Abstract
Long-term potentiation (LTP) and long-term depression (LTD) have both been studied as mechanisms of ocular dominance plasticity in the rat visual cortex. In a previous study, we suggested that a developmental increase in serotonin [5-hydroxytryptamine (5-HT)] might be involved in the decline of LTP, since 5-HT inhibited its induction. In the present study, to further understand the role of 5-HT in a developmental decrease in plasticity, we investigated the effect of 5-HT on the induction of LTD in the pathway from layer 4 to layer 2/3. LTD was inhibited by 5-HT (10 μM) in 5-week-old rats. The inhibitory effect was mediated by activation of 5-HT2 receptors. Since 5-HT also regulates the development of visual cortical circuits, we also investigated the role of 5-HT on the development of inhibition. The development of inhibition was retarded by chronic (2 weeks) depletion of endogenous 5-HT in 5-week-old rats, in which LTD was reinstated. These results suggest that 5-HT regulates the induction of LTD directly via activation of 5-HT2 receptors and indirectly by regulating cortical development. Thus, the present study provides significant insight into the roles of 5-HT on the development of visual cortical circuits and on the age-dependent decline of long-term synaptic plasticity.
References
1. Bear MF. A synaptic basis for memory storage in the cerebral cortex. Proc Natl Acad Sci U S A. 1996; 93:13453–13459.


2. Martin SJ, Grimwood PD, Morris RG. Synaptic plasticity and memory: an evaluation of the hypothesis. Annu Rev Neurosci. 2000; 23:649–711.


3. Katz LC, Shatz CJ. Synaptic activity and the construction of cortical circuits. Science. 1996; 274:1133–1138.


4. Singer W. Development and plasticity of cortical processing architectures. Science. 1995; 270:758–764.


5. Bear MF, Rittenhouse CD. Molecular basis for induction of ocular dominance plasticity. J Neurobiol. 1999; 41:83–91.


6. Smith GB, Heynen AJ, Bear MF. Bidirectional synaptic mechanisms of ocular dominance plasticity in visual cortex. Philos Trans R Soc Lond B Biol Sci. 2009; 364:357–367.


7. Berardi N, Pizzorusso T, Maffei L. Critical periods during sensory development. Curr Opin Neurobiol. 2000; 10:138–145.


8. Perkins ATt, Teyler TJ. A critical period for long-term potentiation in the developing rat visual cortex. Brain Res. 1988; 439:222–229.
9. Dudek SM, Friedlander MJ. Developmental down-regulation of LTD in cortical layer IV and its independence of modulation by inhibition. Neuron. 1996; 16:1097–1106.


10. Pouille F, Scanziani M. Enforcement of temporal fidelity in pyramidal cells by somatic feed-forward inhibition. Science. 2001; 293:1159–1163.


11. Whittington MA, Traub RD. Interneuron diversity series: inhibitory interneurons and network oscillations in vitro. Trends Neurosci. 2003; 26:676–682.
12. Luhmann HJ, Prince DA. Control of NMDA receptor-mediated activity by GABAergic mechanisms in mature and developing rat neocortex. Brain Res Dev Brain Res. 1990; 54:287–290.


13. Nowak L, Bregestovski P, Ascher P, Herbet A, Prochiantz A. Magnesium gates glutamate-activated channels in mouse central neurones. Nature. 1984; 307:462–465.


14. Perkel DJ, Petrozzino JJ, Nicoll RA, Connor JA. The role of Ca2+ entry via synaptically activated NMDA receptors in the induction of long-term potentiation. Neuron. 1993; 11:817–823.
15. Bear MF, Kleinschmidt A, Gu QA, Singer W. Disruption of experience-dependent synaptic modifications in striate cortex by infusion of an NMDA receptor antagonist. J Neurosci. 1990; 10:909–925.


16. Mulkey RM, Malenka RC. Mechanisms underlying induction of homosynaptic long-term depression in area CA1 of the hippocampus. Neuron. 1992; 9:967–975.


17. Burchfiel JL, Duffy FH. Role of intracortical inhibition in deprivation amblyopia: reversal by microiontophoretic bicuculline. Brain Res. 1981; 206:479–484.


18. Hensch TK, Fagiolini M, Mataga N, Stryker MP, Baekkeskov S, Kash SF. Local GABA circuit control of experience-dependent plasticity in developing visual cortex. Science. 1998; 282:1504–1508.


