Abstract
Purpose
Cancer-associated retinopathy is a paraneoplastic retinal degeneration which may primarily result from auto-immune mediated apoptosis. It has been hypothesized that high titer of auto-antibodies are able to cross the blood-retinal barrier (BRB) and to enter retinal cells to activate apoptotic pathway which has been already well-established. However, it still remains to be elucidated whether auto-antibodies could cross BRB in the retina. Herein, we demonstrated that intravenously administrated anti-recoverin antibodies could not pass through BRB and not lead to retinal cell death.
Methods
Anti-recoverin antibody was intravenously injected to C57BL/6 mice, which were sacrificed 1 and 7 days to obtain eye. Vascular endothelial growth factor was intravitreally injected to induce BRB breakdown, which was confirmed by fluorescein angiography and western blotting for zonula occludens (ZO)-1, ZO-2 and occludin. To investigate the location of anti-recoverin antibody in the retina, immunofluorescein was performed. The retinal toxicity of intravenous anti-recoverin antibody was evaluated by histological examination and transferase-mediated dUTP nick-end labeling. Immunofluorescein staining for glial fibrillary acidic protein was done to address glial activation as well.
Results
Intravenously administrated anti-recoverin antibodies were exclusively distributed on retinal vessels which were co-localized with CD31, and led to neither increase of glial fibrillary acidic protein expression, as an indicator of retinal stress, nor apoptotic retinal cell death. Moreover, even in the condition of vascular endothelial growth factor-induced BRB breakdown, anti-recoverin antibodies could not migrate across BRB and still remained on retinal vessels without retinal cytotoxicity.
Cancer-associated retinopathy (CAR) is a paraneoplastic neuronal degeneration in the retina of a cancer patient and is characterized by the rapid, progressive loss of vision [1]. The CAR has been described to occur with small-cell lung cancer, breast cancer, gynecological cancers, and others, in which the remote effects of cancers may stem from autoimmune responses of serum autoantibodies against both tumor and retinal proteins [2,3]. Pathologically, CAR is the degeneration of photoreceptors, with relative sparing of the inner retina, including ganglion cells, bipolar cells, amacrine cells, and horizontal cells, which are supplied by retinal vessels [1-3]. Although the precise mechanism has not been fully established, CAR may primarily result from autoimmune-mediated apoptosis, which is supported by the lack of inflammatory response to the retinal degeneration of CAR [4,5]. Among those autoantibodies found in sera of patients with CAR, those against the 23-kDa retinal protein recoverin appear to be the most common [1,6].
Recoverin is a cytoplasmic calcium-binding protein that is distributed in photoreceptor cells, bipolar cells, and in a small population of cells in the ganglion cell layer [7]. In CAR, it has been hypothesized that a high titer of autoantibodies are able to cross the blood-retinal barrier (BRB) and penetrate susceptible retinal cells, which is followed by cross-reactivity with particular retinal proteins [1,2]. Binding of the anti-recoverin antibody to recoverin enhances rhodopsin phosphorylation, continuously opens cGMP-gated channels, and increases the intracellular calcium level, which leads to activation of the caspase-dependent apoptotic pathway [5]. It has been already well-established that autoantibodies can enter and kill retinal cells [4,5], whereas it has never been established whether autoantibodies can cross the BRB [8,9]. In addition, although intravitreally injected autoantibodies successfully induced retinal degeneration and dysfunction similar to those of CAR [10,11], the intravitreal autoantibody injection is somehow different from the natural pathogenesis of CAR, in which it is hypothesized that circulating autoantibodies that cross the BRB enter retinal cells to induce cell death.
To maintain a stable environment in order to ensure the appropriate activities of retinal neurons, retinal vessels that supply the inner retina form the specialized structure of the BRB [11], which is regulated by modulating both angiogenesis and tight junction formation, as described in our previous findings [12,13]. The BRB is actually located in retinal endothelial cells, whose specific junction molecules are requisite for the maintenance of the barrier function [13,14]. In retinal endothelial cells, tight junction proteins such as zonula occludens (ZO)-1 and occludin are well-characterized, the expressions of which are inversely related to permeability in the BRB [13,15,16]. In a variety of retinal pathologies including uveoretinitis, diabetic retinopathy, or age-related macular degeneration, diverse mechanisms might affect the integrity of the BRB and lead to breakdown [13,14]. Although less is understood about increased vascular permeability and extravasation from the bloodstream in BRB breakdown, it has been proposed that either process may occur to allow for migration through intercellular endothelial tight junctions and for trans-cellular migration through endothelial cells [17,18].
