Abstract
5′-AMP-activated protein kinase (AMPK) is a heterotrimeric complex consisting of a catalytic (α) and two regulatory (β and γ) subunits. Two isoforms are known for catalytic subunit (α1, α2) and are encoded by different genes. To assess the metabolic effects of AMPKα1, we examined the effects of overexpression of adenoviral-mediated AMPKα1 in hyperlipidemic type 2 diabetic rats. The Otsuka Long-Evans Tokushima Fatty (OLETF) rat is an established animal model of type 2 diabetes that exhibits chronic and slowly progressive hyperglycemia and hyperlipidemia. Thirty five-week-old overt type 2 diabetic rats (n=10) were administered intravenously with Ad.AMPKα1. AMPK activity was measured by phosphorylation of acetyl CoA carboxlyase (ACC). To investigate the changes of gene expression related glucose and lipid metabolism, quantitative real-time PCR was performed with liver tissues. Overexpression of AMPKα1 showed that blood glucose concentration was decreased but that glucose tolerance was not completely recovered on 7th day after treatment. Plasma triglyceride concentration was decreased slightly, and hepatic triglyceride content was markedly reduced by decreasing expression of hepatic lipogenic genes. Overexpression of AMPKα1 markedly improved hepatic steatosis and it may have effective role for improving hepatic lipid metabolism in hyperlipidemic state.
References
Asilmaz E., Cohen P., Miyazaki M., Dobrzyn P., Ueki K., Fayzikhodjaeva G., Soukas AA., Kahn CR., Ntambi JM., Socci ND., Friedman JM. Site and mechanism of leptin action in a rodent form of congenital lipodystrophy. J Clin Invest. 113:414–424. 2004.


Cohen P., Ntambi JM., Friedman JM. Stearoyl-CoA desaturase-1 and the metabolic syndrome. Curr Drug Targets Immune Endocr Metabol Disord. 3:271–280. 2003.


Corton JM., Gillespie JG., Hawley SA., Hardie DG. 5-Aminoimidazole-4-carboxamide ribonucleoside. A specific method for activating AMP-activated protein kinase in intact cells? Eur J Biochem. 229:558–565. 1995.


Crawford RM., Treharne KJ., Arnaud-Dabernat S., Daniel JY., Foretz M., Viollet B., Mehta A. Understanding the molecular basis of the interaction between NDPK-A and AMPK alpha 1. Mol Cell Biol. 26:5921–5931. 2006.
Dobrzyn A., Ntambi JM. The role of stearoyl-CoA desaturase in body weight regulation. Trends Cardiovasc Med. 14:77–81. 2004.


Foretz M., Ancellin N., Andreelli F., Saintillan Y., Grondin P., Kahn A., Thorens B., Vaulont S., Viollet B. Short-term overexpression of a constitutively active form of AMP-activated protein kinase in the liver leads to mild hypoglycemia and fatty liver. Diabetes. 54:1331–1339. 2005.


Fryer LG., Parbu-Patel A., Carling D. Protein kinase inhibitors block the stimulation of the AMP-activated protein kinase by 5-amino-4-imidazolecarboxamide riboside. FEBS Lett. 531:189–192. 2002.


Hardie DG. AMP-activated protein kinase: a master switch in glucose and lipid metabolism. Rev Endocr Metab Disord. 5:119–125. 2004.


Hardie DG., Carling D. The AMP-activated protein kinase. Fuel gauge of the mammalian cell? Eur J Biochem. 246:259–273. 1997.


Hardie DG., Carling D., Carlson M. The amp-activated/snf1 protein kinase subfamily: Metabolic Sensors of the Eukaryotic Cell? Annu Rev Biochem. 67:821–855. 1998.


Hardie DG., Pan DA. Regulation of fatty acid synthesis and oxidation by the AMP-activated protein kinase. Biochem Soc Trans. 30:1064–1070. 2002.


Hardie DG., Scott JW., Pan DA., Hudson ER. M.anagement of cellular energy by the AMP-activated protein kinase system. FEBS Lett. 546:113–120. 2003.


Kawano K., Hirashima T., Mori S., Kurosumi M., Saitoh Y., Natori T. Spontaneous long-term hyperglycemic rat with diabetic complications. Otsuka Long-Evans Tokushima fatty (OLETF) strain. Diabetes. 41:1422–1428. 1992.


Minokoshi Y., Kim YB., Peroni OD., Fryer LG., Muller C., Carling D., Kahn BB. Leptin stimulates fatty-acid oxidation by activating AMP-activated protein kinase. Nature. 415:339–343. 2002.


