Abstract
Purpose
To evaluate the thickness and volume of the choroid in healthy Korean children using swept-source optical coherence tomography.
Methods
We examined 80 eyes of 40 healthy children and teenagers (<18 years) using swept-source optical coherence tomography with a tunable long-wavelength laser source. A volumetric macular scan protocol using the Early Treatment Diabetic Retinopathy Study grid was used to construct a choroidal thickness map. We also examined 44 eyes of 35 healthy adult volunteers (≥18 years) and compared adult measurements with the findings in children.
Results
The mean age of the children and teenagers was 9.47 ± 3.80 (4 to 17) vs. 55.04 ± 12.63 years (36 to 70 years) in the adult group (p < 0.001, Student's t-test). Regarding the Early Treatment Diabetic Retinopathy Study subfields, the inner temporal subfield was the thickest (247.96 µm). The inner and outer nasal choroid were thinner (p = 0.004, p = 0.002, respectively) than the surrounding areas. The mean choroidal volumes of the inner and outer nasal areas were smaller (p = 0.004, p = 0.003, respectively) than those of all the other areas in each circle. Among the nine subfields, all areas in the children, except the outer nasal subfield, were thicker than those in adults (p < 0.05). Regression analysis showed that age, axial length, and refractive error correlated with subfoveal choroidal thickness (p < 0.05).
Conclusions
Overall macular choroidal thickness and volume in children and teenagers were significantly greater than in adults. The nasal choroid was significantly thinner than the surrounding areas. The pediatric subfoveal choroid is prone to thinning with increasing age, axial length, and refractive error. These differences should be considered when choroidal thickness is evaluated in children with chorioretinal diseases.
The choroid, which is vascular connective tissue, provides oxygen and nourishment to the outer retina [1]. Because the choroid is associated with the pathophysiology of many diseases affecting the retina, quantitative analysis of choroidal thickness and volume is important in the evaluation of chorioretinal diseases, as well as normal physiology [234567].
Measurements of choroidal thickness have been made possible through the use of enhanced-depth imaging optical coherence tomography (EDI-OCT) [89]. This technique offers a simple, practical method for assessing real-time choroidal sections.
The wavelength of the laser in swept-source OCT (SS-OCT) is longer than that of conventional spectral domain OCT and provides greater penetration through the retinal pigment epithelium, thus enabling precise evaluation of the deeper structures of the eye, including the choroid and retrobulbar optic nerve [1011].
In previous studies using EDI-OCT and SS-OCT, it has been demonstrated that the pediatric choroid tends to thin with age. However, those studies were limited due to insufficient sampling points (or lines) and manual mapping of chorioscleral borders [121314]. Construction of choroidal thickness maps was needed to overcome these limitations. Analysis of choroidal thickness and volume mapping using SS-OCT has been recently reported in normal children and adults [15]. However, to the best of our knowledge, there has been no such report in Korean children.
It is likely that the comprehensive evaluation of pediatric choroidal changes in the absence of any chorioretinal disease would help in understanding choroidal changes associated with retinopathy. The purpose of this study was to determine choroidal thickness and volume by constructing choroidal thickness maps in a Korean pediatric population.
For this purpose, we used a 3-dimensional volumetric macular scan protocol to examine the macular area in healthy pediatric eyes. Using the Early Treatment Diabetic Retinopathy Study (ETDRS) grid [16], we were able to determine mean choroidal thickness and volume in each sector of the grid. Findings in children were compared with measurements in adults.
We studied 80 eyes of 40 healthy children (4 to 17 years) and 44 eyes of 35 healthy adult volunteers (≥18 years) who visited the healthcare clinic at Hanyang University Hospital between July 2013 and January 2014.
All participants were without ophthalmic or systemic signs or symptoms. Standard ophthalmologic examinations included measurement of best-corrected visual acuity, applanation tonometry, slit-lamp biomicroscopy, indirect ophthalmoscopy, auto refractometry (ARK1; Nidek, Gamagory, Japan), and measurement of axial length (IOL-Master, Carl Zeiss Meditec, Jena, Germany).
Exclusion criteria included history of prematurity, systemic disease or previous intraocular surgery; history or evidence of chorioretinal or vitreoretinal disease; refractive errors (spherical equivalent) >6 diopters (D); or eye history that might affect choroidal thickness. The study adhered to the tenets of the Declaration of Helsinki and was approved by the institutional review board of Hanyang University.
