Abstract
Purpose
To investigate the effects of vitreomacular traction (VMT) on ranibizumab treatment response for neovascular age-related macular degeneration (AMD).
Methods
A retrospective review of 85 eyes of 85 patients newly diagnosed with neovascular AMD was conducted. Patients were eligible if they had received more than three consecutive monthly ranibizumab (0.50 mg) treatments and ophthalmic evaluations. Patients were classified into a VMT (+) group or VMT (-) group according to optical coherence tomography imaging. Best corrected visual acuity and central retinal thickness (CRT) measurements were obtained at three and six months after initial injection.
Results
One month after the third injection, mean visual acuity (VA) increases of 6.36 and 9.87 letters were observed in the VMT (+) and VMT (-) groups, respectively. The corresponding mean CRT values decreased by 70.29 µm and 121.68 µm, respectively. A total 41 eyes were identified as eligible for a subsequent fourth injection; 71.1% of patients (27 eyes) in the VMT (+) group but only 29.8% of patients in the VMT (-) group needed a subsequent fourth injection. Follow-up was extended to six months for 42 of the 85 enrolled patients (49.4%). The trends in VA and optical coherence tomography were found to be maintained at six-month follow-up.
Ranibizumab (Lucentis; Novartis Pharma AG, Basel, Switzerland; Genentech Inc., San Francisco, CA, USA) is a humanized monoclonal antibody fragment targeting multiple isoforms of human vascular endothelial growth factor (VEGF)-A and has become a standard treatment for neovascular age-related macular degeneration (AMD) [1234567]. Optical coherence tomography (OCT)/visual acuity (VA)-guided, individualized pro re nata (PRN) treatment, after three initial loading doses, is now regarded to be a successful and cost-effective option to monthly treatment, as verified by several studies [89101112]. Additionally, it is important to identify the factors that correlate to OCT/VA outcome in AMD patients receiving customized PRN anti-VEGF treatment [131415]. Although AMD primarily involves outer retinal layers [161718], several authors have suggested that the vitreous plays a role in the pathogenesis and/or progression of AMD, especially in the presence of incomplete posterior vitreous detachment (PVD) and its associated vitreomacular traction (VMT) [19202122232425262728293031]. Furthermore, it has been suggested that vitreomacular adhesion (VMA) and incomplete PVD-related traction force on the fovea contribute to the development and progression of AMD [19232430].
Controversy surrounds VMT and responsiveness to anti-VEGF treatment. Lee and Koh [25] suggested that chronic tractional forces associated with VMA might antagonize the effect of anti-VEGF treatment in their retrospective study using stratus OCT (Carl Zeiss Meditec AG, Jena, Germany). Conversely, Mathew et al. [26] reported that the presence of VMT did not contribute significantly to responsiveness to anti-VEGF treatment at one-year VA prognosis.
The purpose of the present study was to longitudinally assess the effects of VMT in neovascular AMD patients on response to ranibizumab therapy according to spectral domain (SD)-OCT.
We retrospectively reviewed the records of 85 treatment-nave patients newly diagnosed with choroidal neovascularization (CNV) secondary to AMD at the vitreoretinal clinic of Inha University Hospital. Subjects with disease duration less than one month and those who were treated with intravitreal ranibizumab (0.50 mg) for at least three months were selected. After three consecutive monthly loading doses, the fourth monthly treatment was determined according to the criteria recommended by the PrONTO [8] and SUSTAIN [9] studies. In brief, further treatment was performed if intraretinal fluid (IRF) or subretinal fluid (SRF) persisted or recurred regardless of the presence of pigment epithelial detachment (PED). We treated if there was an increase in OCT ≥100 µm, a loss of vision ≥1 line, or if a de novo macular hemorrhage was evident by clinical examination. This study was performed in accordance with the ethical standards of the Declaration of Helsinki and was approved by the institutional review board of Inha University Hospital.