19. Morales B, Choi SY, Kirkwood A. Dark rearing alters the development of GABAergic transmission in visual cortex. J Neurosci. 2002; 22:8084–8090.


20. Rozas C, Frank H, Heynen AJ, Morales B, Bear MF, Kirkwood A. Developmental inhibitory gate controls the relay of activity to the superficial layers of the visual cortex. J Neurosci. 2001; 21:6791–6801.


21. Gu Q. Neuromodulatory transmitter systems in the cortex and their role in cortical plasticity. Neuroscience. 2002; 111:815–835.


23. Gu Q, Singer W. Involvement of serotonin in developmental plasticity of kitten visual cortex. Eur J Neurosci. 1995; 7:1146–1153.


24. Kojic L, Gu Q, Douglas RM, Cynader MS. Serotonin facilitates synaptic plasticity in kitten visual cortex: an in vitro study. Brain Res Dev Brain Res. 1997; 101:299–304.
25. Edagawa Y, Saito H, Abe K. 5-HT1A receptor-mediated inhibition of long-term potentiation in rat visual cortex. Eur J Pharmacol. 1998; 349:221–224.


26. Edagawa Y, Saito H, Abe K. The serotonin 5-HT2 receptor-phospholipase C system inhibits the induction of long-term potentiation in the rat visual cortex. Eur J Neurosci. 2000; 12:1391–1396.
27. Edagawa Y, Saito H, Abe K. Endogenous serotonin contributes to a developmental decrease in long-term potentiation in the rat visual cortex. J Neurosci. 2001; 21:1532–1537.


28. Jang HJ, Cho KH, Kim HS, Hahn SJ, Kim MS, Rhie DJ. Age-dependent decline in supragranular long-term synaptic plasticity by increased inhibition during the critical period in the rat primary visual cortex. J Neurophysiol. 2009; 101:269–275.


29. Kim HS, Jang HJ, Cho KH, Hahn SJ, Kim MJ, Yoon SH, Jo YH, Kim MS, Rhie DJ. Serotonin inhibits the induction of NMDA receptor-dependent long-term potentiation in the rat primary visual cortex. Brain Res. 2006; 1103:49–55.


30. Yoon BJ, Smith GB, Heynen AJ, Neve RL, Bear MF. Essential role for a long-term depression mechanism in ocular dominance plasticity. Proc Natl Acad Sci U S A. 2009; 106:9860–9865.


31. Paxinos G, Watson C. The rat brain in stereotaxic coordinates. 4th ed.San Diego: Academic Press;1997.
32. Barnes NM, Sharp T. A review of central 5-HT receptors and their function. Neuropharmacology. 1999; 38:1083–1152.


33. Roerig B, Nelson DA, Katz LC. Fast synaptic signaling by nicotinic acetylcholine and serotonin 5-HT3 receptors in developing visual cortex. J Neurosci. 1997; 17:8353–8362.
34. Harvey JA, McMaster SE, Yunger LM. P-Chloramphetamine: Selective neurotoxic action in brain. Science. 1975; 187:841–843.
35. Moreau AW, Amar M, Le Roux N, Morel N, Fossier P. Serotoninergic fine-tuning of the excitation-inhibition balance in rat visual cortical networks. Cereb Cortex. 2010; 20:456–467.
36. Edagawa Y, Saito H, Abe K. Stimulation of the 5-HT1A receptor selectively suppresses NMDA receptor-mediated synaptic excitation in the rat visual cortex. Brain Res. 1999; 827:225–228.


37. Kimura F, Tsumoto T, Nishigori A, Yoshimura Y. Long-term depression but not potentiation is induced in Ca(2+)-chelated visual cortex neurons. Neuroreport. 1990; 1:65–68.
38. Foehring RC, van Brederode JF, Kinney GA, Spain WJ. Serotonergic modulation of supragranular neurons in rat sensorimotor cortex. J Neurosci. 2002; 22:8238–8250.


39. Haidkind R, Eller M, Kask A, Harro M, Rinken A, Oreland L, Harro J. Increased behavioural activity of rats in forced swimming test after partial denervation of serotonergic system by parachloroamphetamine treatment. Neurochem Int. 2004; 45:721–732.


40. Campo-Soria C, Chang Y, Weiss DS. Mechanism of action of benzodiazepines on GABAA receptors. Br J Pharmacol. 2006; 148:984–990.
41. Mattson MP, Maudsley S, Martin B. BDNF and 5-HT: a dynamic duo in age-related neuronal plasticity and neurode-generative disorders. Trends Neurosci. 2004; 27:589–594.