The macular area was examined using an SS-OCT instrument (DRI OCT-1; Topcon, Tokyo, Japan) with a tunable laser light-source centered at a wavelength 1,050 nm (tuning range approximately 100 nm). The 3-dimensional volumetric macular scan protocol covered a 6 × 6 mm2 area centered on the fovea with 512 A-scans × 256 B-scans in 0.8 seconds. All examinations were performed by trained examiners. The distance between the outer border of the retinal pigment epithelium and the inner surface of the chorioscleral border was determined using built-in software. Choroidal thickness was mapped using the ETDRS grid, which was comprised of inner and outer rings (diameters, 1 to 3 mm and 3 to 6 mm, respectively) divided into four quadrants: superior, inferior, temporal, and nasal. The grid was divided into nine subfields: inner and outer temporal, inner and outer superior, inner and outer inferior, inner and outer nasal, and the central area (Fig. 1A and 1B). We used measured choroidal thickness to calculate choroidal volume for each sector of the grid. To reduce the effects of diurnal variations, all examinations took place between 14:00 and 17:00 [1718].
Student's t-tests were used to determine the significance of differences in age, axial length, refractive errors, central or macular choroidal thickness, and central choroidal volume between the two groups of participants. Data were analyzed using one-way ANOVA to compare choroidal thickness and volume in each subfield of the grid. Relationships of age, axial length, and refractive error with choroidal thickness and volume were calculated using simple regression analyses. Correlations according to age subgroups were also evaluated. A p-value less than 0.05 was considered statistically significant. The PASW Statistics ver. 18.0 (SPSS Inc., Chicago, IL, USA) was used for all analyses.
Use of an SS-OCT instrument enabled visualization of choroidal thickness for all study participants, and all images appeared normal (Fig. 1). The mean age for children was 9.47 ± 3.80 vs. 55.04 ± 12.63 years for adults ( p < 0.001, Student's t-test). The mean axial length was 24.01 ± 1.36 mm in children vs. 24.38 ± 1.90 mm in adults (p = 0.224). The mean spherical equivalent was -1.66 ± 1.91 D in children vs. -1.34 ± 4.00 D in adults (p = 0.617).
The macular choroid within circles of diameter 1.0, 3.0, and 6.0 mm was thicker in children (245.48 ± 52.36, 245.30 ± 49.52, 237.90 ± 46.00 µm, respectively) than in adults (192.20 ± 121.96, 192.04 ± 98.91, 185.21 ± 87.53 µm; p < 0.05, respectively). The central choroidal volume within a 1.0 mm circle was also greater in children than in adults (0.192 ± 0.041 vs. 0.155 ± 0.095 mm3, p = 0.018) (Table 1).
Overall, in the nine subfields of the ETDRS grid measured in children, the choroid was thickest in the inner temporal area and thinnest in the outer nasal area. In the inner ring, the choroid became thinner in order of the inferior, superior, central subfoveal, and nasal areas. The choroid in both the inner and outer nasal subfields was thinner than in the surrounding areas (both p < 0.005) (Table 2). There were no significant differences in choroidal thickness in the temporal, superior, or inferior subfields.
In the adult group, the central subfoveal choroid was the thickest among the nine subfields. In the inner and outer rings, the superior subfield was the thickest. Thickness decreased in order of the temporal, inferior, and nasal areas. There were no significant differences among the four inner and four outer sectors (both p > 0.05). In the ETDRS mapping of choroidal thickness, all subfields except the outer nasal area were thicker in children than in adults (p < 0.005). Choroidal volumes in the four inner and four outer sectors were largest on the temporal side and smallest on the nasal side. Volumes in the nasal sectors in each ring differed from those in the other three sectors (p < 0.005) (Table 2).
We also compared choroidal thickness in children and adults according to the age groups of 4 to 8, 9 to 12, 13 to 17, 19 to 50, and 51 to 70 years (Fig. 2A and 2B, Table 3). Among children, there was no significant change in choroidal thickness with increasing age, but the pattern (temporal, followed by subfoveal and nasal area) was maintained. Although the difference was small, changes in choroidal thickness with increasing age appeared greater on the temporal side than on the nasal side (p < 0.05) (Fig. 2 and Table 3). Differences in mean choroidal thickness in the superior, central, and inferior quadrants in pediatric groups were not significant, but changes in choroidal thickness appeared greater in the inferior quadrant than in the superior quadrant (Fig. 2).