Muoio DM., Seefeld K., Witters LA., Coleman RA. AMP-activated kinase reciprocally regulates triacylglycerol synthesis and fatty acid oxidation in liver and muscle: evidence that glycerol-3-phosphate acyltransferase is a novel target. Biochem J. 338:783–791. 1999.
Musi N., Hayashi T., Fujii N., Hirshman MF., Witters LA., Goodyear LJ. AMP-activated protein kinase activity and glucose uptake in rat skeletal muscle. Am J Physiol Endocrinol Metab. 280:E677–E684. 2001.


Reiter AK., Bolster DR., Crozier SJ., Kimball SR., Jefferson LS. Repression of protein synthesis and mTOR signaling in rat liver mediated by the AMPK activator aminoimidazole carboxamide ribonucleoside. Am J Physiol Endocrinol Metab. 288:E980–E988. 2005.


Pencek RR., Shearer J., Camacho RC., James FD., Lacy DB., Fueger PT., Donahue EP., Snead W., Wasserman DH. 5-Aminoimidazole-4-carboxamide-1-β-d-ribofuranoside causes acute hepatic insulin resistance in vivo. Diabetes. 54:355–360. 2005.
Rutter GA., Xavier GDS., Leclerc I. Roles of 5′-AMP-activated protein kinase (AMPK) in mammalian glucose homoeostasis. Biochem J. 375:1–16. 2003.


Saha AK., Avilucea PR., Ye JM., Assifi MM., Kraegen EW., Ruderman NB. Pioglitazone treatment activates AMP-activated protein kinase in rat liver and adipose tissue in vivo. Biochem Biophys Res Commun. 314:580–585. 2004.


Thong FS., Graham TE. The putative roles of adenosine in insulin-and exercise-mediated regulation of glucose transport and glycogen metabolism in skeletal muscle. Can J Appl Physiol. 27:152–178. 2002.
Winder WW., Hardie D. AMP-activated protein kinase, a metabolic master switch: possible roles in type 2 diabetes. Am J Physiol. 277:E1–10. 1999.
Winder WW., Wilson HA., Hardie DG., Rasmussen BB., Hutber CA., Call GB., Clayton RD., Conley LM., Yoon S., Zhou B. Phosphorylation of rat muscle acetyl-CoA carboxylase by AMP-activated protein kinase and protein kinase. A J Appl Physiol. 82:219–225. 1997.
Wojtaszewski JF., Jorgensen SB., Hellsten Y., Hardie DG., Richter EA. Glycogen-dependent effects of 5-aminoimidazole-4-carboxamide (AICA)-riboside on AMP-activated protein kinase and glycogen synthase activities in rat skeletal muscle. Diabetes. 51:284–292. 2002.
Yamauchi T., Kamon J., Minokoshi Y., Ito Y., Waki H., Uchida S., Yamashita S., Noda M., Kita S., Ueki K., Eto K., Akanuma Y., Froguel F., Foufelle F., Ferre P., Carling D., Kimura S., Nagai R., Kahn BB., Kadowaki T. Adiponectin stimulates glucose utilization and fatty-acid oxidation by activating AMP-activated protein kinase. Nat Med. 8:1288–1295. 2002.


