Abstract
Human bone marrow mesenchymal stem cells (hBM-MSCs) represent a potentially valuable cell type for clinical therapeutic applications. The present study was designed to evaluate the effect of long-term culturing (up to 10th passages) of hBM-MSCs from eight individual amyotrophic lateral sclerosis (ALS) patients, focusing on functional ion channels. All hBM-MSCs contain several MSCs markers with no significant differences, whereas the distribution of functional ion channels was shown to be different between cells. Four types of K+ currents, including noise-like Ca+2-activated K+ current (IKCa), a transient outward K+ current (Ito), a delayed rectifier K+ current (IKDR), and an inward-rectifier K+ current (Kir) were heterogeneously present in these cells, and a TTX-sensitive Na+ current (INa,TTX) was also recorded. In the RT-PCR analysis, Kv1.1, heag1, Kv4.2, Kir2.1, MaxiK, and hNE-Na were detected. In particular, INa,TTX showed a significant passage-dependent increase. This is the first report showing that functional ion channel profiling depend on the cellular passage of hBM-MSCs
REFERENCES
Balana B., Nicoletti C., Zahanich I., Graf EM., Christ T., Boxberger S., Ravens U. 5-Azacytidine induces changes in electrophysiological properties of human mesenchymal stem cells. Cell Res. 16:949–960. 2006.


Banfi A., Muraglia A., Dozin B., Mastrogiacomo M., Cancedda R., Quarto R. Proliferation kinetics and differentiation potential of ex vivo expanded human bone marrow stromal cells: Implications for their use in cell therapy. Exp Hematol. 28:707–715. 2000.
Baxter MA., Wynn RF., Jowitt SN., Wraith JE., Fairbairn LJ., Bellantuono I. Study of telomere length reveals rapid aging of human marrow stromal cells following in vitro expansion. Stem Cells. 22:675–682. 2004.


Biagiotti T., D'Amico M., Marzi I., Di Gennaro P., Arcangeli A., Wanke E., Olivotto M. Cell renewing in neuroblastoma: electrophy-siological and immunocytochemical characterization of stem cells and derivatives. Stem Cells. 24:443–453. 2006.


Bonab MM., Alimoghaddam K., Talebian F., Ghaffari SH., Ghavamzadeh A., Nikbin B. Aging of mesenchymal stem cell in vitro. BMC Cell Biol. 7:14. 2006.


Deng XL., Lau CP., Lai K., Cheung KF., Lau GK., Li GR. Cell cycle-dependent expression of potassium channels and cell proliferation in rat mesenchymal stem cells from bone marrow. Cell Prolif. 40:656–670. 2007.


Deng XL., Sun HY., Lau CP., Li GR. Properties of ion channels in rabbit mesenchymal stem cells from bone marrow. Biochem Biophys Res Commun. 348:301–309. 2006.


Friedenstein AJ., Deriglasova UF., Kulagina NN., Panasuk AF., Rudakowa SF., Luria EA., Ruadkow IA. Precursors for fibroblasts in different populations of hematopoietic cells as detected by the in vitro colony assay method. Exp Hematol. 2:83–92. 1974.
Hannouche D., Terai H., Fuchs JR., Terada S., Zand S., Nasseri BA., Petite H., Sedel L., Vacanti JP. Engineering of implantable cartilaginous structures from bone marrow-derived mesenchymal stem cells. Tissue Eng. 13:87–99. 2007.


Karnieli O., Izhar-Prato Y., Bulvik S., Efrat S. Generation of insulin-producing cells from human bone marrow mesenchymal stem cells by genetic manipulation. Stem Cells. 25:2837–2844. 2007.


Li GR., Deng XL., Sun H., Chung SS., Tse HF., Lau CP. Ion channels in mesenchymal stem cells from rat bone marrow. Stem Cells. 24:1519–1528. 2006.


Li GR., Sun H., Deng X., Lau CP. Characterization of ionic currents in human mesenchymal stem cells from bone marrow. Stem Cells. 23:371–382. 2005.