In this study, we demonstrated that intravenously administrated anti-recoverin antibodies could not pass through the BRB and, therefore, do not cause retinal cell death. Moreover, even under the condition of BRB breakdown, anti-recoverin antibodies could not cross the BRB and remained on retinal vessels without retinal cytotoxicity.
Female C57BL/6 mice (eight- to ten-weeks-old, body weight 22 ± 1.6 g) were purchased from Samtako (Seoul, Korea). Care, use, and treatment of all animals in this study were in strict compliance with the ARVO Statement for the Use of Animals in Ophthalmic and Vision Research. The mice were housed under standard 12-hour dark-light cycles at approximately 23℃ room temperature. After intravenous administration of anti-recoverin antibodies, the mice were sacrificed and enucleated at one and seven days. Each group contained ten animals. The enucleated eyes were immersion-fixed in 4% paraformaldehyde and subsequently embedded in paraffin.
Anti-recoverin polyclonal antibodies (Chemicon International Inc., Temecula, CA, USA) were used in this study and were formulated in rabbit serum. According to the manufacturer's specifications, the antibody has specificity for recoverin with immunogenicity for recombinant human recoverin and reacts with mouse recoverin, as well as those of primates, including humans. Anti-recoverin antibodies (40 µL, 1 mg/mL) were intravenously administrated after dialysis to achieve systemic applications.
Human retinal microvascular endothelial cells (HRMECs) were purchased from the Applied Cell Biology Research Institute (Kirkland, WA, USA) and grown on attachment factor-coated plates in complete medium (Cell Systems, Kirkland, WA, USA). The HRMECs used in this study were taken from passages four to six.
Four-micron-thick serial sections were prepared from paraffin blocks. Sections were deparaffinized and hydrated via sequential immersion in xylene and graded alcohol solutions, treated with proteinase K for 5 minutes at 37℃, and then treated with normal serum obtained from the same species in which the secondary antibody was developed for 10 minutes to block nonspecific staining. To stain intravenously administrated anti-recoverin antibodies, slides were incubated with FITC-conjugated IgG (1:400; Santa Cruz Biotechnology, Santa Cruz, CA, USA) and TRITC-conjugated IgG (1:400; Jackson ImmunoResearch, Philadelphia, PA, USA). To stain endothelial cells or astrocytes, slides were incubated overnight at 4℃ with rabbit polyclonal antibody against CD31 for endothelial cells (1:100, Santa-Cruz Biotechnology) or glial fibrillary acidic protein (GFAP) for astrocytes (1:100; Dako, San Francisco, CA, USA). FITC-conjugated IgG (1:400, Santa Cruz Biotechnology) and TRITC-conjugated IgG (1:400, Jackson ImmunoResearch) were used as secondary antibodies. For staining of nuclei, slides were stained with 10 µg/mL 4', 6-diamidino-2-phenolindole (Sigma-Aldrich Co., St. Louis, MO, USA). The slides were observed under a fluorescence microscope (BX50; Olympus, Tokyo, Japan).
Four-micron-thick serial sections were prepared from paraffin blocks. Transferase-mediated dUTP nick-end labeling (TUNEL) staining was performed with a kit (ApopTag; Intergen, Purchase, NY, USA), according to the manufacturer's instructions. TUNEL-positive cells were evaluated by two masked and independent observers (KJH and KJH) who viewed ten randomly-selected fields in each slide at a 400× magnification using a fluorescence microscope (BX50, Olympus).
According to our recent study [16], one day following intravitreal injection of 20 ng/ml vascular endothelial growth factor (VEGF, Sigma-Aldrich Co.), deeply anesthetized mice were perfused through the tail vein with high molecular weight (MW= 500,000) fluorescein-conjugated dextran (Sigma-Aldrich Co.). At 1 hour post-perfuson, the eyes were enucleated and fixed in 4% paraformaldehyde for 2 hours. The retinas were dissected, flat-mounted in Dako mounting medium (DakoCytomation, Glostrup, Denmark), and viewed under fluorescence microscopy (BX50, Olympus) at 400×. These experiments were repeated at least three times.
