Abstract
TREK (TWIK-RElated K+ channels) and TRAAK (TWIK-Related Arachidonic acid Activated K+ channels) were expressed in COS-7 cells, and the channel activities were recorded from inside-out membrane patches using holding potential of −40 mV in symmetrical 150 mM K+ solution. Intracellular application of an oxidizing agent, 5,5'-dithio-bis (2-nitrobenzoic acid) (DTNB), markedly decreased the activity of the TREK2, and the activity was partially reversed by the reducing agent, dithiothreitol (DTT). In order to examine the possibility that the target sites for the oxidizing agents might be located in the C-terminus of TREK2, two chimeras were constructed: TREK2 (1-383)/TASK3C and TREK2 (1-353)/TASK3C. The channel activity in the TREK2 (1-383)/TASK3C chimera was still inhibited by DTNB, but not in the TREK2 (1-353)/TASK3C chimera. These results indicate that TREK2 is inhibited by oxidation, and that the target site for oxidation is located between the amino acid residues 353 and 383 in the C-terminus of the TREK2 protein.
REFERENCES
Bang H., Kim Y., Kim D. TREK-2, a new member of the mecha-nosensitive tandem-pore K+ channel family. J Biol Chem. 275:17412–17419. 2000.
Buckler KJ., Honore E. The lipid-activated two-pore domain K+ channel TREK-1 is resistant to hypoxia: implication for ischaemic neuroprotection. J Physiol. 562(Pt 1):213–222. 2005.
Caley AJ., Gruss M., Franks NP. The effects of hypoxia on the modulation of human TREK-1 potassium channels. J Physiol. 562(Pt 1):205–212. 2005.


DiChiara TJ., Reinhart PH. Redox modulation of hslo Ca2+ -activated K+ channels. J Neurosci. 17:4942–4955. 1997.
Haarmann CS., Fink RH., Dulhunty AF. Oxidation and reduction of pig skeletal muscle ryanodine receptors. Biophys J. 77:3010–3022. 1999.


Honore E. The neuronal background K2P channels: focus on TREK1. Nat Rev Neurosci. 8:251–261. 2007.
Honore E., Maingret F., Lazdunski M., Patel AJ. An intracellular proton sensor commands lipid- and mechano-gating of the K+ channel TREK-1. Embo J. 21:2968–2976. 2002.
Kim D. Physiology and pharmacology of two-pore domain potassium channels. Curr Pharm Des. 11:2717–2736. 2005.


Kim Y., Bang H., Gnatenco C., Kim D. Synergistic interaction and the role of C-terminus in the activation of TRAAK K+ channels by pressure, free fatty acids and alkali. Pflugers Arch. 442:64–72. 2001a.
Kim Y., Gnatenco C., Bang H., Kim D. Localization of TREK-2 K+ channel domains that regulate channel kinetics and sensitivity to pressure, fatty acids and pHi. Pflugers Arch. 442:952–960. 2001b.
Kim Y., Lee SH., Ho WK. Hydrogen peroxide selectively increases TREK-2 currents via myosin light chain kinases. Front Biosci. 12:1642–1650. 2007.


Maingret F., Lauritzen I., Patel AJ., Heurteaux C., Reyes R., Lesage F., Lazdunski M., Honore E. TREK-1 is a heat-activated background K+ channel. Embo J. 19:2483–2491. 2000.
Maingret F., Patel AJ., Lesage F., Lazdunski M., Honore E. Mechanoor acid stimulation, two interactive modes of activation of the TREK-1 potassium channel. J Biol Chem. 274:26691–26696. 1999.
Miller P., Kemp PJ., Lewis A., Chapman CG., Meadows HJ., Peers C. Acute hypoxia occludes hTREK-1 modulation: re-evaluation of the potential role of tandem P domain K+ channels in central neuroprotection. J Physiol. 548(Pt 1):31–37. 2003.
Miller P., Kemp PJ., Peers C. Structural requirements for O2 sensing by the human tandem-P domain channel, hTREK1. Biochem Biophys Res Commun. 331:1253–1256. 2005.
Miller P., Peers C., Kemp PJ. Polymodal regulation of hTREK1 by pH, arachidonic acid, and hypoxia: physiological impact in acidosis and alkalosis. Am J Physiol Cell Physiol. 286:C272–282. 2004.


