Abstract
Purpose
To determine the clinical utility of using photopic negative response (PhNR) by comparing the parameters for normal, primary open angle glaucoma (POAG) and diabetic retinopathy (DR).
Methods
Electroretinography (UTAS E-3000) was performed in 12 normal, 12 POAG, and 12 DR subjects. Amplitudes and implicit times for PhNR were compared among the three groups. The mean deviation (MD) and pattern standard deviation (PSD) were evaluated using standard automated perimetry (SAP). The mean retinal nerve fiber layer (RNFL) thickness and cup-disc ratio were measured using optical coherence tomography.
Results
The a-waves and b-waves were not different among the three groups. However, compared to normal subjects, the PhNR amplitudes were reduced, and the PhNR implicit times were prolonged in the POAG and DR patients (p<0.001, p<0.001). The MD and RNFL thickness were correlated with the amplitudes and implicit times for the PhNR.
Electroretinography (ERG) has been used since the 1940s to evaluate retinal function. This procedure records electrical potentials that originate from cells in the retinal tissue [1-4]. ERG is a useful diagnostic tool in clinical practice. Fundus photography and fluorescein fundus angiography (FA) can reveal anatomical and structural changes, but ERG can estimate the overall functional status of the retina. A typical ERG reveals three individual waves. The a-wave is the first emerging negative potential in the ERG. It originates from and represents the function of the photoreceptor cells. The b-wave is the abrupt positive potential that follows the a-wave, and it indicates the functional activities of Müller cells and bipolar cells. Finally, oscillatory potentials (OPs) are the 3-4 small waves that are included in the ascending portion of the b-wave. OPs originate in the inner plexiform layer (IPL) which receives its nutrient supply from the retinal blood flow. OPs represent the status of the microvascular circulation in the IPL.
The photopic negative response (PhNR) is the negative potential that follows the b-wave. The PhNR is an electrical signal that originates in the retinal ganglion cells (RGC) and their axons and may reflect inner retinal function. Previous studies have indicated that the PhNR may be helpful for the detection of early glaucoma and may also be useful in the evaluation of retinal function for patients with retinal ischemic disorders, such as diabetic retinopathy or central retinal vein occlusion [5-8]. However, there are an insufficient number of clinical studies involving PhNR in Korea.
In this study, the parameters of PhNR were measured in a population of normal Korean adults. In addition, we studied the clinical utility of PhNR by comparing the PhNR parameters of normal patients to those with glaucoma or diabetic retinopathy.
Thirty-six subjects (72 eyes) were involved in this prospective study, with 12 patients assigned to each of the three groups, the normal group, the primary open angle glaucoma (POAG) group, and the diabetic retinopathy (DR) group. Adults who had no significant general medical or ophthalmologic histories and who had a visual acuity of 20/20 were included in the normal group. Subjects who had myopia or hyperopia over 6.0 diopters (D) were excluded from the normal group. The POAG group included patients who had early or advanced glaucoma with intraocular pressures over 21 mmHg, open angle glaucoma with specific glaucomatous changes on their optic discs, corresponding visual field defects, and no other causative factors that could have caused an elevation of the intraocular pressure. Patients were excluded from the POAG group if they were only suspected to have glaucoma or if they had end-stage glaucoma. Patients were included in the DR group if a fundus examination revealed that they had moderate or severe non-proliferative diabetic retinopathy, according to the Modified Airlie-House classification; patients were excluded if they had a history of panretinal photocoagulation, focal or grid laser photocoagulation, or an intravitreal or posterior subtenon triamcinolone acetonide injection.