Western blotting was performed using standard Western blotting methods. The protein concentration was measured using a BCA protein assay kit (Pierce, Rockford, IL, USA). Equal amounts of protein were separated via electrophoresis on a 5% to 10% SDS-PAGE gel and transferred electrophoretically onto a nitrocellulose membrane (Amersham, Little Chalfont, UK). The membranes were blocked for 30 minutes in 5% non-fat milk. Next, the membranes were incubated overnight with anti-CD31 (Santa Cruz Biotechnology), anti-ZO-1 (1:1000; Zymed, San Francisco, CA, USA), anti-ZO-2 (1:2000, Zymed), and anti-occludin (1:1000, Zymed) at 4℃. After they were washed with phosphate balanced solution (PBS-T), the membrane was incubated for 1 hour at room temperature with horseradish peroxidase-conjugated anti-rabbit IgG or anti-mouse IgG (1:10,000; Pierce) in PBS-T and 1% non-fat milk. To ensure the equal loading of protein in each lane, the blots were stripped and reprobed with an antibody against β-actin. Intensity values were normalized relative to control values. The blots were scanned using a flatbed scanner, and the band intensity was analyzed using the TINA software program (Raytest, Straubenhardt, Germany).
To investigate whether intravenously administrated anti-recoverin antibodies could cross the BRB, we injected 40 µg of anti-recoverin antibodies into the tail veins of C57BL/6 mice. As shown in Fig. 1A, anti-recoverin antibodies were exclusively detected in the inner retina, including the ganglion cell layer, inner plexiform layer, inner nuclear layer, and outer plexiform layer, whose distribution interestingly seemed to be confined to the retinal vessels.
To address the distribution of anti-recoverin antibodies on retinal vessels, we compared the distribution of anti-recoverin antibodies with the expression of CD31, an endothelial cell marker. Surprisingly, anti-recoverin antibodies were nearly co-localized with CD31 expression one day following injection, and the distribution pattern remained for seven days after injection, although much of the anti-recoverin antibodies disappeared from the retina (Fig. 1B).
To examine whether intravenously administrated anti-recoverin antibodies could induce retinal cell death, based on the previous reports of apoptotic cell death induced by autoantibodies, we evaluated apoptotic cell death in the retina using a TUNEL assay. As demonstrated in Fig. 2A, intravenously administrated anti-recoverin antibodies never induced retinal apoptotic cell death, as would be expected if they could not cross the BRB. In addition, we investigated whether intravenously administrated anti-recoverin antibodies could up-regulate GFAP expression in the retina as an indicator of retinal stress [19]. The injection of anti-recoverin antibodies never induced a progressive increase in GFAP in Mueller cells (Fig. 2B).
To evaluate whether intravenously administrated anti-recoverin antibodies could pass through the BRB under the conditions of BRB breakdown, we induced VEGF-mediated BRB breakdown via intravitreal injection of 20 ng/mL VEGF and analyzed the passage of autoantibodies across the BRB. Intravitreal injection of VEGF disrupts the BRB and induces profuse leakage from retinal vessels (Fig. 3A), which is closely linked to a decrease in tight junction proteins in retinal endothelial cells (Fig. 3B) [14,18]. Even during BRB breakdown, intravenously administrated anti-recoverin antibodies were confined to retinal vessels without passage across the BRB, were co-localized with CD31, and were detected on retinal vessels with a similar frequency to that of the control (Fig. 3C).
To examine whether intravenously administrated anti-recoverin antibodies under BRB breakdown conditions could induce retinal apoptotic cell death, a TUNEL assay was performed. As demonstrated in Fig. 4, there was no significant change in apoptotic cell count with or without BRB breakdown.
CAR has been thought to be mediated by the autoimmune reactions triggered by antigenic mimicry, where anti-recoverin antibody plays a crucial role in the pathogenesis of CAR, resulting in retinal degeneration [2,4-6]. It is hypothesized that autoantibodies against recoverin develop from a tumor antigen resembling one shared by recoverin, and a high titer of circulating antibodies may increase the possibility of passage through the BRB. Although retinal degeneration in CAR may be mediated by both humoral and cellular immune mechanisms [20], it is not clear whether either plays a major role. As proposed in other autoimmune diseases, anti-recoverin antibodies are able to enter into retinal cells and induce apoptotic cell death, which has been demonstrated in retinal tissue in vivo as well as in an in vitro retinal cell culture [4,5,9,10]. However, we still do not know how anti-recoverin antibodies pass through the BRB because anti-recoverin antibodies were intravitreally injected in the in vivo experiments.