Patel AJ., Honore E., Maingret F., Lesage F., Fink M., Duprat F., Lazdunski M. A mammalian two pore domain mechano-gated S-like K+ channel. Embo J. 17:4283–4290. 1998.
Ruppersberg JP., Fakler B. Complexity of the regulation of Kir2.1 K+ channels. Neuropharmacology. 35:887–893. 1996.
Ruppersberg JP., Stocker M., Pongs O., Heinemann SH., Frank R., Koenen M. Regulation of fast inactivation of cloned mammalian IKA channels by cysteine oxidation. Nature. 352:711–714. 1991.


Sullivan JM., Traynelis SF., Chen HS., Escobar W., Heinemann SF., Lipton SA. Identification of two cysteine residues that are required for redox modulation of the NMDA subtype of glutamate receptor. Neuron. 13:929–936. 1994.


Tang XD., Daggett H., Hanner M., Garcia ML., McManus OB., Brot N., Weissbach H., Heinemann SH., Hoshi T. Oxidative regulation of large conductance calcium-activated potassium channels. J Gen Physiol. 117:253–274. 2001.


Zeidner G., Sadja R., Reuveny E. Redox-dependent gating of G protein-coupled inwardly rectifying K+ channels. J Biol Chem. 276:35564–35570. 2001.
Fig. 1.
The effects of oxidizing chemical agent, DTNB, on TRAAK, TREK1, and TREK2 in inside-out patch configurations. (A) The left panel shows the general single channel activity without DTNB, and the right panel shows channel activity with DTNB (2 mM). DTNB was applied to the bath solution, and the holding potential was held at −40 mV. “Close” represents the closed-channel level, and “open” represents the open-channel level. (B) The relative channel activity with DTNB on each channel. (C) Dose response curve of DTNB on TREK2. The pipette and bath solution contained 150 mM KCl, 5 mM EGTA, 10 mM HEPES, and 1 mM MgCl2. The asterisk indicates a significant difference from the respective control (p<0.05).

Fig. 2.
The effect of extracellular application of DTNB (2 mM) and DTT (5 mM) on TREK2 using outside-out patch configurations. The holding potential was held at −40 mV. (A) The channel activity was not changed by DTNB and DTT. (B) Expanded scale trace of channel activity from A.

