Abstract
Purpose
To evaluate the efficacy of anterior segment swept-source optical coherence tomography (SS-OCT) for examining horizontal extraocular muscle thickness, distance from the corneal limbus to the insertion of the horizontal extraocular muscle (limbus to insertion distance), and scleral thickness in Korean pediatric strabismus patients.
Methods
This study included pediatric strabismus patients between 5 and 10 years of age. Children with any ocular disease other than strabismus or a history of ocular surgery were excluded. SS-OCT was used to measure horizontal extraocular muscle thickness, limbus to insertion distance, and scleral thickness. Eyes were classified into subgroups by sex, spherical equivalent of the refractive error (measured with cycloplegic refraction), and fixating/deviating eye.
Results
One patient initially included in this study was excluded due to poor cooperation. Of the remaining 35 eyes of 20 patients, 19 eyes (54.3%) were from male patients and 16 eyes (45.7%) were from female patients. The mean patient age was 7.86 ± 1.38 years. Lateral scleral thickness was greater in male eyes than in female eyes (p = 0.048). No other differences were noted between male and female children. Additionally, there were no statistically significant differences between fixating and deviating eyes or among spherical equivalent groups for any parameter examined.
The primary goal of strabismus surgery is to alter muscle tension and the force vector by manipulating an extraocular muscle or by changing its insertion point. Therefore, it is important to clearly know extraocular muscle anatomy, especially for complicated strabismus surgery, strabismus reoperation, and unknown extraocular muscle surgery [1]. Unfortunately, it is not currently possible to accurately assess extraocular muscle structures due to the limitations of computed tomography (CT), magnetic resonance imaging (MRI), B-scan ultrasonography, and ultrasound biomicroscopy [2345]. However, optical coherence tomography (OCT) has made it possible to conveniently image the anterior segment, including the extraocular muscles [6789]. Commercial swept-source OCT (SS-OCT) systems are able to produce more accurate anterior segment images compared to time-domain or spectral-domain OCT [10].
This study evaluated the usefulness of anterior segment SS-OCT in measuring horizontal extraocular muscle thickness, distance from the corneal limbus to the insertion of horizontal extraocular muscle (limbus to insertion distance, LTID) and scleral thickness in Korean pediatric strabismus patients.
This retrospective study protocol was reviewed and given exempt status by the institutional review board (2017-02-010-001) at Keimyung University Dongsan Medical Center. Therefore, the requirement for informed consent was waived. All study conduct adhered to the tenets of the Declaration of Helsinki.
This study included children who had been diagnosed with strabismus and who were between the ages of 5 and 10 years. All children had undergone external ocular photography and anterior segment SS-OCT imaging in both eyes. Patients were excluded from analysis if they had a history of ocular disease except strabismus or of ocular surgery (including strabismus surgery). Patient with poor quality images that made it impossible to perform study analyses were also excluded.
All patients had a complete medical history taken and underwent thorough ophthalmic examination. Subjects underwent SS-OCT imaging (DRI OCT Triton plus; Topcon, Tokyo, Japan) performed by a single examiner. An external fixation light that was attached to the device was used to allow the extraocular muscles to be imaged. To image the lateral rectus muscle, the fixation light was moved to the medial side. To image the medial rectus muscle, the fixation light was moved to the lateral side. Horizontal extraocular muscle thickness, LTID and scleral thickness were measured on the lateral and medial sides. For study analyses, the location of the corneal limbus was defined as the intersection between a line of descent perpendicular to a posterior corneal surface tangent on the scleral spur and the corneal-scleral transition point. The extraocular muscle insertion site was defined as the end of the gap between the extraocular muscle and the sclera. Extraocular muscle thickness was measured at the boundary between the extraocular muscle and the corresponding tendon. To achieve consistency with respect to the point of measurement, lateral scleral thickness was measured at 1,500 µm temporal to the insertion site of the lateral rectus muscle and medial scleral thickness was measured at 1,500 µm nasal from the insertion site of the medial rectus muscle. Measurements were made by a single pediatric ophthalmologist using built-in digital calipers (Fig. 1A, 1B). The spherical equivalent (SE) of refractive error was calculated by a cycloplegic refraction using 1% cyclopentolate (Cyclogyl; Alcon, Fort Worth, TX, USA), and the fixating eye was determined using the alternate prism cover test. Subjects were then subdivided according to refractive error as follows: SE <−3.00 diopters (D) (group 1), −3.00 D ≤ SE ≤+3.00 D (group 2), and SE >+3.00 D (group 3). Mann-Whitney and Kruskal-Wallis tests were used to analyze correlations between ocular biometric data, fixating/deviating eye, and patient sex. All statistical analyses were performed using IBM SPSS ver. 22.0 (IBM Corp., Armonk, NY, USA) and statistical significance was defined as p < 0.05.
A total of 44 eyes of 22 children (13 male and 9 female) between the ages of 5 and 10 years of age were considered for inclusion. Five eyes of 5 patients were excluded due to unilateral strabismus surgery, 2 eyes of 1 patient were excluded due to bilateral strabismus surgery, and 2 eyes of 1 patient were excluded due to poor cooperation. Thus, data from 35 eyes of 20 patients (12 male and 8 female) were included in this study. Nineteen eyes (54.3%) were of male children and 16 eyes (45.7%) were of female children. The mean age of this patient group was 7.86 ± 1.38 years, and the mean SE was 0.14 ± 3.45 D.
Measurements with SS-OCT revealed that mean lateral scleral thickness, lateral rectus muscle thickness, medial scleral thickness, and medial rectus muscle thickness were 460.51 ± 48.07, 148.43 ± 34.91, 458.77 ± 52.77, and 151.17 ± 80.50 µm, respectively. LTID were 5198.94 ± 819.47 and 5012.60 ± 814.14 µm on the lateral side and the medial side, respectively. Lateral scleral thickness was significantly greater in male patients (474.21 ± 48.30 µm) than in female patients (444.25 ± 43.79 µm, p = 0.048), but no other significant differences between male and female patients were identified (Table 1). No significant differences between fixating and deviating eyes (Table 2) or between refractive error groups (Table 3) were identified.