42. Juric DM, Miklic S, Carman-Krzan M. Monoaminergic neuronal activity up-regulates BDNF synthesis in cultured neonatal rat astrocytes. Brain Res. 2006; 1108:54–62.
43. Huang ZJ, Kirkwood A, Pizzorusso T, Porciatti V, Morales B, Bear MF, Maffei L, Tonegawa S. BDNF regulates the maturation of inhibition and the critical period of plasticity in mouse visual cortex. Cell. 1999; 98:739–755.


44. Kirkwood A. Serotonergic control of developmental plasticity. Proc Natl Acad Sci U S A. 2000; 97:1951–1952.


45. Maya Vetencourt JF, Sale A, Viegi A, Baroncelli L, De Pasquale R, O'Leary OF, Castren E, Maffei L. The antidepressant fluoxetine restores plasticity in the adult visual cortex. Science. 2008; 320:385–388.
46. D'Amato RJ, Blue ME, Largent BL, Lynch DR, Ledbetter DJ, Molliver ME, Snyder SH. Ontogeny of the serotonergic projection to rat neocortex: transient expression of a dense innervation to primary sensory areas. Proc Natl Acad Sci U S A. 1987; 84:4322–4326.
Fig. 1.
Inhibitory effect of 5-HT on the induction of LTD. (A) Effect of 5-HT on the induction of LTD in 3-week-old rats. Low-frequency stimulation (LFS) with 0.2-msec square pulses was applied to induce LTD under normal ACSF (open circle, ‘0.2 ms Con,’ n=5) and in the presence of 5-HT (10 μM, closed circle, ‘0.2 ms + 5-HT,’ n=9). LTD was also induced by stimulation with 0.1-msec square pulses (open square, ‘0.1 ms Con,’ n=5; closed square, ‘0.1 ms + 5-HT,’ n=9; respectively). Right panel plots individual data (symbols) and averages (thick solid lines). ∗p<0.05 vs. ‘Con’ for each stimulus pulse duration. (B) LFS with stimulus pulse duration of 0.2-msec was applied to the slices from 5-week-old rats (open circle, ‘0.2 ms Con,’ n=5). Concentration-dependent responses were obtained with four different concentrations of 5-HT at 0.01 μM (closed square, n=7), 0.1 μM (closed triangle, n=7), 1 μM (closed circle, n=8), and 10 μM (closed diamond, n=8). Right panel plots individual data (symbols) and averages (thick solid lines). ∗p<0.05 and ∗∗p<0.01 vs. ‘0.2 ms Con’. (C) Concentration-inhibition curve for the effect of 5-HT. The curve was fitted by sigmoid function (IC50=1.3 μM). Insets show average traces taken from a representative experiment at the indicated period.