MacFarlane SN., Sontheimer H. Changes in ion channel expression accompany cell cycle progression of spinal cord astrocytes. Glia. 30:39–48. 2000.


Mazzini L., Mareschi K., Ferrero I., Vassallo E., Oliveri G., Boccaletti R., Testa L., Livigni S., Fagioli F. Autologous mesenchymal stem cells: clinical applications in amyotrophic lateral sclerosis. Neurol Res. 28:523–526. 2006.


Orlic D., Kajstura J., Chimenti S., Jakoniuk I., Anderson SM., Li B., Pickel J., McKay R., Nadal-Ginard B., Bodine DM., Leri A., Anversa P. Bone marrow cells regenerate infarcted myocardium. Nature. 410:701–705. 2001.


Ouadid-Ahidouch H., Roudbaraki M., Delcourt P., Ahidouch A., Joury N., Prevarskaya N. Functional and molecular identification of intermediate-conductance Ca2+-activated K+ channels in breast cancer cells: association with cell cycle progression. Am J Physiol Cell Physiol. 287:C125–134. 2004.
Park KS., Jung KH., Kim SH., Kim KS., Choi MR., Kim Y., Chai YG. Functional expression of ion channels in mesenchymal stem cells derived from umbilical cord vein. Stem Cells. 25:2044–2052. 2007.


Rombouts WJ., Ploemacher RE. Primary murine MSC show highly efficient homing to the bone marrow but lose homing ability following culture. Leukemia. 17:160–170. 2003.


Stenderup K., Justesen J., Clausen C., Kassem M. Aging is associated with decreased maximal life span and accelerated senescence of bone marrow stromal cells. Bone. 33:919–926. 2003.


Tao R., Lau CP., Tse HF., Li GR. Functional ion channels in mouse bone marrow mesenchymal stem cells. Am J Physiol Cell Physiol. 293:C1561–1567. 2007.