In this study, we demonstrated that intravenously administrated anti-recoverin antibodies could not pass through the BRB. Based on the high titer of autoantibodies in CAR patient serum samples [1,2], it has been hypothesized that a high titer of anti-recoverin antibodies is consequently related to penetration through the BRB. In addition, cancer patients with a low titer of anti-recoverin antibodies were reported to demonstrate no visual dysfunction [8]. Given that the blood volume in mice is about 85 to 95 mL/kg [21], and the body weight of the mice used in our experiment was 22 ± 1.6 g, the amount of intravenous injection, 40 µg, is a relatively high titer compared to the serum concentration of CAR patients [1,2]. However, anti-recoverin antibodies were exclusively observed in the retinal vessels. Interestingly, anti-recoverin antibodies still remained on retinal vessels for seven days after intravenous administration, although the frequency decreased. Furthermore, intravenous administration of anti-recoverin antibodies never induced any retinal stress, confirmed by a lack of change in GFAP expression, as well as no retinal cell death. It is reasonable to speculate that these results occurred as a result of no anti-recoverin antibodies crossing the BRB.
Based on retinal vascular changes indicating BRB breakdown in CAR [22], the pathogenesis of CAR might be linked to BRB breakdown. The BRB is a selective barrier that represents the boundary between the systemic circulation and the retinal neuron. Vascular permeability in the retina is therefore actively regulated by the BRB, which is established with a tight junction restricting the paracellular flux of molecules [12,13,18]. VEGF is causally linked to BRB breakdown through the dysregulation of the tight junction, which leads to increased retinal vascular permeability [13,18]. In addition, VEGF upregulates intracellular adhesion molecule (ICAM)-1 in retinal endothelial cells, which increases leukocyte adhesion [23].
The ICAM-1 in endothelial cells activates a Rho A-mediated signal to allow leukocytes to paracellularly migrate across the barrier through the interendothelial cell junction or transcellularly through the endothelial cell itself [17]. In this study, we induced BRB breakdown via intravitreal injection of VEGF, which might enhance penetration of intravascular anti-recoverin antibodies into the retina. Surprisingly, there was no passage of anti-recoverin antibody through the BRB in the case of VEGF-induced BRB breakdown. In addition, anti-recoverin antibodies were observed on retinal vessels with a similar frequency to that of the control, which in part supports the idea that penetration of anti-recoverin antibodies through the BRB might not be mediated by regulation of the tight junction in restriction of paracellular flux, although this is not certain for the transcellular route. The interpretation of this result is only speculative and requires further investigation.
In conclusion, intravenously administrated anti-recoverin antibodies could not pass through the BRB, which therefore does not induce either retinal stress or retinal cell death. Interestingly, intravascular anti-recoverin antibodies could not migrate into the retina even in VEGF-induced BRB breakdown, which also was not accompanied by an increase in retinal cell death. On the basis of the available evidence, we suggest that intravascular anti-recoverin antibodies could not naturally penetrate into the retina, and BRB breakdown mediated by dysregulation of the tight junction might not be sufficient to allow anti-recoverin antibodies to pass through the BRB. Further investigations are required to elucidate other transporting routes of intravascular anti-recoverin antibodies to the retina and for the sustained existence of intravenous administrated anti-recoverin antibodies on retinal vessels.
Acknowledgements
We thank Chang Sik Cho for support with our experimental techniques. This study was supported by the National R&D program for cancer control, Ministry of Health & Welfare, Republic of Korea (800-20100332), by the Mid-Career Researcher Program (2010-0009050) of MEST/NRF, and by the Seoul National University Hospital Research Fund (04-2007-0280).
References
1. Thirkill CE, Roth AM, Keltner JL. Cancer-associated retinopathy. Arch Ophthalmol. 1987; 105:372–375. PMID: 2950846.