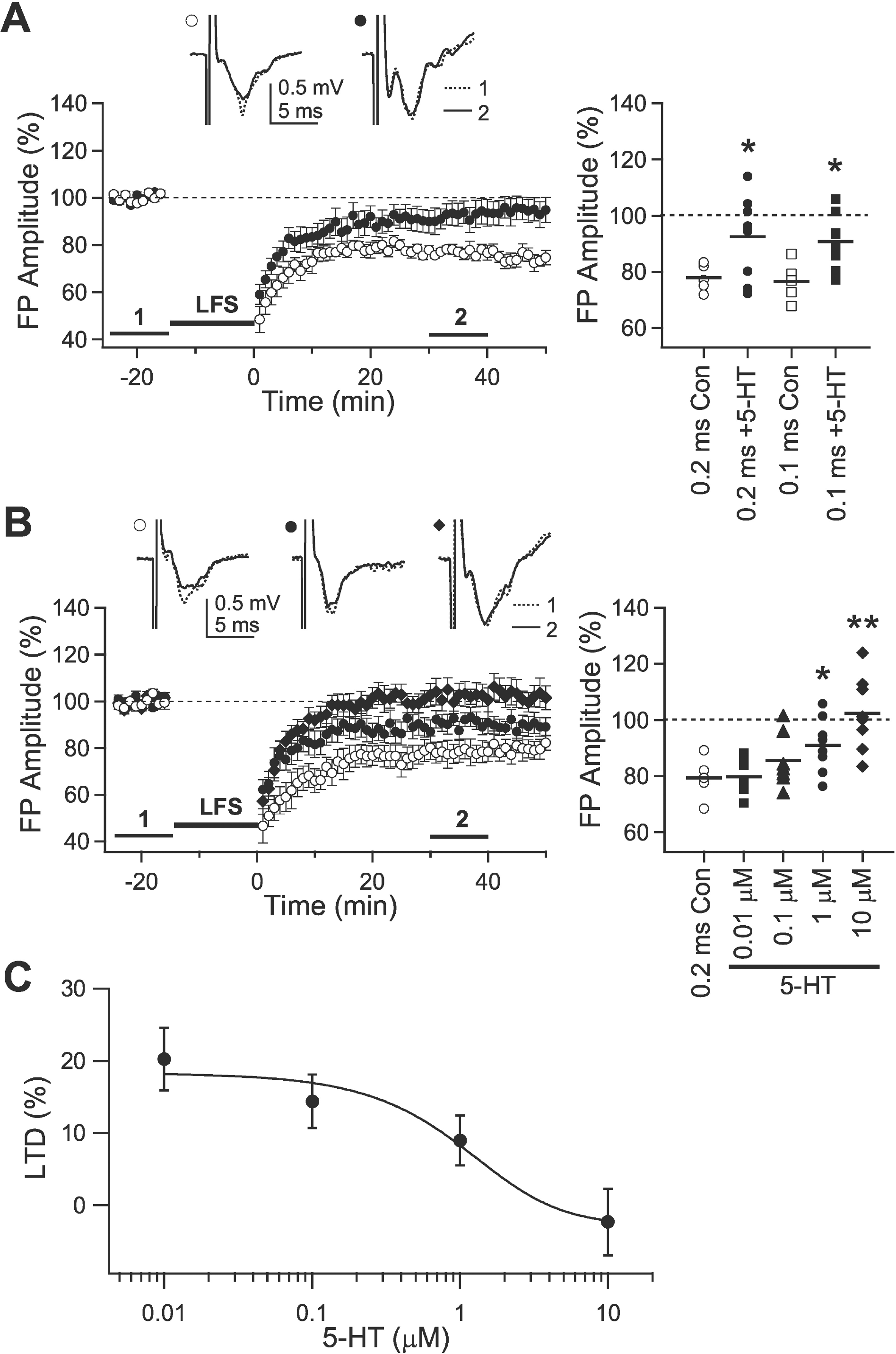
Fig. 2.
Effects of 5-HT receptor subtype-specific agonists and antagonists on the induction of LTD. LFS was applied with the stimulus duration of 0.2-msec to the slices from 5-week-old rats. (A) LFS was applied in the presence of 8-OH-DPAT (10 μM) (closed circle, ‘DPAT,’ n=10) or 5-HT (10 μM) + NAN-190 (10 μM) (open circle, ‘5-HT + NAN,’ n=7). (B) LFS was applied in the presence of DOI (10 μM) (closed circle, ‘DOI,’ n=9), 5-HT (10 μM) + mesulergine (10 μM) (open circle, ‘5-HT + Mes,’ n=7) or 5-HT (10 μM) + ketanserin (10 μM) (open square, ‘5-HT + Ket,’ n=8). (C) LFS was applied in the presence of 2-me-5-HT (30 μM) (closed circle, ‘2-me-5-HT,’ n=7). Insets show average traces taken from a representative experiment at the indicated period. Right panels plot individual data (symbols) and averages (thick solid lines). ∗p<0.05 and ∗∗p<0.01 vs. the baseline response.
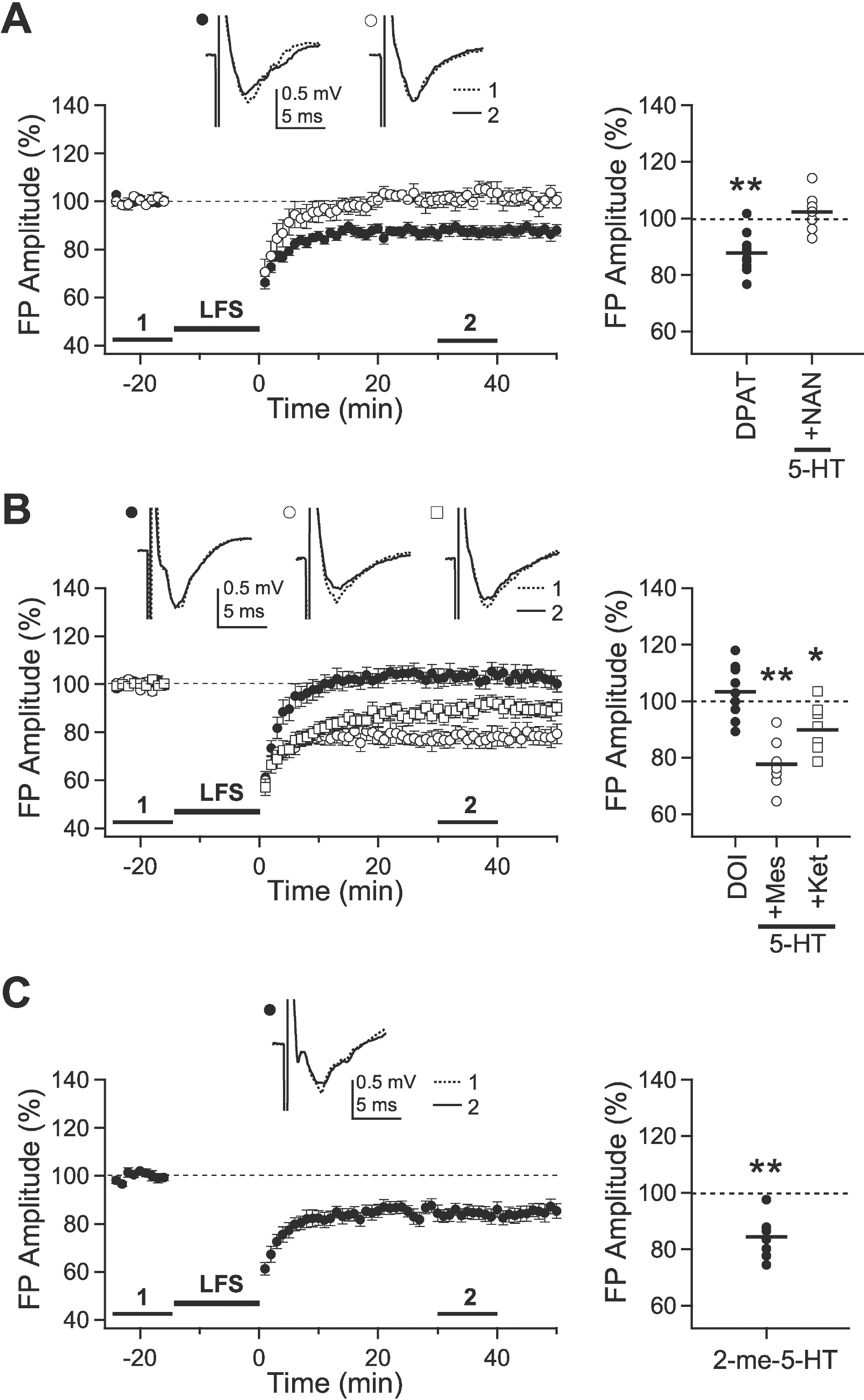
