 | 4.76 | 4.80 | -0.04 |
16 | 4.42 | 4.41 | 0.01 | 4.96 | 4.93 | 0.03 |
17 | 3.64 | 3.63 | 0.01 | 3.98 | 3.94 | 0.04 |
18 | 4.37 | 4.37 | 0 | 4.58 | 4.57 | 0.01 |
Average | 0.01 | 0.05 |
Table 4.
Actual and predicted activities (pIC50) of the test set
Compounds | Caki-2 cells | HL-60 cells | ||||
---|---|---|---|---|---|---|
Actuala | Predictedb | Residualc | Actuala | Predictedd | Residualc | |
T1 | 4.37 | 4.17 | 0.2 | 4.26 | 4.14 | 0.12 |
T2 | 4.16 | 4.10 | 0.06 | 4.32 | 4.18 | 0.14 |
T3 | 4.23 | 4.25 | -0.02 | 4.30 | 4.45 | -0.15 |
T4 | 3.96 | 3.96 | 0 | 4.55 | 4.53 | 0.02 |
Average | 0.07 | 0.11 |