2. Thirkill CE, FitzGerald P, Sergott RC, et al. Cancer-associated retinopathy (CAR syndrome) with antibodies reacting with retinal, optic-nerve, and cancer cells. N Engl J Med. 1989; 321:1589–1594. PMID: 2555714.


3. Khan N, Huang JJ, Foster CS. Cancer associated retinopathy (CAR): an autoimmune-mediated paraneoplastic syndrome. Semin Ophthalmol. 2006; 21:135–141. PMID: 16912011.


4. Chen W, Elias RV, Cao W, et al. Anti-recoverin antibodies cause the apoptotic death of mammalian photoreceptor cells in vitro. J Neurosci Res. 1999; 57:706–718. PMID: 10462694.


5. Maeda T, Maeda A, Maruyama I, et al. Mechanisms of photoreceptor cell death in cancer-associated retinopathy. Invest Ophthalmol Vis Sci. 2001; 42:705–712. PMID: 11222531.
6. Adamus G, Aptsiauri N, Guy J, et al. The occurrence of serum autoantibodies against enolase in cancer-associated retinopathy. Clin Immunol Immunopathol. 1996; 78:120–129. PMID: 8625554.


7. Wiechmann AF, Hammarback JA. Expression of recoverin mRNA in the human retina: localization by in situ hybridization. Exp Eye Res. 1993; 57:763–769. PMID: 8150028.


8. Bazhin AV, Shifrina ON, Savchenko MS, et al. Low titre autoantibodies against recoverin in sera of patients with small cell lung cancer but without a loss of vision. Lung Cancer. 2001; 34:99–104. PMID: 11557119.


9. Bazhin AV, Slepova OS, Tikhomirova NK. Retinal degeneration under the effect of antibodies to recoverin. Bull Exp Biol Med. 2001; 131:350–352. PMID: 11550024.
10. Adamus G, Machnicki M, Elerding H, et al. Antibodies to recoverin induce apoptosis of photoreceptor and bipolar cells in vivo. J Autoimmun. 1998; 11:523–533. PMID: 9802939.
11. Ohguro H, Ogawa K, Maeda T, et al. Cancer-associated retinopathy induced by both anti-recoverin and anti-hsc70 antibodies in vivo. Invest Ophthalmol Vis Sci. 1999; 40:3160–3167. PMID: 10586938.
12. Lee SW, Kim WJ, Choi YK, et al. SeCKS regulates angiogenesis and tight junction formation in blood-brain barrier. Nat Med. 2003; 9:900–906. PMID: 12808449.
13. Choi YK, Kim JH, Kim WJ, et al. AKAP12 regulates human blood-retinal barrier formation by downregulation of hypoxia-inducible factor-1alpha. J Neurosci. 2007; 27:4472–4481. PMID: 17442832.
14. Kim JH, Kim JH, Park JA, et al. Blood-neural barrier: intercellular communication at glio-vascular interface. J Biochem Mol Biol. 2006; 39:339–345. PMID: 16889675.


15. Kim JH, Yu YS, Kim JH, et al. The role of clusterin in in vitro ischemia of human retinal endothelial cells. Curr Eye Res. 2007; 32:693–698. PMID: 17852194.
16. Kim JH, Kim JH, Yu YS, et al. Recruitment of pericytes and astrocytes is closely related to the formation of tight junction in developing retinal vessels. J Neurosci Res. 2009; 87:653–659. PMID: 18816791.


17. Engelhardt B, Wolburg H. Mini-review: transendothelial migration of leukocytes: through the front door or around the side of the house? Eur J Immunol. 2004; 34:2955–2963. PMID: 15376193.


18. Erickson KK, Sundstrom JM, Antonetti DA. Vascular permeability in ocular disease and the role of tight junctions. Angiogenesis. 2007; 10:103–117. PMID: 17340211.


19. Lewis GP, Fisher SK. Up-regulation of glial fibrillary acidic protein in response to retinal injury: its potential role in glial remodeling and a comparison to vimentin expression. Int Rev Cytol. 2003; 230:263–290. PMID: 14692684.