Fig. 3.
Effect of 5-HT depletion on the induction of LTD. Slices from 5-week-old rats were incubated in PCA (10 μM)-containing solution for 2 hours to deplete 5-HT acutely and then LFS was applied with 0.1-msec stimulation duration (closed circle, ‘acute PCA,’ n=9). To investigate the effect of chronic depletion of 5-HT, LFS was applied to the slices from 5-week-old rats, in which 5-HT was depleted for 2 weeks by intraperitoneal injection of PCA (8 mg/kg) (open square, ‘chronic PCA,’ n=10). Insets show average traces taken from a representative experiment at the indicated periods. Right panel plots individual data (symbols) and averages (thick solid lines). ∗∗∗p<0.001 vs. the baseline response.
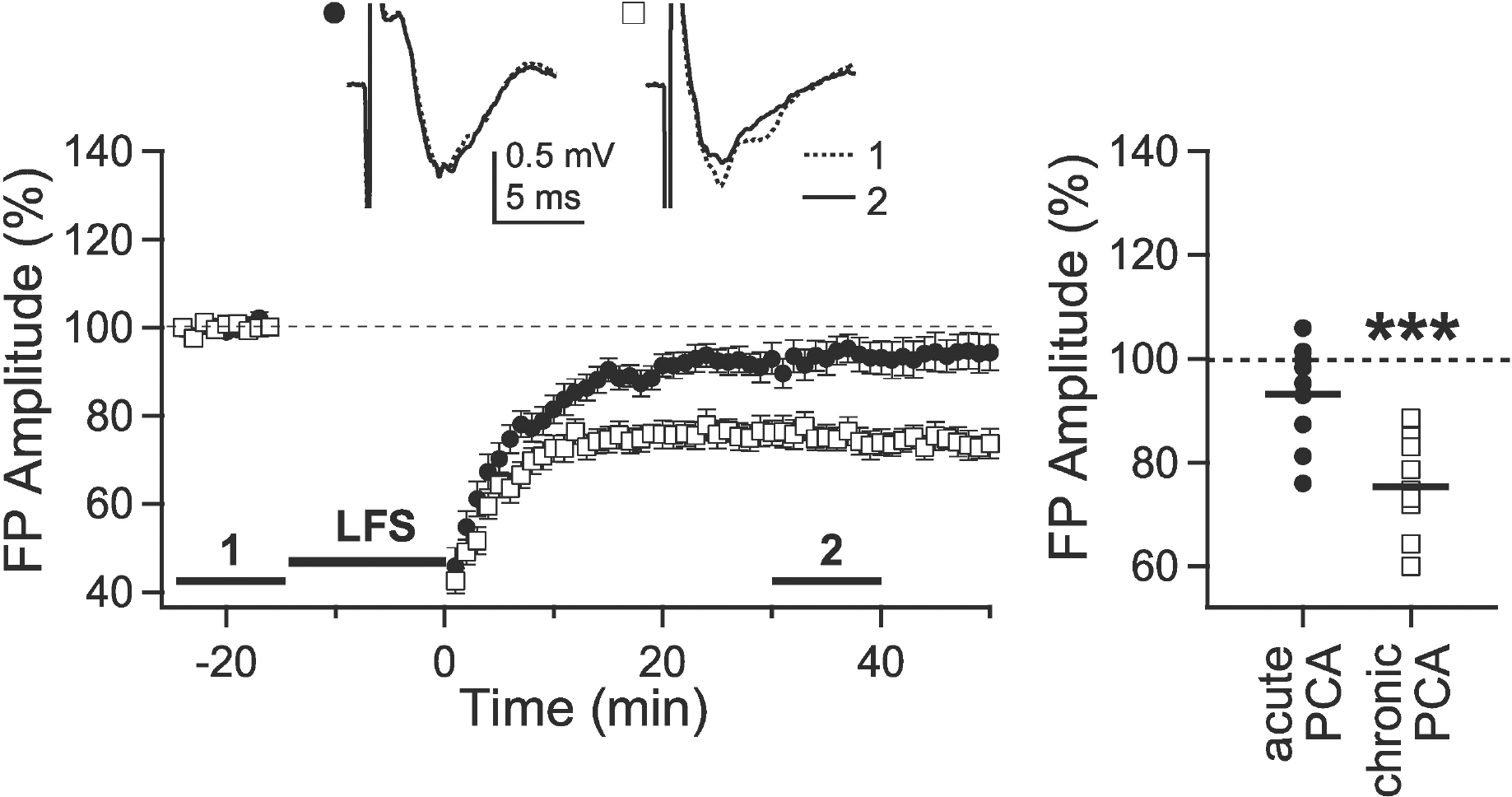
Fig. 4.
Effect of chronic 5-HT depletion on the development of the inhibitory network. 5-HT was depleted by single intraperitoneal injection of PCA (8 mg/kg) at 3 weeks of age and slices were taken at 5 weeks of age. (A) Experimental sequences. 