All 85 enrolled patients had received complete monthly ophthalmic evaluations, which included best corrected VA, fluorescein angiography, and SD-OCT. A subset of patients underwent indocyanine green angiography at their initial visit in order to confirm the presence of polypoidal choroidal vasculopathy or retinal angiomatous proliferation. We excluded polypoidal choroidal vasculopathy and retinal angiomatous proliferation patients because their prognosis is worse than that of neovascular AMD, and these patients are frequently refractory to ranibizumab treatment [32333435]. All patients underwent VA assessment using an Early Treatment Diabetic Retinopathy Study (ETDRS) chart, with the use of standardized refraction and testing protocols at a starting test distance of two meters at monthly visits. The Cirrus SD-OCT (Carl Zeiss Meditec, Dublin, CA, USA), high resolution, five-center lined scans were used at each examination in order to evaluate whether fluid was present. Fluid in the macula was identified as IRF, SRF, or PED and was recorded as an OCT finding in the macula [8]. Central retinal thickness (CRT) was used as the measure of retinal thickness.
To be included in the study, patients had to be at least 50 years of age, have a best-corrected VA of 20 / 40 to 20 / 400 (Snellen equivalent, letter score from 20 to 70 letters using the ETDRS chart at two meters), and have primary CNV associated with AMD. Furthermore, eligible patients had lesion types assessed by fluorescein angiography as predominantly classic, minimally classic, or occult with no classic CNV. When calculating lesion areas, we assumed a standard disk diameter of 1.8 mm and a disk area of 2.54 mm2 [4]. The total lesion size included the CNV and also blockage from hemorrhages, blocked f luorescence not from hemorrhages, PED, and fibrosis [4]. Eligible patients were required to have an OCT CRT of at least 250 µm. Eyes with a coexisting disease involving the posterior pole or one that might affect the presence of VMA, such as diabetic retinopathy, inflammatory disease, vascular occlusion, epiretinal membrane, or macular hole, were excluded. Eyes with refractive errors >+3.00 D or <-6.00 D were not included in order to eliminate the influence of refractive errors on PVD [20]. Eyes that had previously undergone laser surgery or vitreoretinal surgery were excluded, as were eyes that had undergone routine cataract surgery within six months from enrollment.
Patients were divided into a VMT (+) group and VMT (-) group as determined by the presence of VMT at the fovea observed using Cirrus SD-OCT (Carl Zeiss Meditec). The vitreoretinal adhesion area was carefully analyzed by a trained investigator (KHL). We defined VMT as a distortion of the retina secondary to an abnormal tractional force exerted on the macula when a steep slope of the inner macular surface or a sharp angulation and localized deformation of the retinal profile was detected at the hyaloid adhesion site and was therefore distinguished from VMA without traction [2328]. Conversely, VMA, as defined, has incomplete PVD on the macula and does not show traction signs such as sharp angulation or inner retinal distortion on the vitreoretinal interface allocated and was excluded from the baseline [28]. Therefore, the VMT (-) group included only eyes that did not show any traction component on the macula with complete PVD.
Statistical analyses were performed using SPSS ver. 18.0 (SPSS Inc., Chicago, IL, USA). To ensure group similarity at baseline, categorical data were assessed using the chisquare test, and continuous variables were compared using the independent t-test. Within each group, results at baseline and after treatment were compared using the paired t-test. A repeated measures analysis of variance was used to compare mean VA and mean CRT values of the two study groups. Independent variables without a Gaussian distribution were compared using the Mann-Whitney U-test. Statistical significance was accepted for p-values <0.05.
The number of enrolled patients assigned to the VMT (+) and VMT (-) groups were limited at 38 patients and 47 patients, respectively. Therefore, the Kolmogorov-Smirnov single-sample test was performed, and it showed that all continuous variables including age, spherical equivalent refractive error, CRT, and VA in both groups fulfilled the requirements for a parametric statistical analysis (all, p > 0.05). Table 1 summarizes group baseline characteristics. No significant intergroup differences were found. In addition, baseline OCT appearances, including CRT, were similar in the two groups (Table 2).
