Abstract
Purpose
To determine deep optic nerve head structure changes after transient intraocular pressure elevation during laser in situ keratomileusis (LASIK) for myopia.
Methods
Enhanced depth imaging-optical coherence tomography was performed in each myopic eye that underwent LASIK surgery. Enhanced depth imaging-optical coherence tomography images were created at postoperative 1 day, 1 week, 2 weeks, and 1 month. Lamina cribrosa (LC) thickness, LC depth and prelaminar thickness at the superior, middle and inferior portions of the optic nerve head were measured by two investigators.
Results
Forty eyes in 40 patients were included in the present study. During follow-up, there were no significant differences in prelaminar thickness or LC depth. The LC demonstrated increased thickness at postoperative 1 day at all three locations (superior, middle, and inferior) (p < 0.001, p < 0.001, p < 0.001, respectively). However, no significant changes were observed at postoperative 1 week, 2 weeks, and 1 month.
Laser in situ keratomileusis (LASIK) is a commonly preferred refractive surgery to correct myopia [1]. However, myopia is a well-known risk factor of glaucoma. High intraocular pressure (IOP) can occur due to pneumatic suction during LASIK surgery, and additional safety issues of LASIK in myopic patients have been addressed previously [23].
The lamina cribrosa (LC), an array of collagen beams with intervening pores, is the primary site of glaucomatous damage [4]. When IOP is increased, pores in the LC might undergo conformational changes due to IOP-related mechanical strain, eventually causing axonal transport blockage [5]. Therefore, it is important to investigate structural changes in LC to identify whether high IOP during LASIK surgery might induce early glaucomatous changes in myopic eyes. However, changes in deep structures after LASIK surgery have not yet been reported.
Therefore, the objective of this study was to determine changes in deep structures of the optic nerve head after LASIK surgery using enhanced depth imaging spectral-domain (SD) optical coherence tomography (OCT). Results of this study could improve understanding of the impact of high IOP during LASIK surgery on deep structures in the optic nerve head (ONH).
A total of 52 eyes of 52 participants were initially investigated in this prospective observational study. These participants underwent LASIK surgeries performed by a single surgeon (TYC) between July 2013 and September 2013. This study followed all guidelines for experimental investigation in human subjects and was approved by Samsung Medical Center institutional review board (2013-06-061). This study adhered to the tenets of the Declaration of Helsinki and was registered at ClinicalTrials.gov (NCT02676557). Informed consent was obtained from all participants. Inclusion criterion for this study was eyes undergoing LASIK surgery for myopia. Eyes were excluded if images were difficult to investigate, including an unclear LC margin, severe shadowing due to overlying vessels, or poor image quality due to cataracts. Participants underwent a comprehensive ophthalmic assessment, including slit-lamp biomicroscopy, Goldmann applanation tonometry, manifested refraction, dilated fundus photography, and enhanced depth imaging (EDI) SD-OCT (Spectralis OCT; Heidelberg Engineering, Heidelberg, Germany). Eyes were excluded if there had previous ocular disease, including glaucoma. Transient IOP elevation was induced between 15 and 20 seconds in the eye of each subject by a modified LASIK automated corneal shaper (Mel80; Zeiss Meditec, Dublin, CA, USA) after a local anesthetic was administered. Because a LASIK suction ring could potentially produce IOP in excess of 90 mmHg, the rigid plastic tubing connecting the suction ring to the main pump was punctured by two 18-gauge needles that were left in situ to reduce potential intraluminal negative pressure and limit possible peak IOP elevation [6].
The Heidelberg Spectralis OCT EDI mode was used for all images and was set to image a 10° × 15° rectangle for horizontal scans centered on the optic disc. This rectangle was scanned with 65 sections. Each section had 43 average OCT frames based on a previous study [7]. The OCT machine scaling was adjusted to 1 : 1 µm before measurement. The magnification error was corrected using a formula provided by the manufacturer, based on the auto refraction keratometry results and the focus setting during image acquisition. The Heidelberg Spectralis program includes an EDI mode, that automatically places the OCT reference plane toward the bottom of the OCT acquisition screen. The zero delay is placed at the bottom of the OCT screen, which enhances images from the deeper layers of the ONH.