20. Maeda A, Maeda T, Liang Y, et al. Effects of cytotoxic T lymphocyte antigen 4 (CTLA4) signaling and locally applied steroid on retinal dysfunction by recoverin, cancer-associated retinopathy antigen. Mol Vis. 2006; 12:885–891. PMID: 16917481.
21. Riches AC, Sharp JG, Thomas DB, Smith SV. Blood volume determination in the mouse. J Physiol. 1973; 228:279–284. PMID: 4687099.


22. Suzuki T, Obara Y, Sato Y, et al. Cancer-associated retinopathy with presumed vasculitis. Am J Ophthalmol. 1996; 122:125–127. PMID: 8659589.


23. Miyamoto K, Khosrof S, Bursell SE, et al. Vascular endothelial growth factor (VEGF)-induced retinal vascular permeability is mediated by intercellular adhesion molecule-1 (ICAM-1). Am J Pathol. 2000; 156:1733–1739. PMID: 10793084.


Fig. 1
Intravenously administrated anti-recoverin antibodies could not pass through the blood-retinal barrier. After intravenous administration of anti-recoverin antibodies (1 mg/mL, 400 µL), the mice were sacrificed and enucleated before and one and seven days after injection. (A) Immunofluorescence staining for anti-recoverin antibody and 4', 6-diamidino-2-phenolindole (DAPI) staining for nuclei were performed. (B) Immunofluorescence staining of anti-recoverin antibody and CD31 as an endothelial cell marker and DAPI staining for nuclei were performed. Arrow indicates merged immunofluorescence of anti-recoverin antibody and CD31. Asterisk indicates CD31-positive immunoreactivity without anti-recoverin antibody. Quantitative analysis for CD31- and anti-recoverin antibody-positive spots relative to all anti-recoverin antibody-positive spots was performed by measuring ten randomly-selected fields on each slide at 400×. Each point represents the mean (SD) of six independent experiments, *p < 0.05. Scale bar, 100 µm. GCL = ganglion cell layer; IPL=inner plexiform layer; INL = inner nuclear layer; OPL=outer plexiform layer; ONL = outer nuclear layer; HPF=high power field.
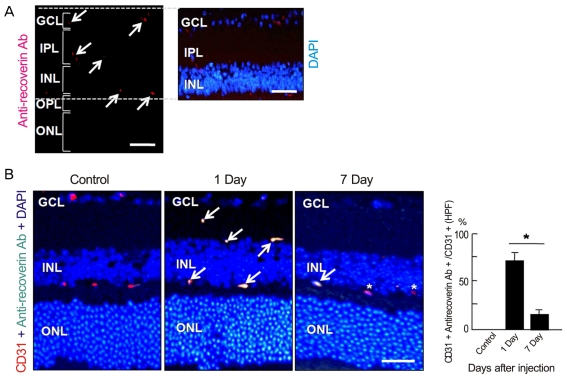
Fig. 2
Intravenously administrated anti-recoverin antibodies never induced cytotoxicity in the retina. After intravenous administration of anti-recoverin antibodies (1 mg/mL, 400 µL), the mice were sacrificed and enucleated before and one and seven days after injection. (A) The apoptotic cells in the sagittal sections from 12 to 6 o/c through the cornea parallel to the optic nerve were assessed using a transferase-mediated dUTP nick-end labeling (TUNEL) assay. TUNEL-positive cells were measured in ten randomly-selected fields on each slide at 400x. Each point represents the mean (SD) of six independent experiments, **p > 0.05. (B) Immunofluorescence staining of glial fibrillary acidic protein (GFAP), an astrocyte marker, was performed. Scale bar, 100 µm. DAPI = 4', 6-diamidino-2-phenolindole; GCL = ganglion cell layer; INL = inner nuclear layer; ONL = outer nuclear layer; HPF=high power field.
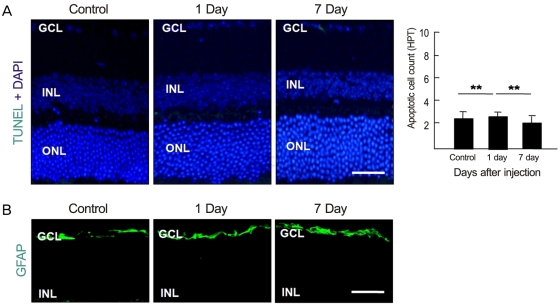
Fig. 3