Fig. 1.
Activity and expression of AMPK by Ad.AMPKα1 injection. (A) Non-radioactive AMPK activity assay. AMPK activity in the liver was measured on day 0 (0 day) and 7 (7 days) after Ad.AMPKα1 injection, and for the positive control with added AMPK recombinant protein (+AMPK). (B) Western blot analysis of phospho-ACC, phospho-AMPKα, AMPKα and myc-tagged AMPKα1 levels in liver on day 0 (0 day) and 7 (7 days) after Ad.AMPKα1 injection into OLETF rats. LETO, lean nondiabetic counterpart rats.
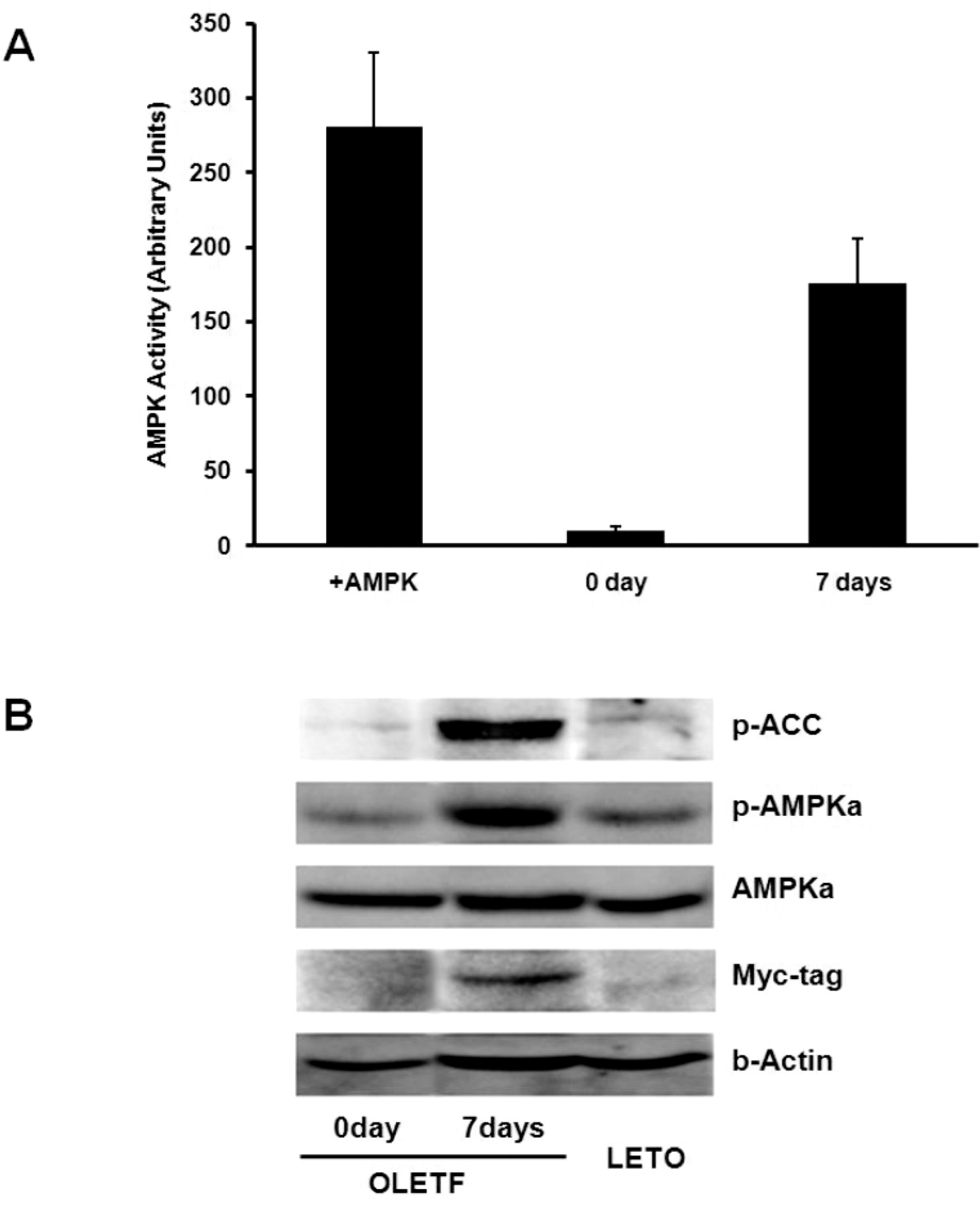
Fig. 2.
Ad.AMPKα1 alters glucose metabolism. (A) Effect of Ad.AMPKα1 on glucose tolerance. Glucose tolerance was measured on days 7 after injection of Ad.lacZ (Ad.lacZ) or Ad.AMPKα1 (Ad.AMPKα1) into OLETF rats. LETO rats were employed as nondiabetic counterpart. (B) Response