Abstract
Purpose
To compare the thickness of the lamina cribrosa (LC) and vascular factors of early normal-tension glaucoma (NTG) patients with high and low intraocular pressure (IOP) that are expected to be associated with the development of glaucoma.
Methods
Seventy-one Korean NTG patients with low IOP (the highest IOP <15 mmHg, 40 patients) and high IOP (the lowest IOP >15 mmHg, 31 patients) were included in this study. The thickness of LC and vascular factors were compared. The thickness of the LC was measured using the enhanced depth imaging method with spectral domain optical coherence tomography (Heidelberg Spectralis).
Results
The mean thickness of the central LC was 190.0 ± 19.2 µm in the low IOP group and 197.8 ± 23.6 µm in the high IOP group, but there was no statistical significant difference between the two groups (p > 0.05). The prevalence of self-reported Raynaud phenomenon was significantly higher in the low IOP group (33.0%) than the high IOP group (10.3%, p = 0.04).
Conclusions
The laminar thickness did not significantly differ between the high and low IOP groups. However, the prevalence of Raynaud phenomenon was higher in the low IOP groups. These results suggest that the development of glaucoma with low IOP patients may be more influenced by peripheral vasospasm, such as Raynaud phenomenon, rather than laminar thickness in NTG.
Normal-tension glaucoma (NTG) is diagnosed when the patient exhibits an excavated optic nerve head (ONH) and shows the visual field loss that is typical in glaucoma, but do not have elevation of the intraocular pressure (IOP) nor evidence of another cause of optic atrophy. In NTG patients, eyes with high IOP have more pronounced ONH damage and visual field loss than eyes with low IOP [1,2,3,4]. In contrast, NTG patients who have low IOP tend to have a much larger peripapillary atrophy area inferior to the ONH than NTG patients with high IOP [5]. Moreover, Kim et al. [6] found that the localized retinal nerve fiber layer defect was closer to the center of the macula in patients with an IOP below 15 mmHg than in patients with an IOP of between 16 and 21 mmHg. These findings provide indirect evidence that the mechanism of ONH damage differs between NTG patients with low IOP and those with high IOP.
There is growing evidence that the laminar region of the ONH is the principal site of the retinal ganglion cell axonal insult in glaucoma [7]. The structural differences in the lamina cribrosa (LC) are believed to be responsible for the different effects of IOP in the tissues and the LC of the ONH [8]. Several reports have suggested that spectral-domain (SD) optical coherence tomography (OCT) imaging of the human ONH can visualize the LC. The limited view of the posterior border of the LC, however, has made it difficult to study its structure and to measure its thickness [9,10]. Recently, a new OCT approach, known as enhanced depth imaging (EDI)-OCT, recently enabled comprehensive evaluation of the LC configuration [11].
It has also been suggested that IOP-independent factors are more deeply involved in the development and progression of in NTG than in primary open angle glaucoma (POAG). The occurrence of NTG led to efforts to discover causes of glaucoma other than IOP; ischemic insults, inflammation, reduced resistance to IOP, and hemodynamic factors were investigated as possible factors [12,13,14].
This study was conducted to compare the laminar thickness and vascular factors of early NTG patients with high and low IOP that are expected to be associated with the development of glaucoma.
The subjects of this cross-sectional study were 71 Korean NTG patients with low IOP (40 patients) and high IOP (31 patients). In cases where both eyes of the patient were eligible for this study, only one eye was randomly chosen for inclusion. The subjects were recruited from the Cheil Eye Hospital Glaucoma Clinic, Daegu, Korea, between February and June 2012. This study was approved by the Yeungnam University Hospital Institutional Review Board. All the subjects gave their written informed consent to participate in this study, and the tenets of the Declaration of Helsinki were adhered to in this study.
The subjects to be included in this study were required to have newly diagnosed NTG without previous treatment, a best-corrected visual acuity of ≥20 / 40, a spherical refraction within ±6.0 diopters, cylinder correction within ±3.0 diopters, and visual field loss with an mean deviation (MD) ≥-6 dB. Eyes with intraocular diseases or neurologic diseases that could cause visual field loss were excluded. Additionally, patients with an IOP fluctuation above and below 15 mmHg were excluded. Eyes were also excluded if they had a history of ocular surgery or if a good-quality image could not be taken.