Fig. 3.
The intracellular C-terminus of TREK2 modulates DTNB effects upon channel activity. (A) The first and second panels show the effect of DTNB (2 mM) on wild type TREK2 (1-538) and TREK2 chimera [TREK2 (1383)/TASK3C], respectively. The third panel shows the effect of DTNB on TREK2 chimera [TREK2 (1-353)/TASK3C]. The fourth panel shows the effect of DTNB on mutant TREK2 (1-383, M375L)/TASK3C (methionine-to-leucine substitution at position 375). (B) Expanded scale trace of channel activity from the A.
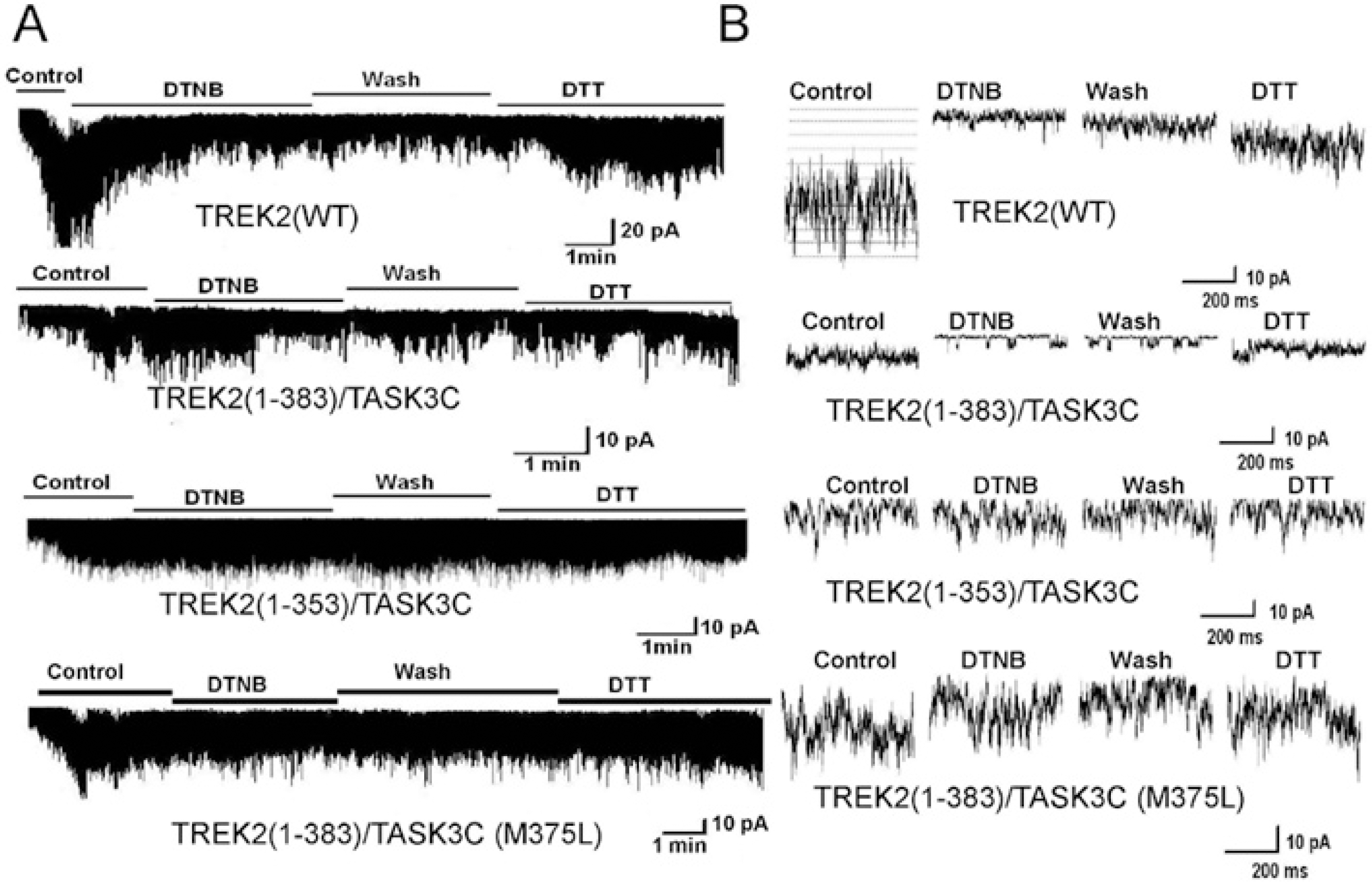
Fig. 4.
The effect of intracellular acidic pH on wild type TREK2 and a chimera [TREK2 (1-353)/TASK3C] in the presence of DTNB. (A) An intracellular acidic pH activated wild type TREK2 in the absence of DTNB, but intracellular acidic pH did not activate wild type TREK2 in the presence of DTNB. (B) An intracellular acidic pH activated the chimera [TREK2 (1-353)/TASK3C] regardless of the presence of DTNB.
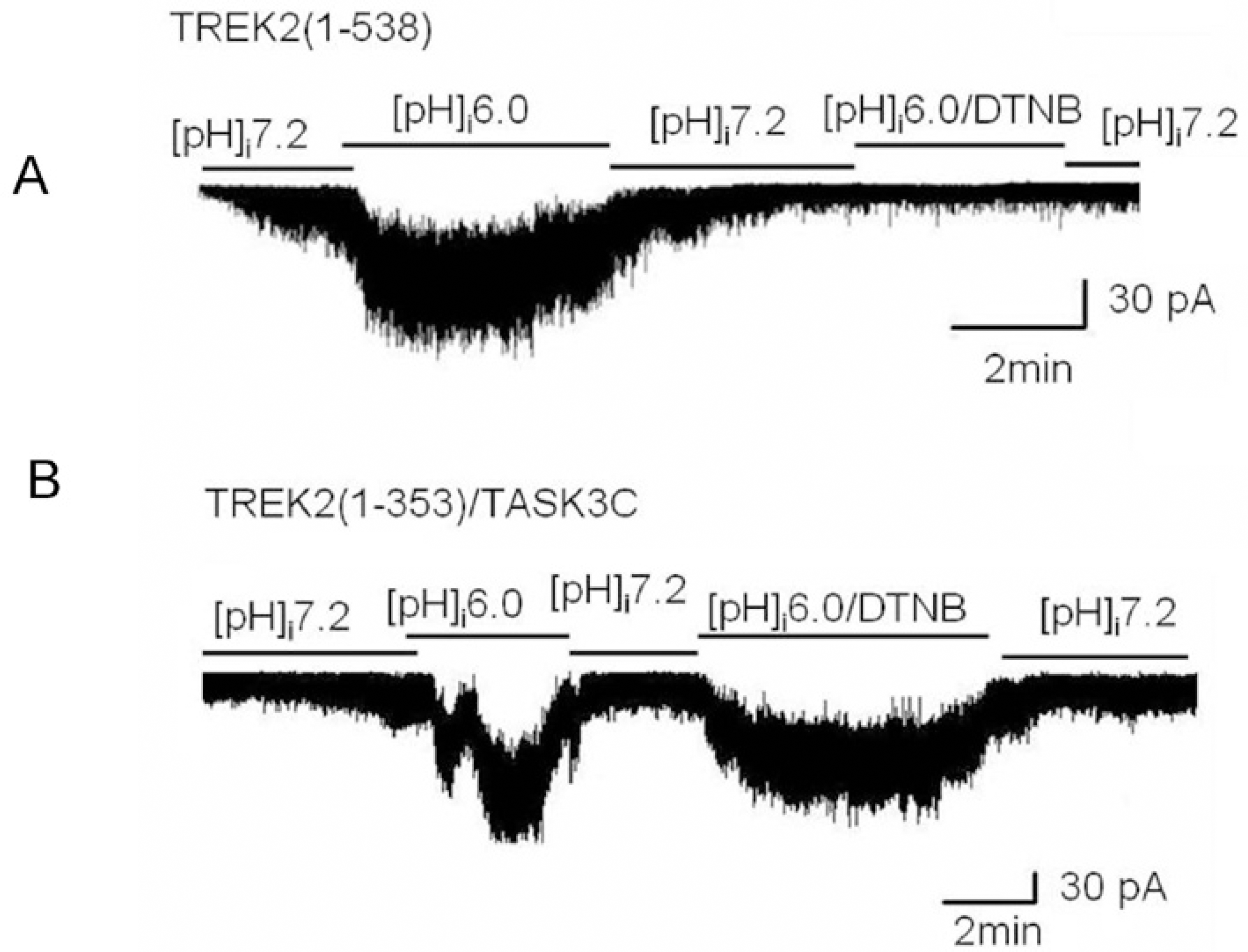
Fig. 5.
