Abstract
Purpose
To evaluate the protective effects of Epigallocatechin gallate (EGCG) against UV irradiation in cultured human retinal pigment epithelial (RPE) cells.
Methods
UV irradiation was produced by a UV lamp for 30 seconds with an irradiance of 3.3 mW/cm2. After 5 minutes and 1 hour, we administered different concentrations of EGCG (0, 5, 10, 15, 25, 50, 100 uM). The cell count was determined under a microscope using a counting chamber and the cell activity was evaluated by 3-(4,5-dimethylthiazol-2-yl)-2,5-diphenyl tetrazolium bromide (MTT) assay.
Results
The cell count of cultured human RPE cells after UV irradiation was markedly increased in the EGCG administration group, compared with the non-administrated group. The cell activity of the cultured human RPE cells after UV irradiation was markedly increased in the EGCG administration group and was increased in a dose-dependent way as determined by the MTT assay.
Light injury in daily life is caused by several mechanisms, especially short wavelength ultraviolet (UV) light. The ultraviolet region of the electromagnetic spectrum is divided into UVC (200-290 nm), UVB (290-320 nm) and UVA (320-400 nm).1 Ultraviolet light with wavelengths between 200 nm and 300 nm is absorbed by the cornea, and UV light between 300 nm and 400 nm penetrates the cornea and is absorbed by the lens. This absorption of UV light by the cornea and lens is helpful in protecting the retina.2,3 In addition, the retina itself has a defense mechanism. Cataract development increases with age and will reduce the amount of UV radiation to which the retina is exposed because of the opacification of the crystalline lens. However, depletion of the ozone layer in combination with the increases in outdoor recreation and the average span of life may result in an increase in the cumulative lifetime exposure of the retina to UV radiation.4 Also, the removal of the crystalline lens by cataract surgery may be associated with a substantial increase in the UV radiation that reaches the retina.5 A xanthophyll pigment in the macula plays the role of decreasing the exposure of photoreceptors and retinal pigment epithelial cells to blue light and ultraviolet light; it, also, inhibits the production of reactive oxygen species (ROS), such as hydrogen peroxide (H2O2), superoxide anion (O2-), singlet oxygen (1O2), and hydroxyl radical (HO-).6,7 Retinal pigment epithelial cells are protected from noxious and reactive chemicals through the actions of melanin and antioxidants, such as superoxide dismutase (SOD), catalase, glutathione peroxide (GSHPx), and vitamin E. However, retinal pigment epithelial cells may produce a large amount of oxygen radicals because they consume a lot of oxygen. These reactive oxygen species may peroxidize the unsaturated fatty acids in the cell membrane, damaging cellular the protein and DNA and causing oxidative cell injury. An investigation into the effects of ultraviolet light and apoptosis on retinal pigment epithelial cells has been performed, yielding various results. Yu et al. suggested that oxidative injury by UV irradiation is a cause of apoptosis in retinal pigment epithelial cells.8 Thus, the prospect of UV radiation playing a role in the progression of age-related diseases of the retina, such as age-related macular degeneration (ARMD), becomes a distinct possibility.9,10 In addition, the role of lipid peroxidation in retinal damage has been well established.11-13 As a related factor of ARMD and glaucoma, the damage due to light was discussed and researched, but it has not been clearly proven. ARMD is a leading cause of blindness in developed countries and will become more of a problem as life expectancy increases. Support for the involvement of oxidative stress in ARMD comes from the finding that daily administration of antioxidant vitamins and/or zinc significantly slows the rate of progression in patients from high-risk atrophic ARMD to neovascular ARMD.14 As a preventative measure, various attempts are being made to reinforce the eye's defenses against oxidative stress. Clinical epidemiological studies have shown that specific dietary habits are associated with a decreased incidence of advanced ARMD.15,16 Specific flavonoids were significantly more effective than either vitamins C or E in protecting human RPE cells from oxidative stress in vitro.17 Flavonoids belongs to a class of natural biological products that have evolved to protect plants from the oxidative damage induced by chronic exposure to UV light. Flavonoids have many physiological health benefits, including protection from cardiovascular disease and cancer, and most of these beneficial effects are thought to stem from their potent antioxidant and free radical-scavenging properties as well as their abilities to modulate many cellular enzyme functions.18 Specific flavonoids protected human RPE cells from oxidative-stress-induced death, with efficacies between 80% and 100% and potencies in the high-nanomolar and low-micromolar range. The toxicities of most of the effective flavonoids were low. The effective flavonoids included the dietary flavonoids fisetin, luteolin, quercetin, eriodictyol, baicalein, galangin, EGCG and the synthetic flavonoids (3, 6-dihydroxy flavonol, and 3, 7-dihydroxy flavonol). Several flavonoids can protect RPE cells even when they are added after the cells have been exposed to oxidative stress. The flavonoids acted through an intracellular route to block the accumulation of reactive oxygen species.17 However, few reports have been published on the protective effects of EGCG after UV irradiation on human retinal pigment epithelium.