Fig. 1.
Characterization of hBM-MSCs. (A) Flow cytometry analysis showing that hBM-MSCswere positive for CD29, CD44, CD105, and CD73 and were negative for CD34, CD45, and HLA-DR. The table shows mean values (%). (B) hBM-MSCs expressed markers for OPN, LIFR, ABCG2, CXCR4, CD44, collagen X, collagen1 and alpha1. The hBM-MSCs RNAs were obtained from different donors (I and II, n=4) (C) Differentiation capacity of hBM-MSCs to adipocytes (upper) and osteoblasts (lower). 200× magnification.
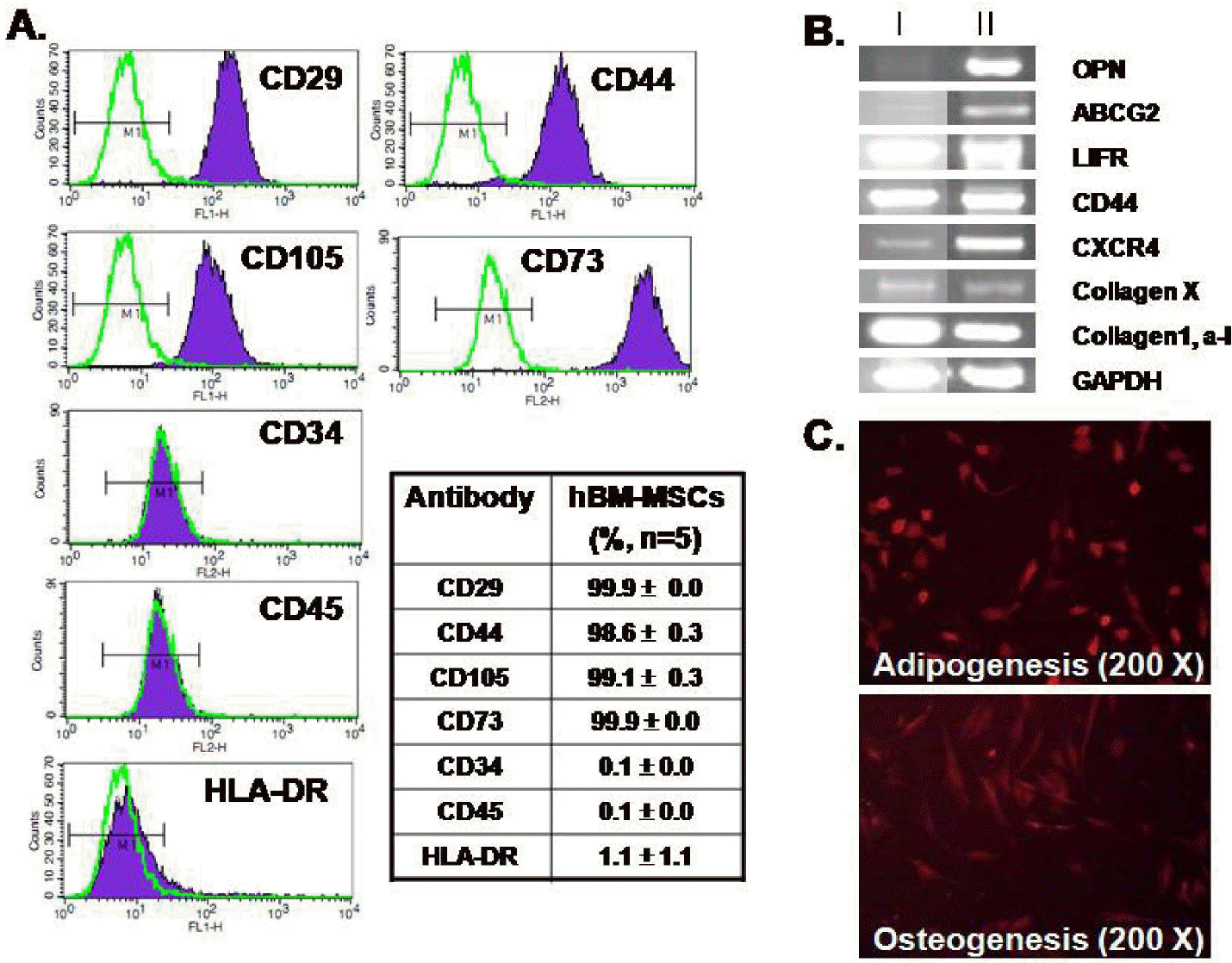
Fig. 2.
Different patterns of membrane currents recorded in hBM-MSCs. Current traces elicited by the voltage step (inset) in hBM-MSCs. (A) a slowly activating