All of the subjects in each group underwent an ERG in both eyes using the UTAS-E3000® system (LKC Technologies Inc., Gaithersburg, MD, USA). Pupil dilation was performed by three instillations of 1% tropicamide and 2.5% phenylephrine hydrochloride. The three doses were administered five minutes apart. After the dilation, light adaptation was achieved using 30 cd/m2 background illumination for ten minutes to measure the cone response. An automated alarm was used to minimize the differences in adaptation duration between the subjects. After light adaptation, a corneal electrode was implanted under topical anesthesia with 0.5% proparacaine (Alcaine®; Alcon-Couvreur, Puurs, Belgium). A Burian-Allen corneal contact lens electrode was used. One percent methylcellulose was spread over the surface of the electrode to prevent mechanical damage to the cornea. The ground electrode was placed on the earlobe ipsilateral to the tested eye, and a reference electrode was attached to the center of the forehead. Full-field light stimulation was then produced using the Ganzfeld dome. A Grass PS22 (Grass-Telefactor; Astro-Med Inc., West Warwick, RI, USA) was used to generate light stimuli, a white stimulus applied at an intensity of 2.4297 cd·s/m2 under a 30 cd/m2 white background illumination. The duration of the stimulation was limited to less than five milliseconds (ms), and the interval between stimuli was greater than two seconds. Electrical signals from the electrode were amplified 1000 times and were digitalized using an analog-to-digital converter. High cutoff and low cutoff limits for the signals were set at 500 Hz and 0.3 Hz, respectively. The averaged results from five repeated measurements were used in this study. The PhNR amplitude was defined as the difference between the baseline and the peak of the negative wave following the b-wave (Fig. 1). The implicit time was defined as the interval between the stimulation and the peak of the negative wave. In order to reduce interpersonal variations, a single skilled technician determined the peak point of the wave. In order to estimate the differences in PhNR parameters according to disease, the mean amplitudes and mean implicit times for the PhNR were compared among the three groups. In addition, the a-waves and b-waves from the subjects in the three groups were also measured and compared.
Automated perimetry (SITA 30-2, Humphrey® Field Analyzer II; Carl Zeiss Meditec, Dublin, CA, USA) was used to evaluate subjects in the normal and POAG groups. It was also used to estimate the correlations between the diagnostic parameters for glaucoma and PhNR, as well as to measure the mean deviation (MD) and pattern standard deviation (PSD). Optical coherence tomography (Cirrus® OCT; Carl Zeiss Meditec) was used to measure the mean thicknesses of the retinal nerve fiber layers (RNFL) and the cup-disc ratios (C/D ratio).
Statistical analysis was performed using linear regressions, Mann-Whitney U-tests, and Kruskal-Wallis H tests. SPSS ver. 15.0 (SPSS Inc., Chicago, IL, USA) was used for all statistical calculations.
A total of 36 subjects and 72 eyes from 20 males and 16 females were involved in this study. The mean age for all of the subjects was 56.8±21.2 years (range, 24 to 81 years). The mean ages were not significantly different among the three groups (p=0.068) (Table 1).
In the normal group, the mean amplitude of the a-wave was 34.67±18.43 uV, and its mean implicit time was 16.21±1.02 ms. The mean amplitude and implicit time for the b-wave were 102.65±14.84 uV and 28.88±2.45 ms, respectively. The mean amplitude of the PhNR in the normal group was 30.67±10.02 uV, and the mean implicit time was 44.01±1.94 ms.
In the POAG group, the mean a-wave amplitude was measured at 33.62±20.55 uV, and the implicit time was 16.10±0.90 ms. The mean b-wave amplitude was 103.89±20.05 uV, and its mean implicit time was 29.12±2.46 ms. The mean amplitude of the PhNR in the POAG group was 15.83±8.18 uV, and its mean implicit time was 15.83±8.18 ms.
In the DR group, the mean amplitude and implicit time for the a-wave were 31.96±19.78 uV and 16.03±0.97 ms, respectively. The mean b-wave amplitude was 100.56±19.33 uV, and its mean implicit time was 29.40±2.50 ms. The mean amplitude of the PhNR in the DR group was 18.06±9.05 uV, and its mean implicit time was 49.11±3.20 ms.