The goal of this study was to evaluate the protective effects of Epigallocatechin gallate (EGCG) against UV irradiation in cultured human retinal pigment epithelial (RPE) cells. Five minutes and 1 hour after UV irradiation, we administered different concentration of EGCG (0, 5, 10, 15, 25, 50, 100 uM). Five minutes and 1 hour were chosen for the time of EGCG administration, after considering in vitro experiments of green tea absorption and retinal protective effects of EGCG. Ordinary persons take green tea inside of a building five minutes to 1 hour after UV exposure from the sun. After another 24-hour incubation period, the cell count was checked under microscope using a counting chamber and cell activity was evaluated by 3-(4,5-dimethylthiazol-2-yl)-2,5-diphenyl tetrazolium bromide (MTT) assay. So the aim of this study is to determine whether EGCG can protect human retinal pigment epithelial cells from UV-induced damage, and to develop a new in vitro model of retinal UV protection using Epigallocatechin gallate (EGCG).
The CRL-2302 (ARPE-19) (ATCC, USA) was used for this study. These human retinal pigment epithelial cells were cultured in Dulbecco's Modified Eagle's Medium (DMEM) (Sigma, St Louis, MO, USA), which is composed of 10% fetal bovine serum (FBS), penicillin (100 U/ml), streptomycin (100 u/ml), and nystatin (25 ug/ml). The cell line was maintained in a humidified incubator in an atmosphere of 95% air and 5% CO2 at 37℃ and the culture medium was changed every 48 hours. The cells were plated in T75 or T150 flasks (Falcon, NJ, USA) and allowed to grow until the cultures were confluent.
Human retinal pigment epithelial cells were irradiated for 30 seconds with an irradiance of 3.3 mW/cm2 after removal of serum-free growth medium. UV irradiance was modulated by adjusting lamp output and calibrated with a UV sensor on a UV lamp (FS-20 T12-UVB, National Biological Corp.). The UVB spectrum was between 280 nm and 320 nm with peak irradiance at 312 nm. The total exposure volume of UVB was 18 mJ/cm2.
Human retinal pigment epithelial cells were cultured for 1 day and the experiments were performed on 3 divided groups: the normal control group, which was not exposed to UV light (Group I); the second group, which was exposed to UV light without administration of EGCG (Sigma, St Louis, MO, USA) (Group II); and the third group, which was exposed to UV light with administration of EGCG (Group III). EGCG was dissolved in dimethyl sulfoxide (DMSO) (Sigma, St Louis, MO, USA) and added to the culture medium concomitantly with the TNF-a stimulus. Five minutes and 1 hour after UV irradiation, different concentration of EGCG (5, 10, 15, 25, 50, and 100 µM) were administered to Group III.
After 24 hours incubation, the cell count was determined under a microscope using a counting chamber, cell activity was evaluated by 3-(4,5-dimethylthiazol-2-yl)-2,5-diphenyl tetrazolium bromide (MTT) assay. Briefly, MTT is reduced to purple formazan in the mitochondria of living cells. A solubilizing liquid is added to dissolve the insoluble purple formazan product, forming a colored solution. MTT assay was carried out after using standard protocol. Optical density was measured at 570 nm using a spectrophotometer.
To increase the reliability of the data, all experiments were repeated 3 times and the average values were obtained. SPSS for Windows Ver. 10.1 was used to compute routine statistics. The data were analyzed for significance using repeated measures, followed by a Duncan's multiple range test of post hoc tests. The data were expressed as a mean percentage of the control value plus S.E.M. A p-value of <0.05 was considered significant.