Prelaminar (PL) thickness was defined as the shortest distance between the anterior border of the PL tissue and the anterior border of the LC. LC thickness was defined as the shortest distance between the anterior border and the posterior border of the LC. The anterior and posterior LC margins were defined by a highly reflective structure below the optic cup. Anterior and posterior LC margins were defined as the line that connected the peripheral points of the anterior and posterior LC margins. The line that connected the two termination points of Bruch's membrane was used as a baseline reference. The LC depth was measured by calculating the average at each periphery of the anterior LC margin. When the shadow of the vessel trunk restricted observation of the peripheral LC margin in the nasal area, the observed LC margin was used to measure the peripheral point of the LC margin. The distance from the reference line to the level of the anterior border of the LC was measured perpendicular to the reference line. Therefore, the average value of each peripheral LC depth was used as the LC depth (Fig. 1A). The same methods were used to measure other parameters, such as the LC thickness, LC depth and PL thickness in the eyes with curved or irregular LC margins.
PL thickness, LC thickness, and LC depth were measured in central, superior, and inferior mid-periphery ONH. Three B-scans in each portion were measured, and the average LC thickness and depth of nine images were used for analysis (Fig. 1B). All images were analyzed and measured twice by two examiners (SML and DYC) who independently determined LC thickness, LC depth, and PL thickness.
Descriptive statistics including the number and percentage of each categorical variable and mean ± standard deviation of each continuous variable were evaluated to show demographic and ocular parameters. The PL thickness, LC thickness, and depth values were compared to baseline values at each time point: postoperative 1 day, 1 week, 2 weeks, and 1 month. Repeated ANOVA test was performed at each time point to examine differences before and after surgery. Univariate and multivariate logistic regression analyses were performed to identify factors associated with LC thickening after LASIK surgery. Independent variables were age, spherical equivalent (SE), central corneal thickness (CCT), IOP and baseline average LC thickness. Parameters with a p-value less than 0.3 in univariate analysis were included in multivariate logistic analysis. The IBM SPSS ver. 23.0 (IBM Corp., Armonk, NY, USA) was used for all statistical analyses of this study. A p-values less than 0.05 was considered statistically significant.
Forty eyes in 40 participants were included in this study. Thirteen of the participants were male (32.5%), and the average age of all participants was 28 years (range, 19 to 51 years). The average CCT was 556 µm (range, 506 to 627 µm), and the average SE was −6 diopter (range, −10 to −3 diopter). The average IOP was 17 mmHg (range, 10 to 23 mmHg) (Table 1).
During follow-up, there was no significant difference in PL thickness or LC depth compared to those at preoperative exams. LC thickness values at the superior, middle and inferior portions were significantly increased at postoperative 1 day (all p < 0.001) (Fig. 2A–2C). However, no significant changes were observed at postoperative 1 week, 2 weeks, or 1 month compared to baseline LC thickness (Table 2).
The LC thickness change at postoperative 1 day was significant in this present study. Therefore, the factors associated with the presence of significant LC thickening at postoperative 1 day after LASIK surgery were examined. Significant LC thickening was defined as an increase over 10 µm, the standard deviation of baseline LC thickness in this study. In univariate logistic regression analysis, age, SE, CCT, IOP, and baseline average LC thickness were not associated with LC thickening at 1 day after LASIK surgery (p = 0.674, p = 0.868, p = 0.244, p = 0.475, p = 0.075, respectively). In multivariate logistic regression analysis, CCT and baseline average LC thickness were not associated with LC thickening at 1 day after LASIK surgery (p = 0.256, p = 0.064) (Table 3).
The results of this study demonstrate that LC thickness can increase after baseline at 1 day after LASIK surgery. However, there were no irreversible changes in deep structures such as PL tissue or LC (Fig. 3). However, just because there are no changes in the LC structure, does not confirm that there is no glaucomatous damage after LASIK. This is because ischemic damages without retinal ganglion cell apoptosis can also induce damages on retinal nerve fiber layer (RNFL) [8]. However, our results showed no change in LC, supporting the hypothesis that LASIK might not induce irreversible changes in LC in healthy myopic eyes. Although there could be damages to the RNFL due to ischemic and mechanical damages after LASIK surgery, permanent changes in LC structures, such as LC thickness, LC depth, and PL thickness, may not occur after LASIK surgery. To the best of our knowledge, this is the first report that visualizes structural changes in optic nerve tissues after LASIK surgery.
A pneumatic suction ring is usually used for a few seconds during LASIK surgery. While applying suction, the IOP should be increased to greater than 65 mmHg to create a lamellar flap [9]. This type of increase in IOP during suction can cause damages to ocular structures, such as retinal vessels, retinal nerve fibers, and the LC. Retinal complications following LASIK have been previously reported [101112]. Maden et al. [10] have reported non-arteritic ischemic optic neuropathy after LASIK with femtosecond laser flap creation. Smith et al. [11] have shown hemi-retinal vein occlusion following LASIK. Lee et al. [12] have reported four cases of optic neuropathy following LASIK. Glaucomatous changes after LASIK surgery have also been suggested [131415]. However, most studies showed that there were no changes in RNFL after LASIK surgery [161718]. Previously, Kim et al. [19] have investigated eyes with or without refractive surgery and found no differences in glaucoma progression between the two groups. Because LC is related to the pathogenesis of glaucoma, the results of the present study support the results of previous studies.