In an analysis of possible correlations between choroidal thickness and other ocular parameters, simple linear regression analysis showed that choroidal thickness in the central subfield was correlated with axial length and refractive error (p < 0.05). Correlations between macular choroidal thickness and ocular parameters were similar to those for central choroidal thickness (p < 0.05). Age was not related to central or macular choroidal thickness (p > 0.05) (Table 4).
Studies on choroidal thickness using OCT have reported that choroidal thickness is influenced by axial length, refractive error, sex, and age [23192021]. Although adult choroidal thickness has been well evaluated, choroidal thickness studies in children have been limited due to difficulties in patient cooperation. Pediatric choroidal thickness has been investigated using EDI-OCT in a study in Korean children [21]. However, EDI-OCT has potential limitations in the manual measurement of the chorioscleral border.
The light source in SS-OCT is longer than in conventional machines and provides greater penetration, enabling deep choroidal imaging. In addition, the rapid test time and built-in software for the construction of maps using ETDRS grids makes evaluation of pediatric choroidal thickness easier, comprehensive, and objective. Still, there have been no prior studies using SS-OCT to measure choroidal thickness and volume in Korean children.
Previous studies on pediatric subfoveal and macular choroidal thickness have yielded differing results. Using SS-OCT, Ruiz-Moreno et al. [13] and Nagasawa et al. [15] determined mean subfoveal choroidal thickness as 312.9 µm (mean age, 9.6 years) and 260 µm (mean age, 7.9 years), respectively. Using EDI-OCT, Kim et al. [21] determined mean choroidal thickness as 293.13 µm in Korean children with a mean age 7.7 years. Ruiz-Moreno et al. [13] and Nagasawa et al. [15] also determined pediatric macular choroidal thickness to be 240.3 and 285.2 µm, respectively. These previous results differ slightly from ours, probably due to differences in age distribution, race, or scanning protocol.
Using a horizontal line scan, which may be inadequate for evaluation of the entire macular area, Ruiz-Moreno et al. [13] reported that macular choroidal thickness in adults and children did not differ. However, our results show that central and macular choroidal thicknesses were significantly greater in children than in adults, in agreement with the findings of Nagasawa et al. [15].
In previous studies, topographic profiles of the pediatric choroid have shown some differences in thickness, but all concluded that the nasal choroid was the thinnest. We obtained the same result for the nasal choroid and observed some differences in the topographic profile. Kim et al. [21] reported that the choroid was thickest in the temporal area, followed by the subfoveal and nasal areas. Nagasawa et al. [15] reported that the choroid was thickest in the inner temporal area (269.4 µm), followed by the outer temporal (262.0 µm) and subfoveal areas (260.4 µm). Ruiz-Moreno et al. [13] also reported that the temporal choroidal was the thickest, followed by the subfoveal and the nasal choroid areas. We found the same order: temporal, followed by the subfoveal and nasal areas. There were also differences between regions in the vertical topographic profile.
There were some differences in the topographic profile of choroidal thickness between children and adults (Table 2). This is likely because choroidal thickness is associated with age-related changes in the choroidal vasculature. In particular, choroidal vascular remodeling may be associated with choroidal growth and maturation and a greater metabolic demand in the macular area than at the periphery, thus leading to the maintenance of thickness in the central area. In adults, choroidal thickness decrease was greater in the temporal than in the nasal area, and the change of choroidal thickness was greater in the inferior area than in the superior area (Fig. 2 and Table 2).
On simple linear regression analyses, we found that subfoveal and mean choroidal thicknesses were significantly correlated with axial length and spherical equivalent (Fig. 3A-3C). In contrast, Nagasawa et al. [15] reported that choroidal thickness correlated with age, axial length, refractive error, and body mass index, while Kim et al. [21] reported that pediatric choroidal thickness was associated only with axial length. The differences between these two studies and ours may be due to racial differences in the case of Nagasawa et al. [15] or to the wider age range of the children (age up to 17 years) in the case of Kim et al. [21].