20 mV of post-synaptic potential (PSP) and corresponding postsynaptic current (PSC) were recorded at –75 mV of membrane potential (left traces). Bath application of DNQX (20 μM) and APV (50 μM) eliminated PSP and PSC (center traces). At 0 mV of membrane potential, isolated inhibitory postsynaptic potential (IPSP) and current (IPSC) were recorded (right traces). CC: current clamp mode; VC: voltage clamp mode. (B) Averaged IPSPs (upper traces) and IPSCs (lower traces) showing differences between groups. Left traces: IPSPs and IPSCs recorded from slices of 3-week-old (thin line, n=8) and 5-week-old rats (thick line, n=8). Center traces: from slices of 5-week-old (thick line) and 5-week-old PCA-injected (thin line, n=8) rats. Right traces: from slices of 5-week-old rats (thick line) and slices of 5-week-old rats in which 5-HT was depleted by PCA incubation for 2 hours (thin line, n=8). (C) Individual data (symbols) and averages (thick lines) for PSP, PSC, IPSP and IPSC for each of the groups indicated in the lower panels. ∗p<0.05, ∗∗p<0.01, and ∗∗∗p<0.001 between groups linked by lines.
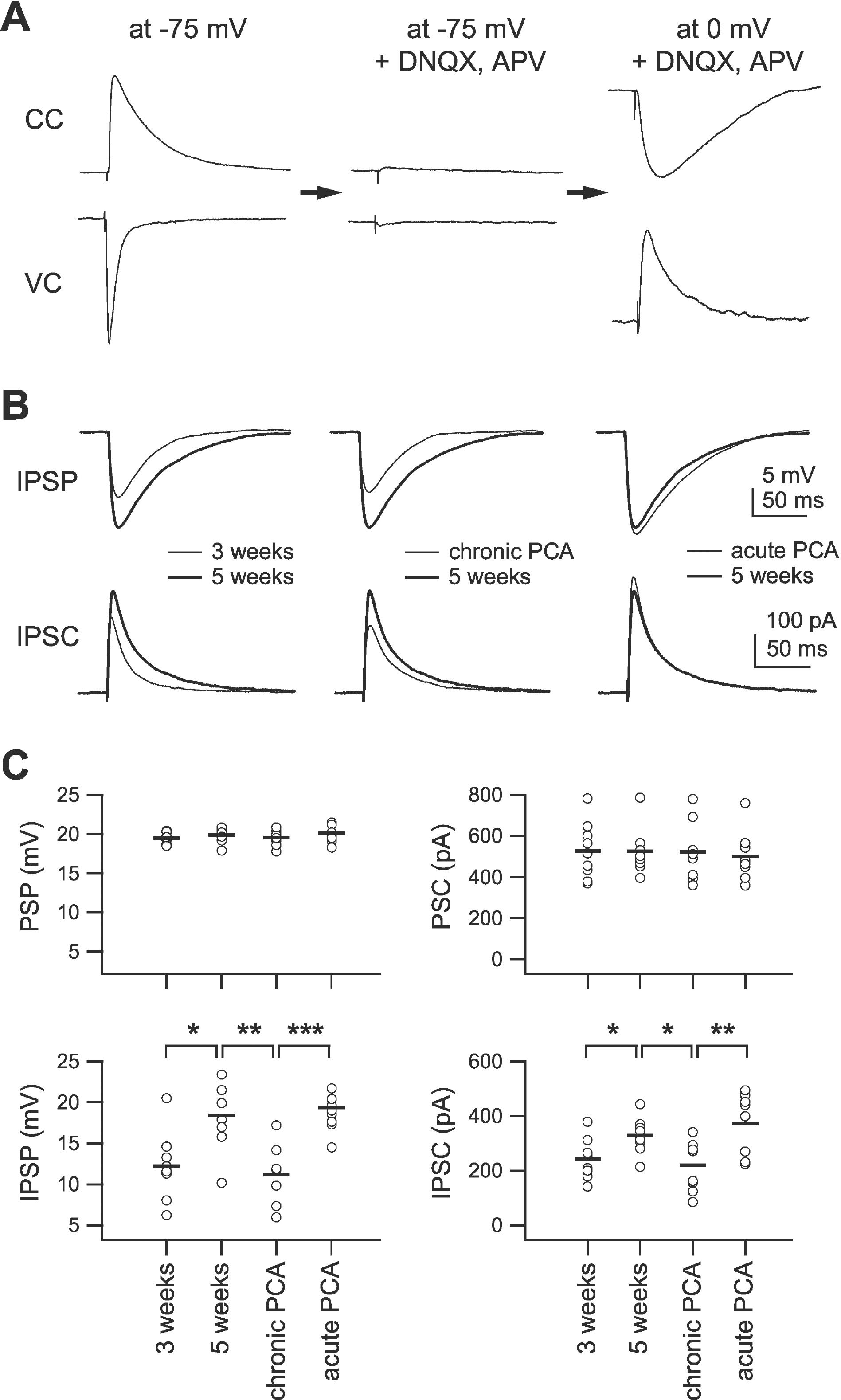