Changes in LC have been reported in previous studies of eyes with glaucoma. A histologic study by Jonas et al. [20] suggested that LCs in eyes with glaucoma are thinner than in normal controls. This suggestion was supported by an in vivo study that used EDI-OCT. Park et al. [21] have shown that normal tension glaucoma (NTG) has thinner LC than normal controls. Kwun et al. [22] have reported that NTG eyes have thinner LC than contralateral normal eyes in monocular NTG patients, while LC is thinner at the location of visual field defect. Kim et al. [19] have shown that open-angle glaucoma has deeper peripheral LC depth than normal controls. In addition, they suggested that this provides evidence of remodeling at the peripheral region of LC. In this study, we did not identify permanent changes in LC after LASIK surgery. This means that LASIK might not induce irreversible structural changes associated with glaucoma in LC.
The reason why high IOP could cause LC thickening has not previously been explained. In non-human experiments, LC that has been exposed to high IOP for several weeks is thicker than baseline LC [2324]. The authors of previous studies have suggested that this might be due to LC remodeling associated with high IOP. However, temporary thicker LC might not be associated with LC remodeling because the exposure time is relatively short (approximately 1 minute). Instead, transient changes in LC thickness might be due to compensatory blood flow after temporary ischemia in deep ONH [25]. Previously, Ozdamar and Ocakoglu [25] have suggested that LASIK could cause temporary increases in blood flow at the LC region in healthy myopic eyes. They proposed that a compensatory mechanism for maintaining blood f low after LASIK-induced ischemia in the LC could count for the increase of blood flow following LASIK. If LC temporary thickening is related to an increased blood flow associated compensatory mechanism, it provides a clinical hypothesis for the mechanism for high IOP to influence deep ONH circulation, including LC. This supports the hypothesis that high IOP can induce temporary ischemia on deep ONH and offers reasons for ischemic complications after LASIK. However, in our study, only LC thickness increased, but no changes were found in PL thickness. Because vascular affluence in peripapillary choroidal vessels may have greater effects on the prelaminar tissue than LC, it is currently difficult to fully explain this result [26]. Thus, additional studies are needed to determine why only LC thickness changes, but no changes in PL thickness were found in the present study.
There were several limitations to our study. First, current findings have not yet been corroborated by histologic evaluations. In vivo imaging offers a non-invasive technique to investigate LC changes; however, this modality cannot specify reasons for associated histologic changes in LC. Second, the present study was conducted only in young healthy subjects. Considering that LC stiffness can differ according to age, our study results should be interpreted with consideration of the specific age demographics. Third, the amount and duration of IOP increase were not measured in the present study. Therefore, different amounts and durations of IOP increase might have affected the results. Fourth, EDI-OCT images obtained at postoperative 1 day are prone to artifacts due to tearing or subtle corneal haze, which can interfere and cause inaccurate evaluation of the optic nerve structures. Images were taken again when they were deemed insufficient for evaluation. Fifth, evaluation of the LC structure in the myopic tilted optic disc was not accurate. However, because we measured the same position during observation periods in the same eye, we could compare the differences of LC structures even in eyes with disc tilt. In addition, the follow-up period was relatively short in this study, and changes in LC were observed for 1 month. However, LC is a dynamic and changeable structure. Lee et al. [27] have examined the responses of LC and PL thickness to glaucoma surgery. Their study showed a significant reduction in posterior displacement of the LC with increases in PL thickness and LC thickness. Since glaucoma is considered a long-term disease, it is currently unclear whether structural changes in LC would occur after long term follow-up.
In conclusion, LC thickness could increase at 1 day after LASIK surgery. However, the thickness will eventually return to the baseline morphology. Therefore, these changes in LC thickness could be attributable to compensatory blood flow after temporary ischemia on LC.
References
1. Yuen LH, Chan WK, Koh J, et al. A 10-year prospective audit of LASIK outcomes for myopia in 37,932 eyes at a single institution in Asia. Ophthalmology. 2010; 117:1236–1244. PMID: 20153899.