Summary of the effects of DTNB on wild type TREK2 and mutants. (A) The amino acid sequence of the C-terminus of TREK2. (B) Membrane topologies of TREK2 and mutants show two pore-forming domains and four transmembrane segments (The membrane topology has been modified from Kim et al, 2001b). The amino acid positions indicate where the C-terminal replacements were made. The portion of the TREK2 C-terminus that was replaced with the C-terminus of TASK3. (C) The bar graph shows a summary of the effects of DTNB on wild type TREK2 and mutants.
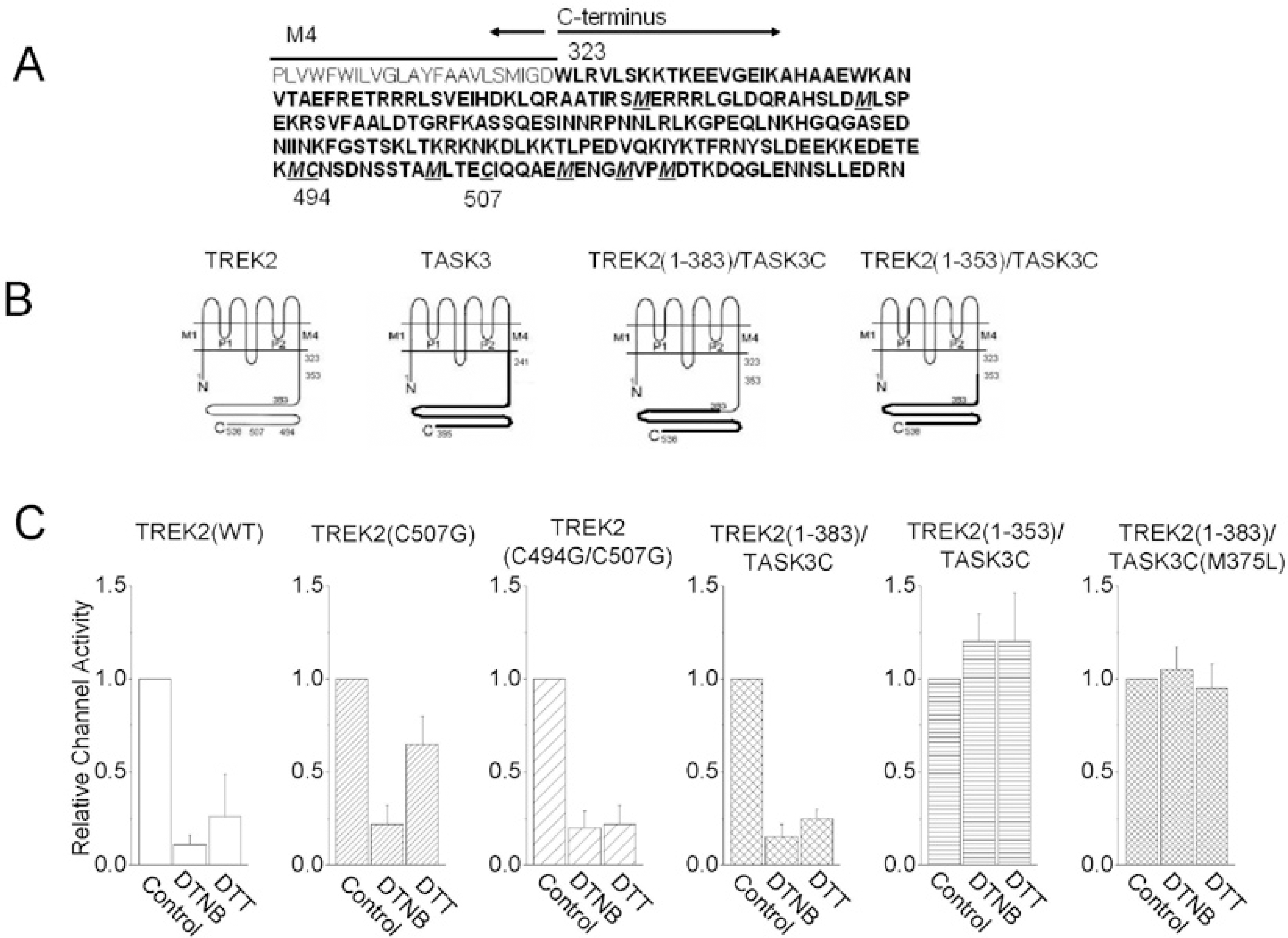