A VA improvement was detected in both groups one month after the first ranibizumab injection and throughout the first three months (Fig. 1). However, the VMT (-) group showed greater improvement in VA during this three-month period (p < 0.05 for all three months). A repeated measures analysis of variance demonstrated a significant difference in mean VA change over time in the VMT (-) group (p = 0.008). In the VMT (-) group, 14 eyes (29.8%) gained at least three lines of VA, and one eye lost more than three lines of VA at three months after the first injection. Conversely, in the VMT (+) group, five eyes (13.2%) gained at least three lines of VA, and more than half of the patients (57.9%) in this group failed to achieve a VA improvement greater than one line (p = 0.032) (Table 3). Improvements in VA were associated with a decrease in CRT, which continued to decrease throughout the first three months (Fig. 2). However, CRT improvement was more significant in the VMT (-) group throughout the three-month period (p < 0.05 for all three months). A repeated measures analysis of variance demonstrated a significant intergroup difference in mean CRT changes in the VMT (-) group (p = 0.003).
As mentioned above, after three monthly loading doses, we administered a fourth ranibizumab injection based on the criteria of the PrONTO [8] and SUSTAIN [9] studies at month three, that is, one month after the third injection. At visits three months after the first injections, 41 eyes were considered eligible for a fourth injection. Of these 41 eyes, 27 (71.1%) in the VMT (+) group needed a fourth injection one month after the third injection, whereas only 29.8% of patients in the VMT (-) group needed a fourth injection.
Only 42 of the 85 study subjects were followed for six months. However, at this time, patient numbers did not fulfill the requirements of parametric statistical analysis. Therefore, non-parametric methods were used to compare median values. Accordingly, 23 eyes in the VMT (+) group were compared with 19 eyes in the VMT (-) group. Baseline characteristics, including VA and CRT, were not significantly different in these two subgroups. Group differences in VA and CRT observed at three months were also observed at six months. At six months after the first injection, median VA increases were 6.0 and 8.5 letters in the VMT (+) and VMT (-) subgroups, respectively (p = 0.026). At this time, VA improvement was associated with a decrease in CRT; median CRT decreased by 85.00 and 120.50 µm in the VMT (+) and VMT (-) subgroups, respectively (p = 0.012).
In our results, mean VA showed greater improvement in the VMT (-) group compared to the VMT (+) group throughout the six months after the initial ranibizumab treatment. Further, corresponding mean CRT values showed a greater decrease in the VMT (-) group compared to the VMT (+) group throughout the six months after the initial ranibizumab treatment. AMD eyes with VMT had a significantly higher rate of nonresponsiveness to anti-VEGF treatment and showed unfavorable short-term outcomes after three loading doses of ranibizumab compared to AMD eyes without VMT. Although the influence of VMT should not be overestimated, mechanical traction forces can antagonize the effect of anti-VEGF treatment. The anterior-posterior traction forces of VMT mainly interact with the vitreoretinal interface and might be directly applied to Müller cells and stretching of the retinal pigment epithelium. This could lead to secretion of various pro-inflammatory cytokines, including VEGF, which plays an important role in the pathogenesis of AMD [2228363738]. These changes might directly affect vitreoretinal adhesion points that induce firmer fibrous attachments and consequently aggravate VMT. Stefansson proposed a mechanism for VMT-related distortion based on Newton's third law of motion [39]. He argued that forces acting in opposite directions within the vitreous apply a traction force to the retina that results in the tissue being pulled apart [2839]. The resulting structural changes can lead to macular edema and could reduce oxygen and nutrient supply to retinal tissues and cause breakdown of the blood-retinal barrier and potentiate disease activity [2228]. Furthermore, VMT also causes local ischemia and disrupts the choroidal supply of macula, consequently increasing the VEGF level [4041]. Therefore, after an anti-VEGF injection to neutralize VEGF, the AMD component stabilizes, and SRF and CNV are reduced. However, the VMT-related mechanical traction force remains and can then trigger macula ischemia and sustained macular edema, eventually affecting visual prognosis (Fig. 3) [42].