current similar to IKDR at potentials from +20 to +100 mV that coexisted with IKCa. (B) Ito (arrow) with IKCa. (C) INa,TTX (arrow) with IKDR and IKCa. (D) IKir (arrow) with IKDR and IKCa. (E) Current-voltage relationships were plotted from A, B, C and D.
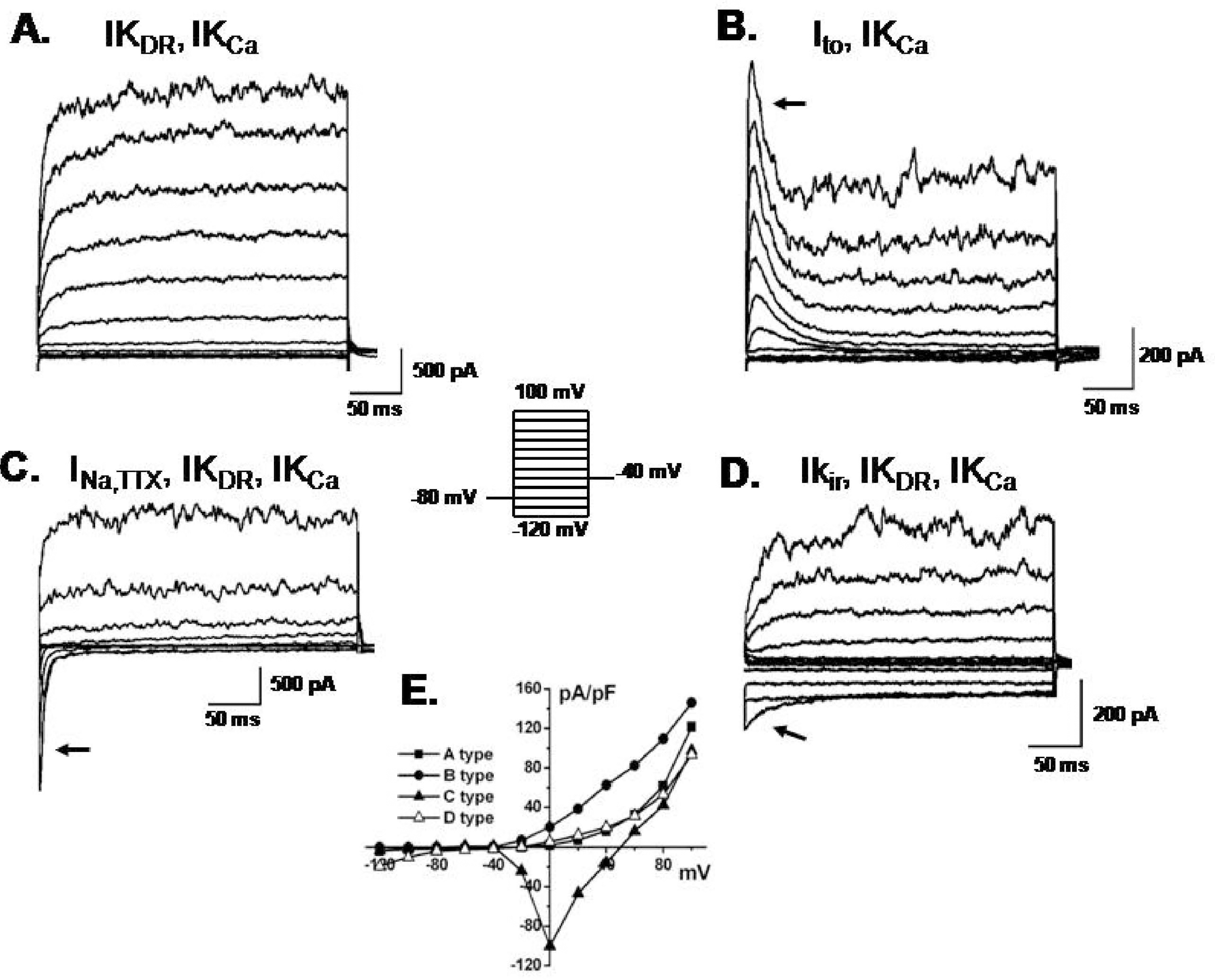
Fig. 3.
Pharmacological effects of functional ion channels in hBM-MSCs. (A) Membrane currents were recorded in the presence of 10 mM TEA or co-application of TEA and 300 μM 4-AP. (B) INa,TTX was continuously recorded under control conditions and in the presence of TTX. INa,TTX was blocked by TTX (left panel) and also blocked by verapamil (right panel). (C) The I-V relationships of Kir were