Many devices, including CT, MRI, B-scan ultrasound, and ultrasound biomicroscopy, have been used to scan the anterior segment including extraocular muscles. These imaging modalities are able to diagnose tumors, inflammation, trauma, vascular malformation, infection, and thyroid-associated ophthalmopathy [451112]. Images obtained with CT and MRI can be used to measure total extraocular muscle length and thickness and to evaluate overall orbital area (including the orbital bone). However, these measurements are less accurate and are not suitable to precisely determine extraocular muscle insertion site. Furthermore, these imaging techniques require sedation or general anesthesia in children, expose children to radiation, and are expensive [23]. The B-scan ultrasound can easily detect anatomical structures, but has poor image resolution [4]. In contrast, ultrasound biomicroscopy provides higher resolution images, but image capture is slow and requires the use of a contact lens. These factors make it difficult to obtain high quality images in uncooperative patients, particularly children [5]. The relatively recent development of OCT has overcome these issues. It is noninvasive, fast, inexpensive, and produces high quality images. Studies have shown that anatomical location of extraocular muscles determined using OCT has high concordance with the positions of extraocular muscles determined by surgery, even in eyes that have undergone prior strabismus surgery [78]. Furthermore, OCT determination of extraocular position is highly reproducible and independent of eyeball direction [9]. The current study successfully examined extraocular muscle anatomy using SS-OCT images in 21 of 22 pediatric patients. Only one patient was excluded because of poor quality images due to poor cooperation. This demonstrates that children are able to tolerate OCT imaging well and to cooperate during image capture. Unfortunately, the exact extraocular muscle insertion site is difficult to identify by anterior segment OCT because the boundary between the extraocular muscle tendon and sclera or Tenon's capsule is ambiguous on OCT images. In this study, a clear boundary was easily identified in most cases. The identified location may have been incorrect by up to 1 mm, which is a clinically insignificant distance. These limitations can be overcome with high-resolution OCT [13].
Optical coherence tomography cannot image the oblique muscle or the posterior portion of extraocular muscles. Prior studies have evaluated extraocular muscle insertion site and thickness using ultrasound, CT, and MRI [1415]. Additionally, it has been shown that extraocular muscle thickness significantly increases with age, and that different extraocular muscles have different growth rates [16]. In contrast, our data showed no significant association between extraocular muscle thickness and age. We attribute this discrepancy to the small number of subjects in the study and the narrow age range of the study group, which included only children. In a previous pediatric study using ultrasound biomicroscopy, the reported distance from the medial rectus insertion site to the corneal limbus was 5.2 ± 0.9 mm, while the distance from the lateral rectus insertion site to the corneal limbus was 6.4 ± 0.7 mm [5]. The OCT measurements of these same distances in adults have been reported to be slightly higher (medial rectus insertion to corneal limbus, 5.72 ± 0.6 mm; lateral rectus muscle insertion to corneal limbus, 6.80 ± 0.6 mm) [7]. Our measurement of the medial rectus to corneal limbus distance was 5.01 ± 0.81 mm, comparable to prior studies. However, we report a lateral rectus to corneal limbus distance of 5.20 ± 0.82 mm, which differs from previously reported values. These discrepancies may be due to differing definition of the corneal limbus for each study, differences in measurement methods and differences in patient groups.
According to a previous study comparing scleral thickness between sexes using AS-OCT, male sclera thickness was thicker than female [17]. However, in this study, lateral scleral thickness was significantly thicker in males, but there was no significant difference in medial sclera thickness. These discrepancies may be because the prior study was not limited to pediatric patients and the small number of patients in this study.
This study has several limitations. First, the design was retrospective, and we were unable to evaluate reproducibility. A future, prospective study is needed to investigate this important factor. Second, the relatively small sample size made it difficult to obtain statistically significant results. Further studies with more patients are necessary to elucidate the effect of specific strabismus type and disease duration. Third, poor imaging in uncooperative subjects made it difficult to identify muscle insertion sites and anatomical structures. This limitation undoubtedly introduced measurement error. Fourth, since thickness of the sclera was measured at a single location, there was limited certainty regarding representation of overall scleral thickness. Lastly, all distances were manually measured by a single tester and measurement technique differences from other studies may have introduced variation in data between studies.
Despite these limitations, our study results showed that anterior segment SS-OCT can successfully and comfortably be used to examine extraocular muscles and sclera in children in Korea. Furthermore, anatomical information gained from this technique can be used to help plan treatment or surgery and to detect anatomical abnormalities (e.g., paralytic strabismus, congenital strabismus, and thyroid-associated ophthalmopathy) without equipment such as MRI, which is more expensive and difficult for children to undergo.
Figures and Tables
![]() | Fig. 1Swept-source optical coherence tomography (SS-OCT) image from a 9-year-old boy. (A) External view of the eye showing the medial rectus muscle. The horizontal line represents the part of the eye being imaged. (B) The SS-OCT image of the eye along the corresponding horizontal line in (A). Distance from the corneal limbus to insertion of medial rectus muscle (black arrow), scleral thickness (yellow arrow), and thickness of medial rectus muscle (orange arrow) are also shown and measured in microns with digital caliper. |
Table 1
Comparison of age, SE and ocular parameters between sexes
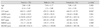
SE = spherical equivalent; LST = lateral scleral thickness; LRMT = lateral rectus muscle thickness; LLTID = lateral limbus to insertion distance; MST = medial scleral thickness; MRMT = medial rectus muscle thickness; MLTID = medial limbus to insertion distance.
*The p-values were calculated using Mann-Whitney test; †Significant difference between groups.
References
1. Dahlmann-Noor AH, Tillaux PJ. P. J. Tillaux: of the way the muscles insert onto the sclera. 1877. Strabismus. 2008; 16:174–175.
2. van der Pol CB, Chakraborty S, Gao J, et al. Imaging anatomy and pathology of extraocular muscles in adults. Can Assoc Radiol J. 2014; 65:366–371.