Additionally, the anti-VEGF agent itself might increase VMT traction force by causing contraction of the vitreous and posterior hyaloid membrane, thus worsening retinal distortion [43]. Furthermore, there is a possibility that anti-VEGF agents induce chorio-retinal vasoconstriction, which could further increase hypoxic damage [444546474849]. Although a vitrectomy likely accelerates the clearance of drugs injected into the vitreous cavity and possibly undermines the goal of reducing the treatment burden [5051], pharmacologic vitreolysis agents or surgical vitrectomies might be an adjuvant treatment option with VMT and for the resistance to conventional anti-VEGF treatments in select patients [235253].
Our study has several limitations. First, we conducted this retrospective study using medical records. Therefore, we were unable to evaluate a cause and effect relationship between VMT and neovascular AMD. More precisely, we could not determine whether VMT promoted the development or progression of AMD [21545556] or whether AMD promoted VMT through local inflammatory changes that strengthen adhesion between the posterior vitreous face and the internal limiting membrane [2857]. There is also the possibility that VMT rapidly progressed and affected the prognosis independent of the effects of anti-VEGF treatment or AMD progression. A second study limitation is the mean follow-up of only three to six months, which is too short of a period to allow definitive conclusions about treatment response to be drawn. However, the prognosis of neovascular AMD in several studies has been reported to be strongly correlated with an immediate response following three monthly loading doses [456789]. Third, we enrolled a relatively small number of patients, and the sample size was too small to thoroughly evaluate intergroup differences. Therefore, we suggest that a large population-based study be undertaken to confirm our findings.
In conclusion, our results suggest that VMT affects the treatment response to ranibizumab in eyes with AMD. VMT associated with AMD frequently causes chronic traction forces that might antagonize the effect of anti-VEGF treatment and can cause pharmacologic resistance in a subpopulation of patients. Although intravitreal ranibizumab is associated with a substantial short-term reduction in exudation, VMT associated with AMD is much more refractory to intravitreal ranibizumab than AMD without VMT.
Figures and Tables
Fig. 1
Visual acuities of eyes with neovascular age-related macular degeneration treated with three consecutive monthly loadings of ranibizumab. Red and blue lines indicate mean values in the vitreomacular traction (-) and vitreomacular traction (+) groups, respectively. Statistical significance of differences throughout the three-month period was confirmed by p-values of 0.036, 0.016, 0.014, respectively, according to independent t-test.
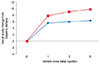
Fig. 2
Central retinal thicknesses of eyes with neovascular age-related macular degeneration treated with ranibizumab over three months. Red and blue lines indicate mean values in the vitreomacular traction (-) and vitreomacular traction (+) groups, respectively. Statistical significance of differences throughout the three-month period was confirmed by p-values of 0.023, 0.018, and 0.007, respectively, according to independent t-test.
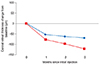
Fig. 3
The anterior-posterior traction forces of vitreomacular traction (VMT) lead to stretching of retinal pigment epithelium (RPE) cells and stimulate Müller cells to release vascular endothelial growth factor (VEGF). VEGF then induces chronic lowgrade inflammation, which directly aggravates VMT. This VMT then completes a vicious cycle by causing local ischemia and consequently increasing VEGF level. BRB = blood-retinal barrier.
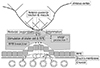
Table 1
Characteristics of patients with and without VMT in eyes with neovascular age-related macular degeneration
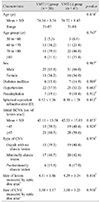
Table 2
Baseline OCT characteristics of patients with and without VMT in eyes with neovascular age-related macular degeneration
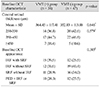
Values are presented as number (%) unless otherwise indicated.
OCT = optical coherence tomography; VMT = vitreomacular traction; IRF = intraretinal fluid; SRF = subretinal fluid; PED = pigment epithelial detachment.
*Independent t-test; †Chi-square test; ‡Chi-square test without "PED ± IRF or SRF" variable.