of insulin secretion to glucose loading. Plasma concentrations of insulin were measured on the same time of assessing blood glucose. (C) Quantitative RT-PCR analysis of enzymes related to gluconeogenesis and glycolysis. ∗Significantly different from Ad.lacZ-injected OLETF rats (p<0.05).
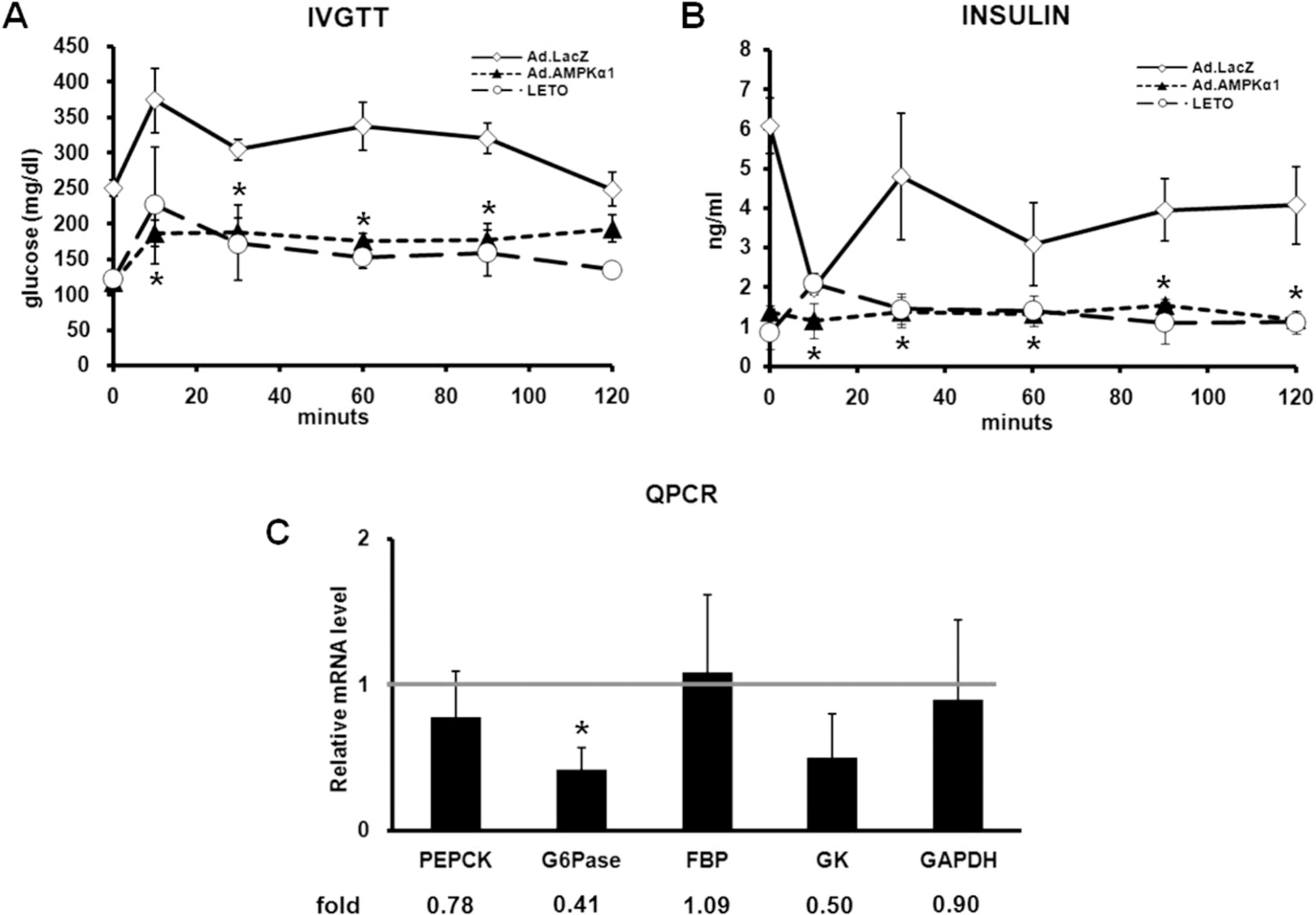
Fig. 3.
Ad.AMPKα1 decreases lipid content in liver. (A) Sudan Black B stained liver cryostat section from normal LETO rats, Ad.lacZ-injected OLETF rats (Ad.lacZ) and Ad.AMPKα1-injected OLETF rats (Ad.AMPKα1). Magnification ×200. The liver of Ad.lacZ-injected OLETF rat shows accumulation of large fat globules (Black). The liver of Ad.AMPKα1-injected OLETF rat shows marked decrease of fat globules. (B) Quantitative RT-PCR analysis of enzymes related to