Epigallocatechin gallate (EGCG) effectively protected cultured human retinal pigment epithelial (RPE) cells from oxidative-stress-induced cytotoxicity. Compared with the control group, which was not exposed to UV irradiation (Fig. 1A), exposure to UV irradiation without administration of EGCG showed significant cell loss (Fig. 1B). Another group to which 100 µM EGCG was administered 5 minutes (Fig. 1C) and 1 hour (Fig. 1D) after UV irradiation, experienced an increased cell count compared with that of the control group. The cell count of cultured human RPE cells after UV irradiation was markedly increased in the EGCG administration group, compared with the group that did not receive EGCG (Fig. 2). There was no significant relationship between the time to administration of EGCG and cell loss (P=0.279). Cell activity of the cultured human RPE cells after UV irradiation was markedly increased with EGCG administration, and it increased in a dose-dependent way, as determined by the MTT assay (Fig. 3). Within the group recieving EGCG, significant differences were shown between 5 µM (Mean=8.00±3.630) and 10 µM (M=25.83±5.399) and between 10 µM (M=25.83±5.399) and 15 µM (M=58.90±4.623) (P<0.001). There were no significant differences between 0 µM (M=0.00±0.000) and 5 µM (M=8.00±3.630) (P=0.260) among 15 µM (M=58.90±4.623), 25 µM (M=61.27±5.206), 50 µM (M=63.30±1.871), and 100 µM (M=68.10±5.229) (P=0.072). In comparison with the group that did not receive EGCG, cell activity was increased by approximately 43.9%, 68.1% respectively in 100 µM of the EGCG administration group. Moreover, post-treatment of RPE cells with EGCG (100 µM) after 1 hour showed significantly reduced cell deaths as a result of UV irradiation. There was a significant relationship between the time to administration of EGCG and cell activity (P=0.001).
Increasing interest in the health benefits of tea has led to the inclusion of tea extracts in dietary supplements and functional foods. While tea contains a number of bioactive chemicals, it is particularly rich in catechins. The main catechin group consists of eight polyphenolic flavonoid-type compounds: (+)-catechin (C), (-)-epicatechin (EC), (+)- gallocatechin (GC), (-)-epigallocatechin (EGC), (+)-catechin gallate (CG), (-)-epicatechin gallate (ECG), (+)-gallocatechin gallate (GCG), and (-)-epigallocatechin gallate (EGCG). (-)-Epigallocatechin gallate is the most abundant of the tea catechins and is thought to be responsible for the majority of the green tea extracts's biological activity, such as its anti-cancer and antioxidant properties.19,20 Although a number of in vitro and in vivo studies have shown EGCG acts as an antioxidant21-24 and an antiapoptotic agent,25 the actual mechanism of its action as an antioxidant remains unclear. Importantly, total plasma antioxidant activity has been shown to increase following significant intake of green tea26,27 in laboratory studies. Moreover, an increase in plasma catalase activity and a decrease in the nitric oxide levels28 occurred, suggesting that catechins have both direct antioxidant effects and indirect influences on increasing the activity of other antioxidants or enzymes. It has been suggested by Sutherland et al. that catechins like EGCG elicit antioxidant actions in a number of ways.29
EGCG promotes human keratinocyte survival and inhibits ultraviolet light-induced apoptosis by dual mechanisms, namely by phosphorylating Bad protein through Erk and Akt pathways, respectively, and by increasing the Bcl-2 to Bax ratio.30 Retinal pigment epithelial cells may produce a large amount of oxygen radicals because they consume a large amount of oxygen. These reactive oxygen species may peroxidize the unsaturated fatty acids in cell membranes, damaging the protein and DNA, thereby causing oxidative cell injury. An investigation about ultraviolet light and apoptosis in retinal pigment epithelial cells has been performed with various methods. Yu et al. suggested the possibility that an oxidative injury by UV irradiation is a cause of apoptosis in retinal pigment epithelial cells.8 Although research for suppression and protection against apoptosis of retinal pigmented epithelium is continuing, the correct mechanism has not been identified yet. ARMD is a leading cause for blindness in developed countries and will be more of a problem as life expectancy increases. Support for the involvement of oxidative stress in ARMD comes from the finding that daily administration of antioxidant vitamins and/or zinc significantly slowed