2. Chan KC, Poostchi A, Wong T, et al. Visual field changes after transient elevation of intraocular pressure in eyes with and without glaucoma. Ophthalmology. 2008; 115:667–672. PMID: 17716733.


3. Lleo-Perez A, Sanchis Gimeno J. Changes in the visual field following laser in situ keratomileusis for myopia. Ophthalmic Physiol Opt. 2007; 27:201–209. PMID: 17324211.
4. Quigley HA, Addicks EM, Green WR, Maumenee AE. Optic nerve damage in human glaucoma. II. The site of injury and susceptibility to damage. Arch Ophthalmol. 1981; 99:635–649. PMID: 6164357.
5. Minckler DS, Bunt AH, Johanson GW. Orthograde and retrograde axoplasmic transport during acute ocular hypertension in the monkey. Invest Ophthalmol Vis Sci. 1977; 16:426–441. PMID: 67096.
6. Kasetsuwan N, Pangilinan RT, Moreira LL, et al. Real time intraocular pressure and lamellar corneal flap thickness in keratomileusis. Cornea. 2001; 20:41–44. PMID: 11189002.


7. Han JC, Choi DY, Kwun YK, et al. Evaluation of lamina cribrosa thickness and depth in ocular hypertension. Jpn J Ophthalmol. 2016; 60:14–19. PMID: 26361960.


8. Bernstein SL, Johnson MA, Miller NR. Nonarteritic anterior ischemic optic neuropathy (NAION) and its experimental models. Prog Retin Eye Res. 2011; 30:167–187. PMID: 21376134.


9. Cameron BD, Saffra NA, Strominger MB. Laser in situ keratomileusis-induced optic neuropathy. Ophthalmology. 2001; 108:660–665. PMID: 11297479.


10. Maden A, Yilmaz S, Yurdakul NS. Nonarteritic ischemic optic neuropathy after LASIK with femtosecond laser flap creation. J Neuroophthalmol. 2008; 28:242–243. PMID: 18769298.


11. Smith BT, Park CH, Fekrat S. Hemi-retinal vein occlusion following LASIK. Ann Ophthalmol (Skokie). 2006; 38:139–140. PMID: 17416945.


12. Lee AG, Kohnen T, Ebner R, et al. Optic neuropathy associated with laser in situ keratomileusis. J Cataract Refract Surg. 2000; 26:1581–1584. PMID: 11084263.


13. Shaikh NM, Shaikh S, Singh K, Manche E. Progression to end-stage glaucoma after laser in situ keratomileusis. J Cataract Refract Surg. 2002; 28:356–359. PMID: 11821221.


14. Bushley DM, Parmley VC, Paglen P. Visual field defect associated with laser in situ keratomileusis. Am J Ophthalmol. 2000; 129:668–671. PMID: 10844064.


15. Weiss HS, Rubinfeld RS, Anderschat JF. Case reports and small case series: LASIK-associated visual field loss in a glaucoma suspect. Arch Ophthalmol. 2001; 119:774–775. PMID: 11346411.
16. Whitson JT, McCulley JP, Cavanagh HD, et al. Effect of laser in situ keratomileusis on optic nerve head topography and retinal nerve fiber layer thickness. J Cataract Refract Surg. 2003; 29:2302–2305. PMID: 14709290.


17. Hamada N, Kaiya T, Oshika T, et al. Optic disc and retinal nerve fiber layer analysis with scanning laser tomography after LASIK. J Refract Surg. 2006; 22:372–375. PMID: 16629070.