lipid synthesis. (C) Western blot analysis of SCD1 and HMGCR in liver on day 7 after injection. ∗Significantly different from Ad.lacZ-injected OLETF rats (p<0.05).
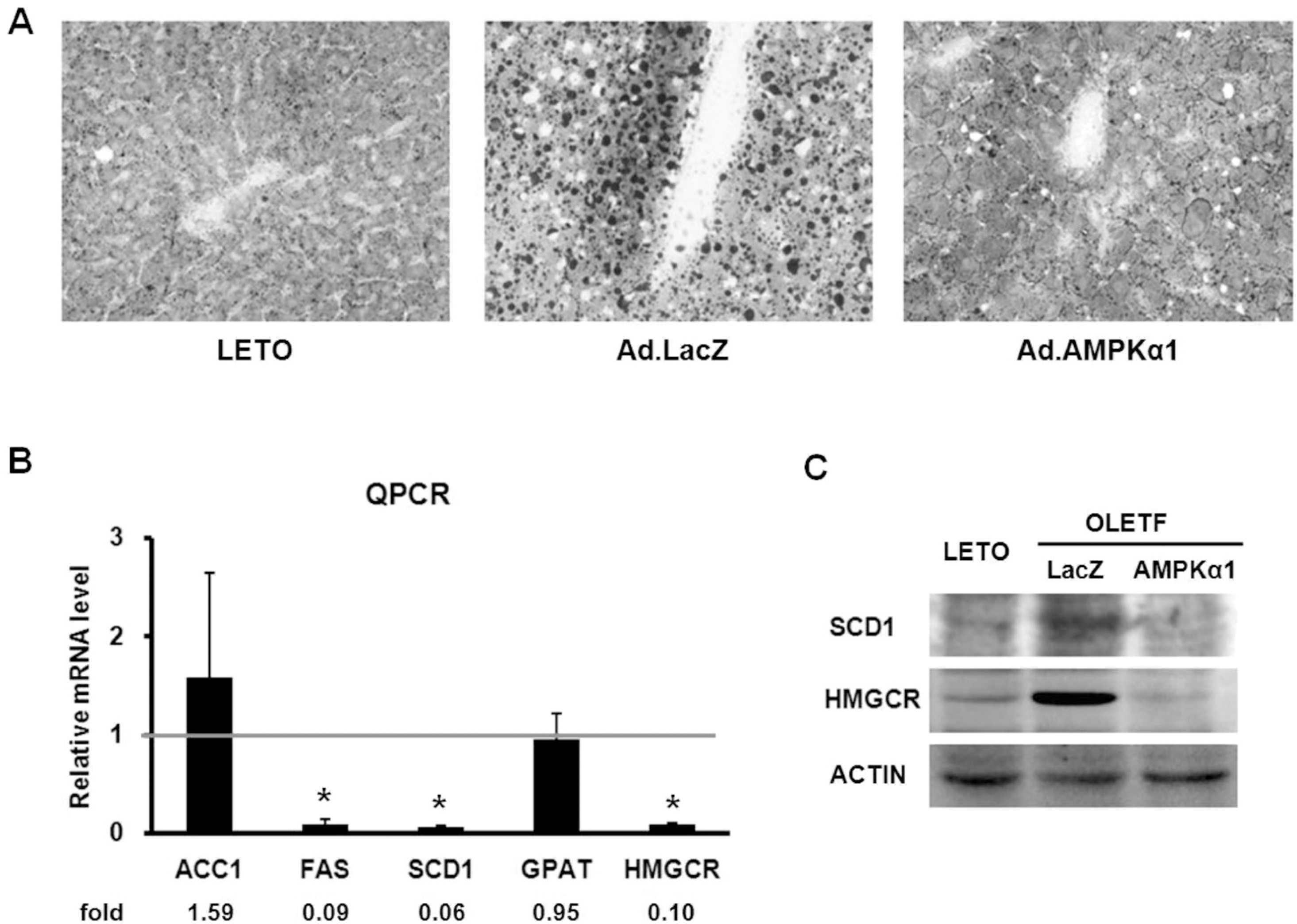
Table 1.
Metabolic characteristics of Ad.AMPKα1-injected OLETF rats
Parameter | OLETF | LETO | |
---|---|---|---|
Ad.LacZ | Ad.AMPKα1 | ||
Glucose (mg/dl) | 251±21 | 117±11∗∗ | 123±9∗ |
Insulin (ng/ml) | 6.08±1.42 | 1.37±0.82∗∗ | 0.84±0.34∗∗ |
HOMA index | 81.5±22.3 | 8.8±7.7∗∗ | 5.4±2.0∗∗ |
Plasma triglyceride (mg/dl) | 367±41 | 272±74∗ | 68±14∗∗ |
Plasma FFA (uEq/l) | 418±115 | 512±88 | 354±52 |
Plasma cholesterol (mg/dl) | 150±34 | 188±13∗ | 55±3∗ |
Liver triglyceride (mg/g liver wt) | 95.6±13.7 | 62.5±10.2∗ | 72.2±23.2 |
Liver cholesterol (mg/g liver wt) | 10.6±1.1 | 9.0±1.2 | 8.3±1.6 |