References
1. Ferrara N, Damico L, Shams N, et al. Development of ranibizumab, an anti-vascular endothelial growth factor antigen binding fragment, as therapy for neovascular age-related macular degeneration. Retina. 2006; 26:859–870.
2. Ferrara N, Mass RD, Campa C, Kim R. Targeting VEGF-A to treat cancer and age-related macular degeneration. Annu Rev Med. 2007; 58:491–504.
3. Witmer AN, Vrensen GF, Van Noorden CJ, Schlingemann RO. Vascular endothelial growth factors and angiogenesis in eye disease. Prog Retin Eye Res. 2003; 22:1–29.
4. Rosenfeld PJ, Brown DM, Heier JS, et al. Ranibizumab for neovascular age-related macular degeneration. N Engl J Med. 2006; 355:1419–1431.
5. Brown DM, Kaiser PK, Michels M, et al. Ranibizumab versus verteporfin for neovascular age-related macular degeneration. N Engl J Med. 2006; 355:1432–1444.
6. Regillo CD, Brown DM, Abraham P, et al. Randomized, double-masked, sham-controlled trial of ranibizumab for neovascular age-related macular degeneration: PIER Study year 1. Am J Ophthalmol. 2008; 145:239–248.
7. Schmidt-Erfurth U, Eldem B, Guymer R, et al. Efficacy and safety of monthly versus quarterly ranibizumab treatment in neovascular age-related macular degeneration: the EXCITE study. Ophthalmology. 2011; 118:831–839.
8. Fung AE, Lalwani GA, Rosenfeld PJ, et al. An optical coherence tomography-guided, variable dosing regimen with intravitreal ranibizumab (Lucentis) for neovascular age-related macular degeneration. Am J Ophthalmol. 2007; 143:566–583.
9. Holz FG, Amoaku W, Donate J, et al. Safety and efficacy of a flexible dosing regimen of ranibizumab in neovascular age-related macular degeneration: the SUSTAIN study. Ophthalmology. 2011; 118:663–671.
10. CATT Research Group. Martin DF, Maguire MG, et al. Ranibizumab and bevacizumab for neovascular age-related macular degeneration. N Engl J Med. 2011; 364:1897–1908.
11. Comparison of Age-related Macular Degeneration Treatments Trials (CATT) Research Group. Martin DF, Maguire MG, et al. Ranibizumab and bevacizumab for treatment of neovascular age-related macular degeneration: two-year results. Ophthalmology. 2012; 119:1388–1398.
12. IVAN Study Investigators. Chakravarthy U, Harding SP, et al. Ranibizumab versus bevacizumab to treat neovascular age-related macular degeneration: one-year findings from the IVAN randomized trial. Ophthalmology. 2012; 119:1399–1411.
13. Lim JH, Wickremasinghe SS, Xie J, et al. Delay to treatment and visual outcomes in patients treated with anti-vascular endothelial growth factor for age-related macular degeneration. Am J Ophthalmol. 2012; 153:678–686. 686.e1–686.e2.
14. Lux A, Llacer H, Heussen FM, Joussen AM. Non-responders to bevacizumab (Avastin) therapy of choroidal neovascular lesions. Br J Ophthalmol. 2007; 91:1318–1322.
15. Teper SJ, Nowinska A, Pilat J, et al. Involvement of genetic factors in the response to a variable-dosing ranibizumab treatment regimen for age-related macular degeneration. Mol Vis. 2010; 16:2598–2604.
16. Klein RJ, Zeiss C, Chew EY, et al. Complement factor H polymorphism in age-related macular degeneration. Science. 2005; 308:385–389.
17. Dewan A, Liu M, Hartman S, et al. HTRA1 promoter polymorphism in wet age-related macular degeneration. Science. 2006; 314:989–992.
18. Mori K, Horie-Inoue K, Kohda M, et al. Association of the HTRA1 gene variant with age-related macular degeneration in the Japanese population. J Hum Genet. 2007; 52:636–641.