In the present study, axial length in children did not differ from that in adults, but was greater (24.01 mm) than in the study (22.96 mm) by Kim et al. [21]. This was possibly because the mean age of our children was higher (9.47 years) than those of Kim et al. [21] (7.67 years). On the other hand, central and macular choroidal thickness was significantly greater in children than in adults in our study. It has been reported that as axial length increases with body growth, the choroid becomes thinner [22]. However, although rapid physical growth in children affects axial elongation in the eye ball, choroidal thickness seems to be more or less constant to adulthood.
To the best of our knowledge, there has been only one report, that of Nagasawa et al. [15], on the measurement of adult choroidal volume using SS-OCT. Evaluation of choroidal volume is important because it can reflect choroidal vascular changes (for example, vascular hyperpermeability or vasodilation) seen in chorioretinal diseases [22]. The thickness of the choroid has been evaluated using several different approaches, including simple measurement at a few points, or using a radial or raster scan protocol. However, such methods can be affected by focal thickening or thinning of the choroid, or by irregularities of the chorioscleral border, and therefore are inadequate for evaluation of the choroid as a whole [91421]. Using SS-OCT, we can construct choroidal maps of volumetric macular scans that reduce the error of the choroidal boundary and enable comprehensive evaluation of choroidal volume, similar to the estimation of retinal volume using spectral domain OCT.
There are several limitations to our study. First, the error due to irregularities in the chorioscleral border in 256 B-scan images was not evaluated. Using SS-OCT, Nagasawa et al. [15] reported a 25.1% error when evaluating normal pediatric choroidal thickness. Although SS-OCT in the normal population has a smaller proportional error with shorter test times and longer wavelengths, the error at the choroidal boundary in volumetric macular scanning needs to be determined. Second, the number of subjects in the age groups differed, especially for mid-to-late teenage subjects, who were relatively low in number. Third, we do not provide an explanation for why these topographic profiles appear in children. This question requires dedicated study, as does the question of why changes in topographic order occur in the macular area.
In summary, choroidal thickness in Korean children is greatest in the inner temporal area, followed by the superior, subfoveal, and nasal choroid areas. The longer axial length in myopia tends to be associated with a thinner choroid. Knowledge of the normal choroidal profile and thickness in pediatric eyes may help in understanding physiologic changes and evaluating chorioretinal conditions in pediatric patients.
Figures and Tables
Fig. 1
Choroidal thickness map obtained using swept-source optical coherence tomography in a healthy 9-year-old girl. The 3-dimensional (D) volumetric macular scan protocol with 512 A-scans × 256 B-scans was used to obtain 3D imaging data of a 6 × 6 mm area. (A) Autosegmentation of the chorioscleral border in the B-scan image. (B) Choroidal thickness map of a 6 × 6 mm area centered on the fovea obtained from analysis of the B-scan images in the 3D data set. Mean choroidal thickness for each sector was obtained by applying the Early Treatment Diabetic Retinopathy Study grid to the map.
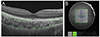
Fig. 2
Pediatric and adult choroidal thinning with increasing age in each (A) horizontal and (B) vertical segment of the Early Treatment Diabetic Retinopathy Study grid. OT = outer temporal; IT = inner temporal; SF = subfoveal; IN = inner nasal; ON = outer nasal; OS = outer superior; IS = inner superior; II = inner inferior; OI = outer inferior.
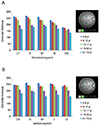
Fig. 3
Scatterplots show the relationship between central choroidal thickness and (A) age, (B) axial length, and (C) refractive error. As age and axial length increased, central choroidal thickness (A,B) decreased. In contrast, as the spherical equivalent increased, central choroidal thickness (C) tended to increase.
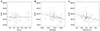
References
1. Harris A, Bingaman D, Ciulla T, Martin B. Retinal and choroidal blood flow in health and disease. In : Ryan SJ, Hinton DR, Schachat AP, Wilkinson P, editors. Retina. 4th ed. Philadelphia: Elsevier;2006. p. 83–102.
2. Kim KH, Kim DG. The relationship among refractive power, axial length and choroidal thickness measured by SD-OCT in myopia. J Korean Ophthalmol Soc. 2012; 53:626–631.