3. Tian S, Nishida Y, Isberg B, Lennerstrand G. MRI measurements of normal extraocular muscles and other orbital structures. Graefes Arch Clin Exp Ophthalmol. 2000; 238:393–404.


4. Tamburrelli C, Salgarello T, Vaiano AS, et al. Ultrasound of the horizontal rectus muscle insertion sites: implications in preoperative assessment of strabismus. Invest Ophthalmol Vis Sci. 2003; 44:618–622.


5. Watts P, Smith D, Mackeen L, et al. Evaluation of the ultrasound biomicroscope in strabismus surgery. J AAPOS. 2002; 6:187–190.


6. Garcia JP Jr, Rosen RB. Anterior segment imaging: optical coherence tomography versus ultrasound biomicroscopy. Ophthalmic Surg Lasers Imaging. 2008; 39:476–484.


7. Liu X, Wang F, Xiao Y, et al. Measurement of the limbus-insertion distance in adult strabismus patients with anterior segment optical coherence tomography. Invest Ophthalmol Vis Sci. 2011; 52:8370–8373.


8. Ngo CS, Smith D, Kraft SP. The accuracy of anterior segment optical coherence tomography (AS-OCT) in localizing extraocular rectus muscles insertions. J AAPOS. 2015; 19:233–236.


9. Park KA, Lee JY, Oh SY. Reproducibility of horizontal extraocular muscle insertion distance in anterior segment optical coherence tomography and the effect of head position. J AAPOS. 2014; 18:15–20.


10. Yasuno Y, Madjarova VD, Makita S, et al. Three-dimensional and high-speed swept-source optical coherence tomography for in vivo investigation of human anterior eye segments. Opt Express. 2005; 13:10652–10664.


11. Ozgen A, Ariyurek M. Normative measurements of orbital structures using CT. AJR Am J Roentgenol. 1998; 170:1093–1096.


12. Ozgen A, Aydingoz U. Normative measurements of orbital structures using MRI. J Comput Assist Tomogr. 2000; 24:493–496.
13. Wang J, Abou Shousha M, Perez VL, et al. Ultra-high resolution optical coherence tomography for imaging the anterior segment of the eye. Ophthalmic Surg Lasers Imaging. 2011; 42:Suppl. S15–S27.


14. Demer JL, Kerman BM. Comparison of standardized echography with magnetic resonance imaging to measure extraocular muscle size. Am J Ophthalmol. 1994; 118:351–361.


15. Mann K, Schoner W, Maier-Hauff K, et al. Comparative examination of endocrine ophthalmopathy by means of ultrasonography, computerized tomography and fish bioassay. Klin Wochenschr. 1979; 57:831–837.