19. Krebs I, Glittenberg C, Zeiler F, Binder S. Spectral domain optical coherence tomography for higher precision in the evaluation of vitreoretinal adhesions in exudative age-related macular degeneration. Br J Ophthalmol. 2011; 95:1415–1418.
20. Ondes F, Yilmaz G, Acar MA, et al. Role of the vitreous in age-related macular degeneration. Jpn J Ophthalmol. 2000; 44:91–93.
21. Krebs I, Brannath W, Glittenberg C, et al. Posterior vitreomacular adhesion: a potential risk factor for exudative age-related macular degeneration? Am J Ophthalmol. 2007; 144:741–746.
22. Schulze S, Hoerle S, Mennel S, Kroll P. Vitreomacular traction and exudative age-related macular degeneration. Acta Ophthalmol. 2008; 86:470–481.
23. Mojana F, Cheng L, Bartsch DU, et al. The role of abnormal vitreomacular adhesion in age-related macular degeneration: spectral optical coherence tomography and surgical results. Am J Ophthalmol. 2008; 146:218–227.
24. Lee SJ, Lee CS, Koh HJ. Posterior vitreomacular adhesion and risk of exudative age-related macular degeneration: paired eye study. Am J Ophthalmol. 2009; 147:621–626.e1.
25. Lee SJ, Koh HJ. Effects of vitreomacular adhesion on anti-vascular endothelial growth factor treatment for exudative age-related macular degeneration. Ophthalmology. 2011; 118:101–110.
26. Mathew R, Richardson M, Sivaprasad S. Predictive value of spectral-domain optical coherence tomography features in assessment of visual prognosis in eyes with neovascular age-related macular degeneration treated with ranibizumab. Am J Ophthalmol. 2013; 155:720–726.e1.
27. Green-Simms AE, Bakri SJ. Vitreomacular traction and age-related macular degeneration. Semin Ophthalmol. 2011; 26:137–138.
28. Simpson AR, Petrarca R, Jackson TL. Vitreomacular adhesion and neovascular age-related macular degeneration. Surv Ophthalmol. 2012; 57:498–509.
29. Maier M, Pfrommer S, Burzer S, et al. Vitreomacular interface and posterior vitreomacular adhesion in exudative age-related macular degeneration (AMD): an OCT-based comparative study. Klin Monbl Augenheilkd. 2012; 229:1030–1035.
30. Jackson TL, Nicod E, Angelis A, et al. Vitreous attachment in age-related macular degeneration, diabetic macular edema, and retinal vein occlusion: a systematic review and metaanalysis. Retina. 2013; 33:1099–1108.
31. Weber-Krause B, Eckardt U. Incidence of posterior vitreous detachment in eyes with and without age-related macular degeneration: an ultrasonic study. Ophthalmologe. 1996; 93:660–665.
32. Yannuzzi LA, Slakter JS, Sorenson JA, et al. Digital indocyanine green videoangiography and choroidal neovascularization. 1992. Retina. 2012; 32:Suppl 1. 191.
33. Yannuzzi LA, Sorenson J, Spaide RF, Lipson B. Idiopathic polypoidal choroidal vasculopathy (IPCV). Retina. 1990; 10:1–8.
34. Ross RD, Gitter KA, Cohen G, Schomaker KS. Idiopathic polypoidal choroidal vasculopathy associated with retinal arterial macroaneurysm and hypertensive retinopathy. Retina. 1996; 16:105–111.
35. Stangos AN, Gandhi JS, Nair-Sahni J, et al. Polypoidal choroidal vasculopathy masquerading as neovascular age-related macular degeneration refractory to ranibizumab. Am J Ophthalmol. 2010; 150:666–673.
36. Adamis AP, Shima DT. The role of vascular endothelial growth factor in ocular health and disease. Retina. 2005; 25:111–118.
37. Seko Y, Seko Y, Fujikura H, et al. Induction of vascular endothelial growth factor after application of mechanical stress to retinal pigment epithelium of the rat in vitro. Invest Ophthalmol Vis Sci. 1999; 40:3287–3291.