18. Zangwill LM, Abunto T, Bowd C, et al. Scanning laser polarimetry retinal nerve fiber layer thickness measurements after LASIK. Ophthalmology. 2005; 112:200–207. PMID: 15691551.


19. Kim YJ, Yun SC, Na JH, et al. Glaucoma progression in eyes with a history of refractive corneal surgery. Invest Ophthalmol Vis Sci. 2012; 53:4485–4489. PMID: 22695960.


20. Jonas JB, Jonas SB, Jonas RA, et al. Histology of the parapapillary region in high myopia. Am J Ophthalmol. 2011; 152:1021–1029. PMID: 21821229.


21. Park HY, Jeon SH, Park CK. Enhanced depth imaging detects lamina cribrosa thickness differences in normal tension glaucoma and primary open-angle glaucoma. Ophthalmology. 2012; 119:10–20. PMID: 22015382.


22. Kwun Y, Han JC, Kee C. Comparison of lamina cribrosa thickness in normal tension glaucoma patients with unilateral visual field defect. Am J Ophthalmol. 2015; 159:512–518. PMID: 25498357.


23. Roberts MD, Grau V, Grimm J, et al. Remodeling of the connective tissue microarchitecture of the lamina cribrosa in early experimental glaucoma. Invest Ophthalmol Vis Sci. 2009; 50:681–690. PMID: 18806292.


24. Yang H, Thompson H, Roberts MD, et al. Deformation of the early glaucomatous monkey optic nerve head connective tissue after acute IOP elevation in 3-D histomorphometric reconstructions. Invest Ophthalmol Vis Sci. 2011; 52:345–363. PMID: 20702834.


25. Ozdamar A, Ocakoglu O. Optic nerve head blood flow using scanning laser Doppler flowmetry after laser in situ keratomileusis. J Refract Surg. 2003; 19:433–437. PMID: 12899474.


26. Hayreh SS. Blood supply of the optic nerve head and its role in optic atrophy, glaucoma, and oedema of the optic disc. Br J Ophthalmol. 1969; 53:721–748. PMID: 4982590.


27. Lee EJ, Kim TW, Weinreb RN. Reversal of lamina cribrosa displacement and thickness after trabeculectomy in glaucoma. Ophthalmology. 2012; 119:1359–1366. PMID: 22464141.