NTG was defined according to the following criteria: a diurnal IOP of 21 mmHg or lower without any medication, glaucomatous optic neuropathy, a glaucomatous visual field defect, an open iridocorneal angle, and no neuroradiological evidence of a non-glaucomatous cause of optic nerve damage. A glaucomatous visual field defect was defined as a glaucoma hemifield test result outside the normal limits in at least two consecutive visual field tests or the presence of at least three contiguous test points within the same hemifield on the pattern deviation plot at p < 0.01, with at least one point at p < 0.005. The reliability indices of the visual field test results of eligible patients had to be better than 25%. The patients were divided into two groups according to the IOP level [5,6,15]. The highest IOP in the low IOP group was <15 mmHg and the lowest IOP in the high IOP group was >15 mmHg.
Before the study, each subject underwent comprehensive ophthalmic assessment that included slit-lamp biomicroscopy; measurement of the best-corrected visual acuity, diurnal IOP, and central corneal thickness; dilated stereoscopic examination of the ONH and fundus; color disc photography; red-free retinal nerve fiber layer photography; and visual field examination. The diurnal IOP was measured at 8:30 a.m., 10:30 a.m., 12:30 a.m., 2:30 p.m., 4:30 p.m., and 6:00 p.m., via Goldmann applanation tonometry. The central corneal thickness was measured using an ultrasonic pachymeter (SP-3000; Tomey Corporation, Nagoya, Japan). An average of five readings was made in each eye. Color disc photographs were taken using a fundus camera (CR-2; Canon Inc., Tokyo, Japan). Visual fields were evaluated with achromatic automated perimetry using the 24-2 Swedish Interactive Threshold Algorithm standard program (Humphrey Visual Field Analyzer II; Carl Zeiss-Meditec, Dublin, CA, USA). The prevalence of vascular factors, such as diabetes mellitus, systemic hypertension (HTN), migraine, the Raynaud phenomenon, and sleep apnea, was determined with a questionnaire during the history-taking.
Experienced ophthalmologists performed the EDI-OCT of the ONH using Heidelberg Spectralis (software ver. 5.3; Heidelberg Engineering, Heidelberg, Germany). The method described in a previous report was used [11]. Briefly, the OCT device was set to produce an image of a 15 × 10-degree rectangle for horizontal scans and of a 10 × 15-degree rectangle for vertical scans, centered on the optic disc. These rectangles were scanned with 65 sections, and each section had an average of 40 OCT frames. The position of the LC structures in the lower part of the screen was used to acquire high-quality images. A new upgraded version of the Heidelberg Spectralis program includes an EDI mode, which automatically places the OCT reference plane toward the bottom of the OCT acquisition screen. All subjects underwent EDI-OCT on the first day, a nd a n additional scan was conducted one month later. Three observers (TYL, JWL, and JHK) measured the LC thickness twice in each eye from the obtained EDI-OCT images. The mean of the six measurements was regarded as the LC thickness in each eye. We defined the thickness of the lamina cribrosa as the distance between the anterior and posterior borders of the highly reflective region in the horizontal EDI-OCT cross-sections of the ONH. The measurement of LC thickness was performed as centrally as possible where there was minimal effect of vascular shadows since thick retinal vessels cause shadows in the OCT cross-sectional images that prevent visualization of the structures underlying the vessels (Fig. 1).
Statistical analyses were performed using the PASW ver. 18.0 (SPSS Inc., Chicago, IL, USA). Independent Student's t-tests, chi-squared tests, and Fisher's exact tests were used to compare the data between the groups. To evaluate the intra- and inter-observer reproducibility of the measurement method that was used in this study, three examiners evaluated all the EDI-OCT images of the ONH and the intraclass correlation coefficient was calculated. Fleiss termed scores ≥0.75, between 0.40 a nd 0.75, a nd ≤0.4 as excellent, moderate, and poor, respectively [16]. The statistical significance level was set at p < 0.05.