obtained by ramp voltage induced currents against membrane potentials in bath solutions containing 5, 15, 30, 75, and 150 mM K+ as indicated (left panel). Reversal potentials from six patches were observed and plotted as a function of external [K+] concentrations (right panel). The dotted line represents the slope from the Nernst equation (slope, 58 mV/decade). Experimental values (O) were fitted by linear regression (slope, 66 mV/decade).
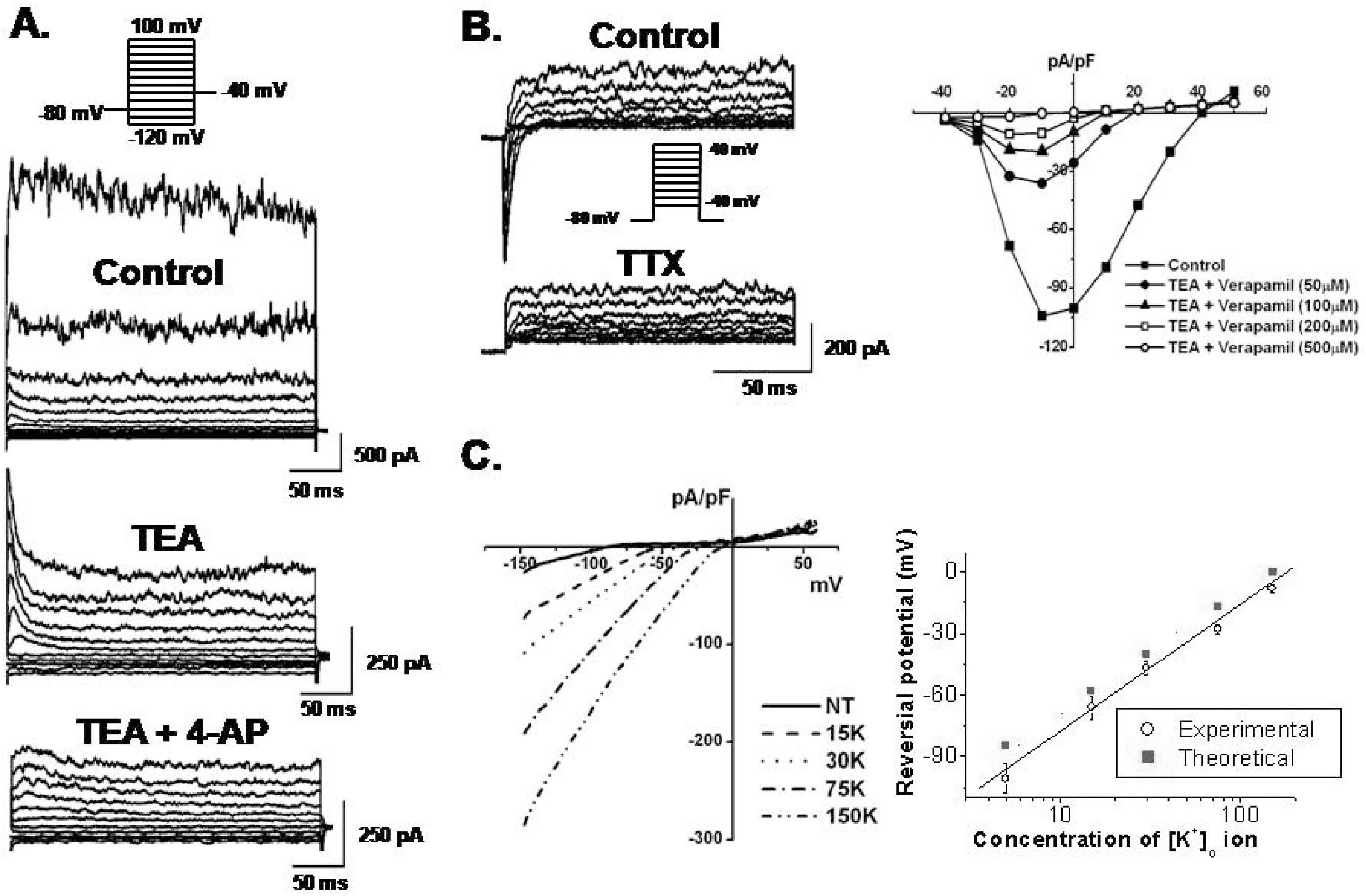
Fig. 4.
Comparison of mRNA expression of ion channels between passages. (A) Kv1.1, heag1 (for IKDR), Kv4.2 (for Ito), Kir2.1 (for Kir), MaxiK (for IKCa) and hNE-Na (for INa.TTX) were detected in hBM-MSCs, but