Intravascular anti-recoverin antibodies could not pass through the blood-retinal barrier (BRB) in the case of BRB breakdown. (A) Fluorescein angiography using high molecular weight (500,000) fluorescein-conjugated dextran was performed to evaluate vascular endothelial growth factor (VEGF)-induced vascular leakage in the retina. One day following intravitreal injection of 20 ng/mL VEGF in 1 µL PBS or PBS only to mice, a whole mount retinal preparation was performed 1 hour post-perfusion with fluorescein-conjugated dextran. These experiments were repeated six times with similar results. Scale bar, 100 µm. (B) Human retinal microvascular endothelial cells were incubated for 12 hours with 20 ng/mL VEGF. Western blot analysis for zonula occludens (ZO)-1, ZO-2, and occludin was performed. β-Actin served as the loading control. Quantitative analysis was performed by measuring protein expression relative to that of the control. Each point represents the mean (SD) of three independent experiments. *p < 0.05. Figures were selected as representative data from three independent experiments. (C) Intravenous administration of anti-recoverin antibodies (1 mg/mL, 400 µL) was performed one day after intravitreal injection of 20 ng/mL VEGF. The mice were sacrificed and enucleated one day after anti-recoverin antibody injection. Immunofluorescence staining of anti-recoverin antibody and CD31 as an endothelial cell marker and 4', 6-diamidino-2-phenolindole (DAPI) staining for nuclei were performed. Arrow indicates merged immunofluorescence of anti-recoverin antibody and CD31. Quantitative analysis for CD31- and anti-recoverin antibody-positive spots relative to all anti-recoverin antibody-positive spots was performed by measuring ten randomly-selected fields on each slide at 400×. Each point represents the mean (SD) of six independent experiments, *p < 0.05. Scale bar, 100 µm. GCL = ganglion cell layer; INL = inner nuclear layer; ONL = outer nuclear layer; HPF=high power field.
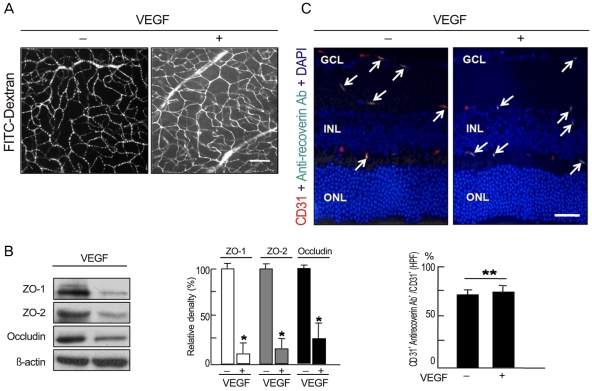
Fig. 4
Intravenously administrated anti-recoverin antibodies under blood-retinal barrier breakdown conditions never increased retinal cell death. Intravenous administration of anti-recoverin antibodies (1 mg/mL, 400 µL) was performed one day after intravitreal injection of 20 ng/mL vascular endothelial growth factor (VEGF). The mice were sacrificed and enucleated one and seven days after anti-recoverin antibody injection. The apoptotic cells in the sagittal sections from 12 to 6 o/c through the cornea parallel to the optic nerve were assessed using a transferase-mediated dUTP nick-end labeling (TUNEL) assay. TUNEL-positive cells were measured in ten randomly-selected fields on each slide at 400×. Each point represents the mean (SD) of six independent experiments, **p > 0.05. Scale bar, 100 µm. DAPI = 4', 6-diamidino-2-phenolindole; GCL = ganglion cell layer; INL = inner nuclear layer; ONL = outer nuclear layer; HPF=high power field.
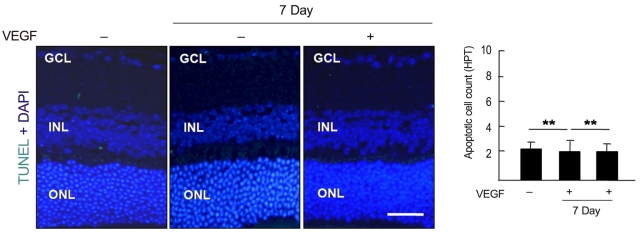