38. Meyer CH, Toth CA. Retinal pigment epithelial tear with vitreomacular attachment: a novel pathogenic feature. Graefes Arch Clin Exp Ophthalmol. 2001; 239:325–333.
39. Stefansson E. Physiology of vitreous surgery. Graefes Arch Clin Exp Ophthalmol. 2009; 247:147–163.
40. Reese AB, Jones IS, Cooper WC. Macular changes secondary to vitreous traction. Am J Ophthalmol. 1967; 64:Suppl. 544–549.
41. Spaide RF, Armstrong D, Browne R. Continuing medical education review: choroidal neovascularization in age-related macular degeneration: what is the cause? Retina. 2003; 23:595–614.
42. Rotsos T, Sagoo MS, daCruz L, et al. Intravitreal anti-VEGF treatment in eyes with combined choroidal neovascularisation and vitreomacular traction syndrome. Br J Ophthalmol. 2010; 94:1205–1210.
43. Ozsutcu M, Gulkilik G, Ayintap E, et al. Intravitreal bevacizumab may increase diabetic macular edema in eyes with attached posterior vitreous. Case Rep Ophthalmol. 2013; 4:7–10.
44. Manousaridis K, Talks J. Macular ischaemia: a contraindication for anti-VEGF treatment in retinal vascular disease? Br J Ophthalmol. 2012; 96:179–184.
45. Mendrinos E, Mangioris G, Papadopoulou DN, et al. Long-term results of the effect of intravitreal ranibizumab on the retinal arteriolar diameter in patients with neovascular age-related macular degeneration. Acta Ophthalmol. 2013; 91:e184–e190.
46. Papadopoulou DN, Mendrinos E, Mangioris G, et al. Intravitreal ranibizumab may induce retinal arteriolar vasoconstriction in patients with neovascular age-related macular degeneration. Ophthalmology. 2009; 116:1755–1761.
47. Marneros AG, Fan J, Yokoyama Y, et al. Vascular endothelial growth factor expression in the retinal pigment epithelium is essential for choriocapillaris development and visual function. Am J Pathol. 2005; 167:1451–1459.
48. Peters S, Heiduschka P, Julien S, et al. Ultrastructural findings in the primate eye after intravitreal injection of bevacizumab. Am J Ophthalmol. 2007; 143:995–1002.
49. Shah SU, Haller JA. Vitreomacular traction in a case of exudative age-related macular degeneration resistant to anti-VEGF therapy. Acta Ophthalmol. 2012; 90:e569–e570.
50. Kakinoki M, Sawada O, Sawada T, et al. Effect of vitrectomy on aqueous VEGF concentration and pharmacokinetics of bevacizumab in macaque monkeys. Invest Ophthalmol Vis Sci. 2012; 53:5877–5880.
51. Christoforidis JB, Williams MM, Wang J, et al. Anatomic and pharmacokinetic properties of intravitreal bevacizumab and ranibizumab after vitrectomy and lensectomy. Retina. 2013; 33:946–952.
52. Stalmans P, Benz MS, Gandorfer A, et al. Enzymatic vitreolysis with ocriplasmin for vitreomacular traction and macular holes. N Engl J Med. 2012; 367:606–615.
53. Stalmans P, Duker JS, Kaiser PK, et al. Oct-based interpretation of the vitreomacular interface and indications for pharmacologic vitreolysis. Retina. 2013; 33:2003–2011.
54. Anderson DH, Mullins RF, Hageman GS, Johnson LV. A role for local inflammation in the formation of drusen in the aging eye. Am J Ophthalmol. 2002; 134:411–431.
55. Donoso LA, Kim D, Frost A, et al. The role of inflammation in the pathogenesis of age-related macular degeneration. Surv Ophthalmol. 2006; 51:137–152.
56. Zarbin MA. Current concepts in the pathogenesis of age-related macular degeneration. Arch Ophthalmol. 2004; 122:598–614.
57. Ryan SJ, editor. Retina. 3rd ed. Philadelphia: Mosby;2001.