There were no significant differences in the amplitudes of the a-waves and b-waves among the three groups (p=0.632, p=0.821) (Table 1, Fig. 2). Similarly, the implicit times of the a-waves and b-waves showed no significant differences among the three groups (p=0.975, p=0.263). However, the amplitudes of the PhNR were significantly reduced in the POAG and DR groups compared to that of the normal group (p<0.001, Kruskal-Wallis H test) (Fig. 2). The implicit times of the PhNR were also significantly prolonged in the POAG and DR groups compared to that of the normal group (p<0.001, Kruskal-Wallis H test) (Fig. 2). In particular, the PhNR implicit time of the DR group was significantly prolonged compared to those of the other groups (p<0.001, p<0.001, Mann-Whitney U-test). The amplitudes of the PhNR in the POAG and DR groups were reduced and the implicit times were prolonged (Fig. 3, 4)
Among the diagnostic parameters for glaucoma, MD showed a significant correlation with the amplitude (p=0.027, R2=0.122) and implicit time of the PhNR (p<0.001, R2=0.340) (Fig. 5). However, three patients who had an MD less than 20 dB revealed improvement in the PSD. Therefore, we reanalyzed the results excluding those three patients. The MD remained correlated with the amplitude (p=0.032, R2=0.288) and implicit time of the PhNR (p=0.017, R2=0.132). In addition, the RNFL thickness was now correlated with the amplitude (p=0.007, R2=0.178) and implicit time of the PhNR (p<0.001, R2=0.391) (Fig. 6). However, the PSD had no correlation with the amplitude or implicit time of the PhNR (p=0.932, p=0.155). The C/D ratio was also not correlated with the parameters of the PhNR (p=0.201, p=0.073) (Fig. 6).
ERG is a diagnostic tool that is used to record the electrical responses of retinal tissues to light stimulation. ERG waves are the summation of multiple signals that originate from neural and non-neural cells in the retina. All responses from retinal cells are expressed and visualized as negative and positive electrical waves. Therefore, based on an analysis and comparison of these waves, we are able to evaluate retinal function and to recognize specific retinal lesions [9]. Among the various ERG waves, PhNR was first reported by Viswanathan et al. in 1999 [10]. PhNR is known to correlate with dysfunction of the inner retina [10]. Several reports have shown a correlation between the amplitudes of the PhNR wave and the existence of ocular diseases such as glaucoma, retinal vascular disease, and hereditary retinal diseases [5,6].
The PhNR is represented by the negative wave following the b-wave. It originates from the RGC and their axons [5-8]. RGCs have voltage-gated sodium channels, allowing them to take part in the generation of an electrical potential via the Na+-K-exchange. Viswanathan et al. [10] compared the ERG findings between normal eyes and tetrodotoxin-injected eyes in monkeys. Tetrodotoxin inhibits the electrical activity of RGCs and amacrine cells. The results of their study showed that the PhNR amplitude was reduced and the implicit time was prolonged in the tetrodotoxin-injected eyes. Therefore, the investigators verified that the PhNR wave originates in the RGC layer [10].
Glaucoma occurs as a result of the degeneration of RGCs and the retinal nerve fiber layer. In patients who have diabetic retinopathy, the RGC layer is at risk for damage due to retinal ischemia. Therefore, RGC atrophy and IPL and internal limiting membrane degeneration may occur. The PhNR wave represents the functional status of the inner retina. We measured the amplitudes and implicit times of the PhNRs in normal subjects, POAG patients, and diabetic retinopathy patients. Based on the results of the PhNR parameters, we sought to reveal the phases of the PhNR parameters according to the disease and to determine the clinical utility of PhNR waves.
In this study, the PhNR amplitude was reduced and the implicit time was prolonged in the POAG group compared to those of the normal group. Glaucoma is a disease that affects RGCs and their axons. Conversely, photoreceptor cells, Müller cells, and bipolar cell functions may be relatively preserved in patients with glaucoma. The amplitudes and implicit times of the a-wave and b-wave are not significantly different between glaucoma patients and normal subjects. Therefore, the PhNR wave may reflect the functional statuses of RGCs and their axons. Viswanathan et al. [6] compared the PhNR amplitudes between POAG patients and normal subjects. They reported that the PhNR amplitude was significantly lower in POAG patients compared to that of normal subjects, similar to the results of the present study [6]. Colotto et al. [7] also noted that the PhNR amplitude was reduced in POAG patients. However, they also noted that there was no difference in PhNR implicit time between the POAG and normal groups [7]. These findings differed from those of the present study. We believe that the differences are attributable to dissimilarities in the ERG procedure and the selection of the patients.