The mean age of the low IOP and high IOP groups was 60.0 ± 14.4 and 58.0 ± 9.4 years. Between the two groups, we found no significant differences in age (p = 0.57), gender (p = 0.92), family history of glaucoma (p = 0.54), or central corneal thickness (p = 0.91). The spherical equivalent was -0.14 ± 2.1 diopters in the low IOP group and -0.60 ± 1.72 diopters in the high IOP group, but there was no significant difference between the two groups. The mean IOP was significantly higher in the high IOP group (17.3 ± 1.4 mmHg) than in the low IOP group (12.3 ± 1.71 mmHg, p < 0.01). The visual field showed similar stage of glaucoma damage in the two groups in terms of MD and pattern standard deviation (PSD). The MD was -3.6 ± 2.2 dB in the low IOP group and -3.3 ± 2.1 in the high IOP group. The PSD was 3.7 ± 2.4 in the low IOP group and 3.7 ± 2.3 in the high IOP group (Table 1). The prevalence of self-reported Raynaud pheomenon was significantly higher in the low IOP group (33.0%) than the high IOP group (10.3%, p = 0.04). The prevalence of diabetes mellitus (5.0% vs. 12.9%, p = 0.34), HTN (32.5% vs. 16.1%, p = 0.13), migraine (7.5% vs. 9.6%, p = 1.00), and sleep apnea (7.5% vs. 0.0%, p = 0.55) did not differ significantly between low and high IOP groups. The mean thickness of the LC was 190.0 ± 19.2 µm in the low IOP group and 197.8 ± 23.6 µm in high IOP group, but there was no significant difference seen between two groups (Table 2). The LC measurement had excellent intra- and inter-observer reproducibility (Table 3).
The laminar thickness in the early NTG patients was measured by imaging the ONH with EDI-OCT. The mean laminar thickness of the low IOP group (190.0 µm) was slightly thinner than that of the high IOP group (197.8 µm), but the difference was not significant.
Damage to retinal ganglion cell axons in the LC is the principal pathophysiology that underlies glaucomatous vision loss and ONH cupping [17,18]. The ONH is particularly interesting from a biomechanical perspective because it is a weak spot in an otherwise strong corneo-scleral envelope. The LC structurally and functionally supports the retinal ganglion cell axons as they pass from the high-pressure environment in the eye to a low-pressure region in the retrobulbar cerebrospinal space [19]. The structural change in the LC may deform the laminar pores. This process may subject the axons passing through the pores to extension, compressive, and shearing stress, which will result in the loss of the neuronal function of the axons. Moreover, the ONH is nourished by the short posterior ciliary arteries, which penetrate the immediate peripapillary sclera to feed the capillaries in the LC. The deformation and compression of the lamina may alter the blood supply and consequently, the nutrient delivery to the axons, which will also contribute to axonal injury [19,20,21].
The recent development of the SD-OCT and EDI techniques has enabled enhanced visualization of the lamina structures and measurement of the thickness of human eyes [9,11,22]. In a study by Inoue et al. [9], the LC in living human eyes was measured using three-dimensional SD-OCT. It revealed that the thickness could be correlated with the concurrently measured MD. Poor visualization of the deeper portion of the LC was believed to have been the primary limitation in the imaging and measurement of the LC using SD-OCT. Using the EDI technique in SD-OCT, the full-thickness LC was clearly visualized in all examined eyes [11]. Park et al. [23] found, using EDI-OCT, that the lamina was thinner in NTG patients than in POAG patients and thinner in NTG patients with disc hemorrhage than in NTG patients without disc hemorrhage. Among Korean patients, the mean laminar thickness in early NTG patients was 192 µm, and the measured mean laminar thickness of Japanese early glaucoma patients was 198 µm [9,23]. These findings are very similar to those of this study. Moreover, other studies regarding early POAG patients reported a central laminar thickness of 219 to 277 µm [11,23], which is thicker than that in the early NTG patients in this study. This result indicates that the thinner lamina may more easily lead to deformation of the lamina and a block in axonal transport, even at a normal IOP.