3. Ikuno Y, Kawaguchi K, Nouchi T, Yasuno Y. Choroidal thickness in healthy Japanese subjects. Invest Ophthalmol Vis Sci. 2010; 51:2173–2176.
4. Kim SW, Oh J, Kwon SS, et al. Comparison of choroidal thickness among patients with healthy eyes, early age-related maculopathy, neovascular age-related macular degeneration, central serous chorioretinopathy, and polypoidal choroidal vasculopathy. Retina. 2011; 31:1904–1911.
5. Cho JH, Bae SH, Han JR, et al. Comparison of choroidal thickness in eyes with central serous chorioretinopathy, asymptomatic fellow eyes and normal eyes. J Korean Ophthalmol Soc. 2012; 53:87–93.
6. Chung SE, Kang SW, Lee JH, Kim YT. Choroidal thickness in polypoidal choroidal vasculopathy and exudative age-related macular degeneration. Ophthalmology. 2011; 118:840–845.
7. Manjunath V, Goren J, Fujimoto JG, Duker JS. Analysis of choroidal thickness in age-related macular degeneration using spectral-domain optical coherence tomography. Am J Ophthalmol. 2011; 152:663–668.
8. Spaide RF, Koizumi H, Pozzoni MC. Enhanced depth imaging spectral-domain optical coherence tomography. Am J Ophthalmol. 2008; 146:496–500.
9. Margolis R, Spaide RF. A pilot study of enhanced depth imaging optical coherence tomography of the choroid in normal eyes. Am J Ophthalmol. 2009; 147:811–815.
10. Spaide RF, Akiba M, Ohno-Matsui K. Evaluation of peripapillary intrachoroidal cavitation with swept source and enhanced depth imaging optical coherence tomography. Retina. 2012; 32:1037–1044.
11. Nagasawa T, Mitamura Y, Katome T, et al. Swept-source optical coherence tomographic findings in morning glory syndrome. Retina. 2014; 34:206–208.
12. Hirata M, Tsujikawa A, Matsumoto A, et al. Macular choroidal thickness and volume in normal subjects measured by swept-source optical coherence tomography. Invest Ophthalmol Vis Sci. 2011; 52:4971–4978.
13. Ruiz-Moreno JM, Flores-Moreno I, Lugo F, et al. Macular choroidal thickness in normal pediatric population measured by swept-source optical coherence tomography. Invest Ophthalmol Vis Sci. 2013; 54:353–359.
14. Read SA, Collins MJ, Vincent SJ, Alonso-Caneiro D. Choroidal thickness in childhood. Invest Ophthalmol Vis Sci. 2013; 54:3586–3593.
15. Nagasawa T, Mitamura Y, Katome T, et al. Macular choroidal thickness and volume in healthy pediatric individuals measured by swept-source optical coherence tomography. Invest Ophthalmol Vis Sci. 2013; 54:7068–7074.
16. Early Treatment Diabetic Retinopathy Study design and baseline patient characteristics. ETDRS report number 7. Ophthalmology. 1991; 98:741–756.
17. Brown JS, Flitcroft DI, Ying GS, et al. In vivo human choroidal thickness measurements: evidence for diurnal fluctuations. Invest Ophthalmol Vis Sci. 2009; 50:5–12.
18. Tan CS, Ouyang Y, Ruiz H, Sadda SR. Diurnal variation of choroidal thickness in normal, healthy subjects measured by spectral domain optical coherence tomography. Invest Ophthalmol Vis Sci. 2012; 53:261–266.
19. Kim EJ, Kim JH, Koo SH, et al. Choroidal thickness changes according to the refractive errors and axial length in Korean myopia patients. J Korean Ophthalmol Soc. 2012; 53:1814–1822.
20. Li XQ, Larsen M, Munch IC. Subfoveal choroidal thickness in relation to sex and axial length in 93 Danish university students. Invest Ophthalmol Vis Sci. 2011; 52:8438–8441.
21. Kim JH, Kim JS, Lee KW, Lee JH. The posterior choroidal profiles measured by spectral domain optical coherence tomography in healthy Korean children. J Korean Ophthalmol Soc. 2013; 54:1708–1714.
22. Shin JW, Shin YU, Lee BR. Choroidal thickness and volume mapping by a six radial scan protocol on spectral-domain optical coherence tomography. Ophthalmology. 2012; 119:1017–1023.