The amplitude and implicit time of the PhNR wave were significantly correlated with MD. Conversely, these values did not correlate with PSD. The PhNR parameters were correlated with the RNFL thickness, but not with the C/D ratio. The MD is the average of the differences between the measured thresholds for each retinal point tested and the age-corrected normal. The PSD is the average of the 17 worst tested points and can reveal the relatively depressed areas in the individual visual field, which can then be used to eliminate the generalized noise derived from a source such as cataract. The PhNR showed a significant correlation with MD but not with PSD because the PhNR represents the electrical potential obtained from full-field light stimulation. In addition, the amplitudes and implicit times of the PhNR were correlated with the mean RNFL thickness. This finding is thought to indicate a correlation between RNFL thickness and RGC electrical activity [11]. Conversely, the PhNR parameters showed no correlation with the C/D ratio, as the C/D ratio varied in the normal population, as well as in the glaucoma patients [12,13].
The PhNR amplitude was reduced and the implicit time was prolonged in DR patients compared to those of normal subjects. In this study, the implicit time was more significantly delayed in the DR patients than it was in the glaucoma patients. Increased glutamate in the vitreous bodies of the DR patients induced degeneration of retinal tissue cells, which played an important role in retinal ischemia [14,15], as RGCs are particularly vulnerable to glutamate-induced cellular toxicity [15]. The reduced amplitudes and prolonged implicit times of the PhNR waves may indicate cellular toxicity in the RGCs and their axons due to increased intravitreal glutamate. Kizawa et al. reported that the PhNR/b-wave amplitude ratio was normal in DR patients, but the ratio was significantly reduced as the DR became more severe [16]. These results suggest that the RGCs incur more severe damage from diabetic changes than do the cone cells or bipolar cells.
There are some limitations to this study. First, only 12 patients were included in each group. Second, the glaucoma patients were not subdivided. Therefore, patients with early onset glaucoma in addition to those with advanced or end-stage glaucoma may have been included in the POAG groups. Third, moderate to severe NPDR patients were involved in this study. The DR patients were not subdivided into mild, moderate, or severe NPDR and PDR. For these reasons, further studies regarding the phases of the PhNR waves according to disease subtype are needed.
In conclusion, the PhNR wave is clinically useful for the evaluation of retinal function in patients with diseases that are characterized by inner retinal dysfunction, including glaucoma and diabetic retinopathy. In order to optimize the clinical application of the PhNR wave, it is necessary to standardize the intensity and duration of the stimulation and the intensity of the background illumination. In addition, further studies in larger populations are necessary to investigate the PhNR waves in patients with other retinal diseases.
Figures and Tables
Fig. 1
Characteristic waveform of a photopic negative response (PhNR). The PhNR is the slow negative potential following the b-wave in photopic electroretinography.
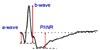
Fig. 2
(A, B) Comparison of a-waves, b-waves and photopic negative responses (PhNRs) among the three groups. The amplitudes and implicit times of the a-waves and b-waves were not significantly different among the groups.
*Amplitudes of the PhNR waves in the primary open angle glaucoma (POAG) and diabetic retinopathy (DR) groups were significantly reduced compared to that of the normal group (p<0.001, Kruskal-Wallis H test). †The implicit times in the POAG and DR groups were significantly prolonged compared to that of the normal group (p<0.001, Kruskal-Wallis H test).
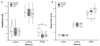
Fig. 3
The photopic negative response (PhNR) waves from the normal (A) and primary open angle glaucoma (POAG) groups (B). Normal subjects showed normal cup-disc (C/D) ratios and no visual field defects. Glaucoma patients had retinal nerve fiber layer (RNFL) defects, large C/D ratios, and glaucomatous visual field defects. The PhNR waves in POAG patients had reduced amplitudes and prolonged implicit times compared to those of the normal subjects (p<0.001, p=0.006, Mann-Whitney U-test).
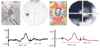
Fig. 4
The photopic negative response (PhNR) waves from a normal (A) and a diabetic retinopathy (DR) patient (B). The normal subject showed normal fundus findings, while the DR patient had evidence of non-proliferative diabetic retinopathy, including multiple retinal hemorrhages and exudates. The PhNR wave in the DR patient had a reduced amplitude and a prolonged implicit time compared to those of the normal subject (p<0.001, p<0.001, Mann-Whitney U-test).