Many previous studies that focused on the pathogenesis of glaucomatous optic neuropathy in NTG patients were conducted from the perspectives of various vascular theories as well as IOP-related mechanical theories, including of LC [24,25,26]. Raynaud phenomenon and migraine are considered manifestations of primary vascular dysregulation syndrome, which causes unstable ocular perfusion and reperfusion injury [27]. In addition, sleep apnea causes glaucoma due to decreased ocular blood flow [28]. Accordingly, the low IOP group had a significantly higher prevalence of Raynaud phenomenon in this study. However, other vascular factors were not related to development of NTG in the low IOP group.
This study had a few limitations. First, the sample size was small. Second, the prevalence of vascular risk factors was assessed on the basis of the questionnaire data, which might have had limited reliability and accuracy because of their reliance on patient recall.
In conclusion, the laminar thickness did not significantly differ between the high and low IOP groups. However, the prevalence of Raynaud phenomenon was higher in low IOP groups. These results suggest that the development of glaucoma with low IOP patients may be more influenced by peripheral vasospasm, such as Raynaud phenomenon, rather than laminar thickness in NTG.
Figures and Tables
Fig. 1
(A) Fundus photograph and (B,C) spectral-domain optical coherence tomography (OCT) images in the enhanced depth imaging (EDI) mode in a 78-year-old woman with normal-tension glaucoma. The mean intraocular pressure was 10 mmHg. In (A), the center of the optic nerve head (ONH) was identified at the point where the vertical center (line α) of the ONH met the horizontal center from which the trunk of the central retinal vessels came out from the ONH (line β). In (B), in the horizontal cross-sectional view of the EDI-OCT, the lamina cribrosa thickness (204 µm) was measured along line α, between lines β-1 and β-2. In (C), both the anterior and posterior borders (delineated with white arrows) of the lamina cribrosa were identified in the EDI-OCT.
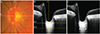
References
1. Cartwright MJ, Anderson DR. Correlation of asymmetric damage with asymmetric intraocular pressure in normal-tension glaucoma (low-tension glaucoma). Arch Ophthalmol. 1988; 106:898–900.
2. Crichton A, Drance SM, Douglas GR, Schulzer M. Unequal intraocular pressure and its relation to asymmetric visual field defects in low-tension glaucoma. Ophthalmology. 1989; 96:1312–1314.
3. Haefliger IO, Hitchings RA. Relationship between asymmetry of visual field defects and intraocular pressure difference in an untreated normal (low) tension glaucoma population. Acta Ophthalmol (Copenh). 1990; 68:564–567.
4. Jonas JB, Grundler AE, Gonzales-Cortes J. Pressure-dependent neuroretinal rim loss in normal-pressure glaucoma. Am J Ophthalmol. 1998; 125:137–144.
5. Wang XH, Stewart WC, Jackson GJ. Differences in optic discs in low-tension glaucoma patients with relatively low or high pressures. Acta Ophthalmol Scand. 1996; 74:364–367.
6. Kim DM, Seo JH, Kim SH, Hwang SS. Comparison of localized retinal nerve fiber layer defects between a low-teen intraocular pressure group and a high-teen intraocular pressure group in normal-tension glaucoma patients. J Glaucoma. 2007; 16:293–296.
7. Bellezza AJ, Rintalan CJ, Thompson HW, et al. Deformation of the lamina cribrosa and anterior scleral canal wall in early experimental glaucoma. Invest Ophthalmol Vis Sci. 2003; 44:623–637.
8. Burgoyne CF, Downs JC. Premise and prediction-how optic nerve head biomechanics underlies the susceptibility and clinical behavior of the aged optic nerve head. J Glaucoma. 2008; 17:318–328.
9. Inoue R, Hangai M, Kotera Y, et al. Three-dimensional high-speed optical coherence tomography imaging of lamina cribrosa in glaucoma. Ophthalmology. 2009; 116:214–222.