Fig. 1
Delineation of the border of the lamina cribrosa (LC). (A) Prelaminar (PL) thickness was defined as the distance between the anterior border of PL tissue and the anterior LC border (green line arrow). LC thickness was defined as the distance between the anterior border and the posterior border of the LC (red line arrow). LC depth was defined as the average distances (two dotted red line arrows) between the peripheral points of the anterior LC margins and the reference line connecting both the Bruch's membrane openings (white line). When the shadow of the vessel trunk restricts observation of the peripheral LC margin in the nasal area, the observed LC margin was used to measure the peripheral point of the LC margin. (B) LC thickness and depth were measured at the presumed vertical center of three areas (superior, center, and inferior).
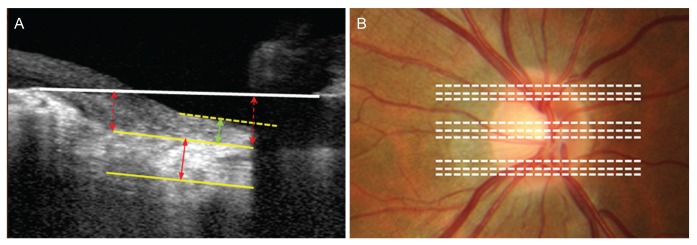
Fig. 2
Lamina cribrosa thickness (µm) changes. Lamina cribrosa thickness at postoperative day 1 was significantly increased compared to that at baseline. However, this value was not significantly different compared with other time points. (A) Superior, (B) middle, and (C) inferior.
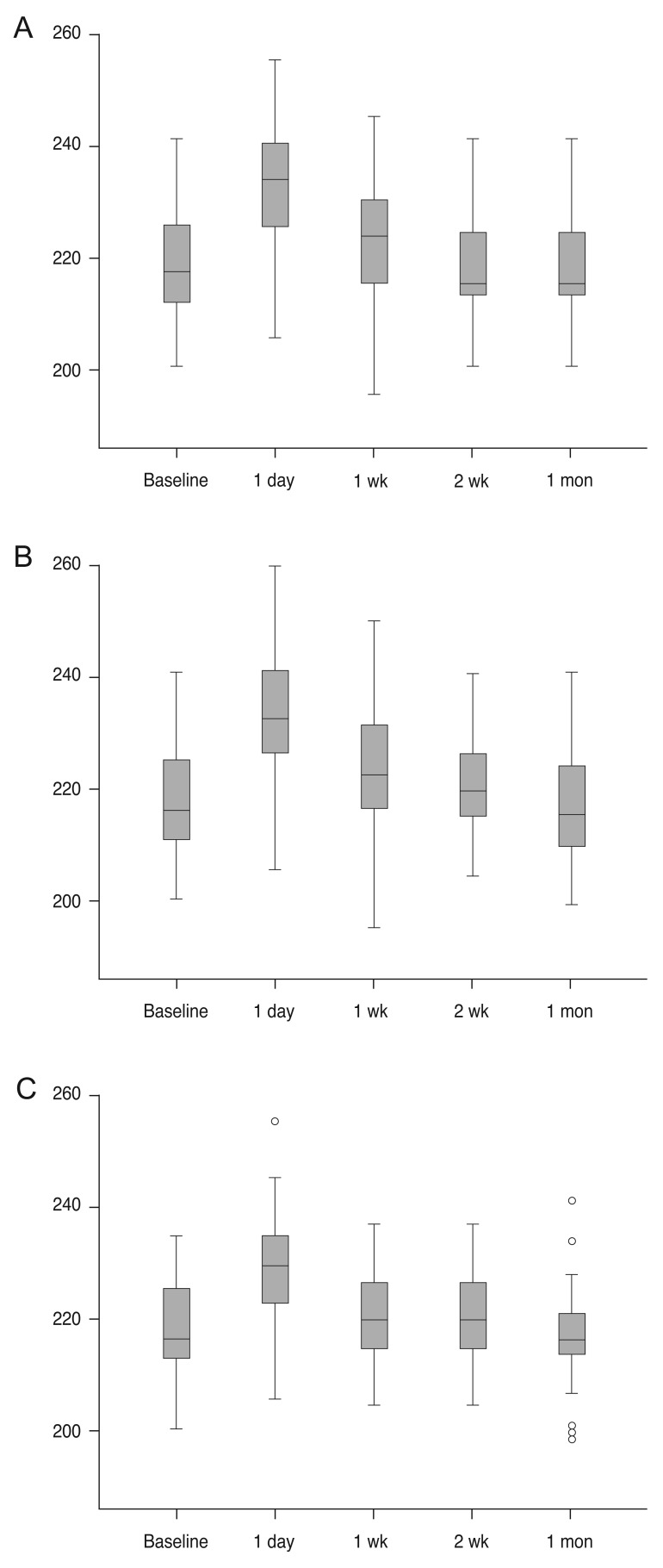
Fig. 3
A representative case. Enhanced depth imaging-optical coherence tomography images of the optic nerve head before and after laser in situ keratomileusis surgery of a 24-year-old female with −4.5 diopters of spherical equivalent. When the images were compared before and after surgery, lamina cribrosa (LC) thickness slightly increased (A) from 125 µm (B) to 173 µm at postoperative 1 day and returned to baseline LC thickness at postoperative (C) 1 week and (D) 1 month. Other parameters such as LC depth or prelaminar (PL) thickness were consistent, based on the results.
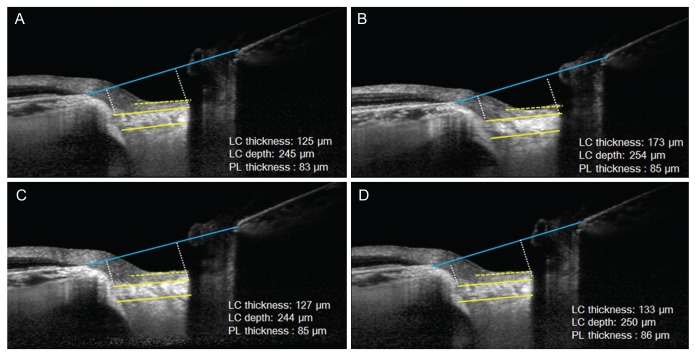