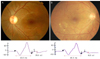
Fig. 5
Correlations among the photopic negative response (PhNR) waves, mean deviations (MD), and pattern standard deviations (PSD) in the normal and primary open angle glaucoma (POAG) groups. The MD was correlated with the amplitudes and implicit times of the PhNR in POAG patients (A: p=0.027 r2=0.122, B: p<0.001, r2=0.340). However, the PSD had no correlation with the amplitudes or implicit times of the PhNR waves in POAG patients (C: p=0.932, D: p=0.155).
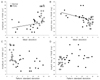
Fig. 6
Correlations among the photopic negative response (PhNR) waves, mean retinal nerve fiber layer (RNFL) thicknesses, and cup-disc (C/D) ratios in patients with primary open angle glaucoma (POAG). The mean RNFL thickness was correlated with the amplitude and implicit time of the PhNR wave in POAG patients (A: p=0.007, r2=0.157, B: p<0.001, r2=0.391). However, the C/D ratios showed no correlation with the amplitude or implicit time of the PhNR wave in POAG patients (C: p=0.201, D: p=0.073).
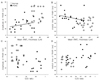
Notes
References
1. Marmor MF, Arden GB, Nisson SE, et al. Standard for clinical electroretinography. Arch Ophthalmol. 1989. 107:816–819.
2. Marmor MF, Zrenner E. Standard for clinical electroretinography. Doc Ophthalmol. 1995. 89:199–210.
3. Marmor MF. An updated standard for clinical electroretinography. Arch Ophthalmol. 1995. 113:1375–1376.
4. Marmor MF. The ERG is alive and well. Arch Ophthalmol. 1995. 113:1371–1372.
5. Drasdo N, Aldedasi YH, Chiti Z, et al. The s-cone PHNR and pattern ERG in primary open angle glaucoma. Invest Ophthalmol Vis Sci. 2001. 42:1266–1272.
6. Viswanathan S, Frishman LJ, Robson JG, Walters JW. The photopic negative response of the flash electroretinogram in primary open angle glaucoma. Invest Ophthalmol Vis Sci. 2001. 42:514–522.
7. Colotto A, Falsini B, Salgarello T, et al. Photopic negative response of the human ERG: losses associated with glaucomatous damage. Invest Ophthalmol Vis Sci. 2000. 41:2205–2211.
8. Machida S, Gotoh Y, Tanaka M, et al. Predominant loss of the photopic negative response in central retinal artery occlusion. Am J Ophthalmol. 2004. 137:938–940.
9. Korean Society for Clinical Electrophysiology of Vision (KSCEV). Korean Society for Clinical Electrophysiology of Vision. Clinical electrophysiology of vision. 2009. Seoul: Korean Society for Clinical Electrophysiology of Vision;36–45.
10. Viswanathan S, Frishman LJ, Robson JG, et al. The photopic negative response of the macaque electroretinogram: reduction by experimental glaucoma. Invest Ophthalmol Vis Sci. 1999. 40:1124–1136.
11. Ventura LM, Sorokac N, De Los Santos R, et al. The relationship between retinal ganglion cell function and retinal nerve fiber thickness in early glaucoma. Invest Ophthalmol Vis Sci. 2006. 47:3904–3911.
12. Barkana Y, Harizman N, Gerber Y, et al. Measurements of optic disk size with HRT II, Stratus OCT, and funduscopy are not interchangeable. Am J Ophthalmol. 2006. 142:375–380.
13. Jonas JB, Budde WM, Panda-Jonas S. Ophthalmoscopic evaluation of the optic nerve head. Surv Ophthalmol. 1999. 43:293–320.
14. Ambati J, Chalam KV, Chawla DK, et al. Elevated gamma aminobutyric acid, glutamate and vascular endothelial growth factor levels in the vitreous of patients with proliferative diabetic retinopathy. Arch Ophthalmol. 1997. 115:1161–1166.
15. Nakazawa T, Takahashi H, Nishijima K, et al. Pitavastatin prevents NMDA-induced retinal ganglion cell death by suppressing leukocyte recruitment. J Neurochem. 2007. 100:1018–1031.
16. Kizawa J, Machida S, Kobayashi T, et al. Changes of oscillatory potentials and photopic negative response in patients with early diabetic retinopathy. Jpn J Ophthalmol. 2006. 50:367–373.