10. Strouthidis NG, Grimm J, Williams GA, et al. A comparison of optic nerve head morphology viewed by spectral domain optical coherence tomography and by serial histology. Invest Ophthalmol Vis Sci. 2010; 51:1464–1474.
11. Lee EJ, Kim TW, Weinreb RN, et al. Visualization of the lamina cribrosa using enhanced depth imaging spectral-domain optical coherence tomography. Am J Ophthalmol. 2011; 152:87–95.e1.
12. Drance SM, Sweeney VP, Morgan RW, Feldman F. Studies of factors involved in the production of low tension glaucoma. Arch Ophthalmol. 1973; 89:457–465.
13. Rojanapongpun P, Drance SM. The response of blood flow velocity in the ophthalmic artery and blood flow of the finger to warm and cold stimuli in glaucomatous patients. Graefes Arch Clin Exp Ophthalmol. 1993; 231:375–377.
14. Gasser P. Ocular vasospasm: a risk factor in the pathogenesis of low-tension glaucoma. Int Ophthalmol. 1989; 13:281–290.
15. Shirakashi M, Funaki S, Funaki H, et al. Measurement of retinal nerve fibre layer by scanning laser polarimetry and high pass resolution perimetry in normal tension glaucoma with relatively high or low intraocular pressure. Br J Ophthalmol. 1999; 83:353–357.
16. Fleiss JL. The design and analysis of clinical experiments. New York: Wiley;1986. p. 1–32.
17. Anderson DR, Hendrickson A. Effect of intraocular pressure on rapid axoplasmic transport in monkey optic nerve. Invest Ophthalmol. 1974; 13:771–783.
18. Quigley H, Anderson DR. The dynamics and location of axonal transport blockade by acute intraocular pressure elevation in primate optic nerve. Invest Ophthalmol. 1976; 15:606–616.
19. Downs JC, Roberts MD, Burgoyne CF. Mechanical environment of the optic nerve head in glaucoma. Optom Vis Sci. 2008; 85:425–435.
20. Sigal IA, Flanagan JG, Tertinegg I, Ethier CR. Predicted extension, compression and shearing of optic nerve head tissues. Exp Eye Res. 2007; 85:312–322.
21. Sigal IA, Flanagan JG, Tertinegg I, Ethier CR. Modeling individual-specific human optic nerve head biomechanics. Part I: IOP-induced deformations and influence of geometry. Biomech Model Mechanobiol. 2009; 8:85–98.
22. Park SC, De Moraes CG, Teng CC, et al. Enhanced depth imaging optical coherence tomography of deep optic nerve complex structures in glaucoma. Ophthalmology. 2012; 119:3–9.
23. Park HY, Jeon SH, Park CK. Enhanced depth imaging detects lamina cribrosa thickness differences in normal tension glaucoma and primary open-angle glaucoma. Ophthalmology. 2012; 119:10–20.
24. Kondo Y, Niwa Y, Yamamoto T, et al. Retrobulbar hemodynamics in normal-tension glaucoma with asymmetric visual field change and asymmetric ocular perfusion pressure. Am J Ophthalmol. 2000; 130:454–460.
25. Park HY, Jung KI, Na KS, et al. Visual field characteristics in normal-tension glaucoma patients with autonomic dysfunction and abnormal peripheral microcirculation. Am J Ophthalmol. 2012; 154:466–475.
26. Tielsch JM, Katz J, Sommer A, et al. Hypertension, perfusion pressure, and primary open-angle glaucoma: a population-based assessment. Arch Ophthalmol. 1995; 113:216–221.
27. Flammer J, Mozaffarieh M. What is the present pathogenetic concept of glaucomatous optic neuropathy? Surv Ophthalmol. 2007; 52:Suppl 2. S162–S173.
28. Karakucuk S, Goktas S, Aksu M, et al. Ocular blood flow in patients with obstructive sleep apnea syndrome (OSAS). Graefes Arch Clin Exp Ophthalmol. 2008; 246:129–134.