not SCN5A (for TTX-resistant INa). β -actin was used as the control. (B) The “recording rate (%)” of INa,TTX in various passage of hBM-MSCs. (C) Relative passage-dependent mRNA quantities of the hNE-Na gene by quantitative RT-PCR (n=3). The inset in Fig. 4C shows a representative result for the hNE-Na gene from two different samples. (D) Relative passage-dependent MaxiK gene expression levels in hBM-MSCs. Inset in Fig. 4D displays representative passage-dependent MaxiK channel gene expression patterns from two different samples. ∗p<0.05, ∗∗p<0.005 when compared with each 3rd passage group.
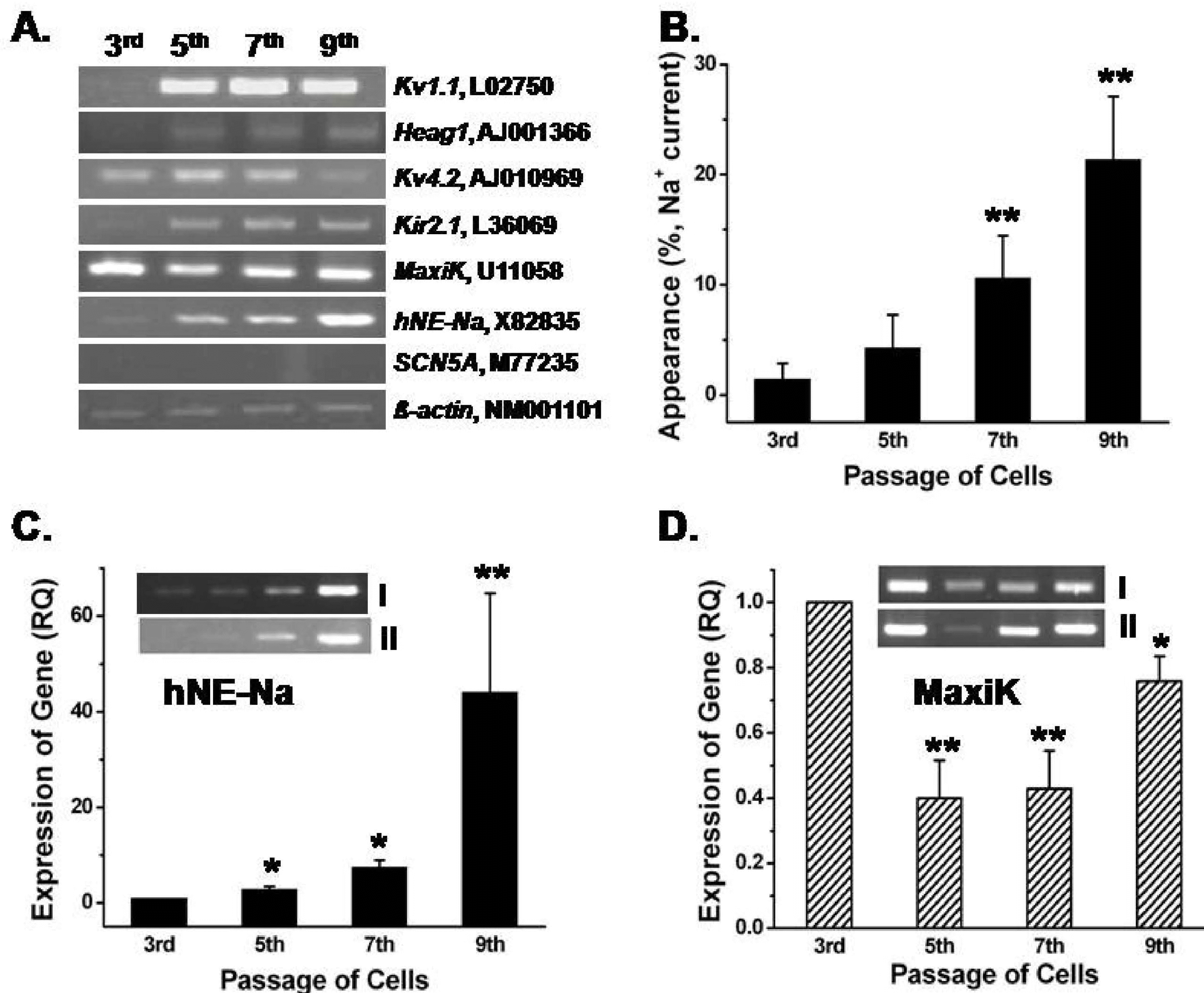
Table 1.
List of ion channel primers used for RT-PCR