the rate of progression in patients from high risk atrophic ARMD to neovascular ARMD.14 A number of age-related pathologies have been attributed to cumulative oxidative damage caused by ROS, such as nitric oxide (NO), hydrogen peroxide (H2O2), singlet oxygen, superoxide anion, and hydroxyl radicals. ROS arise as by-products of cellular metabolism or photochemical reactions.31,32 Furthermore, the oxygen supply to the photoreceptors via the choroidal and retinal vessels can cause an elevated oxygen tension in the retinal pigment epithelium and photoreceptors.33,34 Moreover, UV radiation can cause damage to DNA, leading to single-strand DNA breaks in the retina.4,35 EGCG attenuates the effect of an ischemia/ reperfusion insult to the intact retina, where oxidative stress is implicated,37 and to neurons in vitro by H2O2, an oxidant. It is generally thought that oxidative stress plays a part in the pathogenesis of glaucoma,37,38 initiated by ischemia to the optic nerve head components,39,40 because of a reduced optic nerve head blood supply.40 EGCG, besides being a powerful antioxidant,24 also has anti-inflammatory41,42 and vasodilator43 effects; both inflammation and vasoconstriction36,44 are strongly suspected of playing a part in the pathogenesis of glaucoma. Moreover, EGCG can intervene in other cellular mechanisms that are relevant to retinal ganglion cell loss in glaucoma, independently from its antioxidant properties. It is known that the oxidative damage to the retinal pigment epithelial cells play an important role in the development of ARMD and glaucoma.
In conclusion, the results of this study have shown that EGCG increases the cell count and cell activity after UV irradiation in cultured human retinal pigment epithelial cells; we can conclude that EGCG provides protective effects against UV damage in cultured human retinal pigment epithelial cells. Although the results show thatEGCG can protect human retinal pigment epithelial cells from UV induced damage, further in vivo and in vitro studies investigating areas such as mitochondrial damage, nucleic acid damage, phagocytotic activity after UV exposure in human retinal pigment epithelial cells will be required.
Figures and Tables
Fig. 1
RPE cells loss after UV irradiation A: Control without UV irradiation. B: After UV irradiation without administration of Epigallocatechin gallate (EGCG). C: 5 minutes after UV irradiation with administration of 100 µM EGCG. D: 1 hour after UV irradiation with administration of 100 µM EGCG. The photographs were taken by an inverted microscope. (×200)
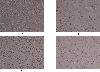
Fig. 2
RPE cells count after UV irradiation. The cell count of cultured human retinal pigment epithelial (RPE) cells after UV irradiation was markedly increased in the Epigallocatechin gallate (EGCG) administration group, compared with that of the group not receiving EGCG.. The control group did not receive UV irradiation. There was no significant relationship between the time of administrating EGCG and the loss of cells (P=0.279). A: 5 minutes after UV irradiation, B: 1 hour after UV irradiation.
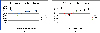
Fig. 3
RPE cell activity after UV irradiation. The cell activity of cultured human retinal pigment epithelial (RPE) cells after UV irradiation was markedly increased in the Epigallocatechin gallate (EGCG) administration group and increased in a dose-dependent way. Basis (0) was the group with UV irradiation without administration of EGCG. There was a significant relationship between the time of administration of EGCG and cell activities (P=0.001). A: 5 minutes after UV irradiation, B: 1 hour after UV irradiation.
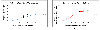
References
1. McCarty CA, Taylor HR. Recent developments in vision research : light damage in cataract. Invest Ophthalmol Vis Sci. 1996. 37:1720–1723.
2. Norren DV, Vos JJ. Spectral transmission of the human ocular media. Vision Res. 1974. 14:1237–1244.
3. Margrain TH, Boulton M, Marshall J, Sliney DH. Do blue light filters confer protection against age-related macular degeneration. Prog Retin Eye Res. 2004. 23:523–531.
4. Patton WP, Chakravarthy U, Davies RJ, Archer DB. Comet assay of UV-induced DNA damage in retinal pigment epithelial cells. Invest Ophthalmol Vis Sci. 1999. 40:3268–3275.
5. Mainster MA. The spectra, classification, and rationale of ultraviolet-protective intraocular lenses. Am J Ophthalmol. 1986. 102:727–732.
6. Sujak A, Gbrielska J, Grudzinski W, et al. Lutein and zeaxanthin as protectors of lipid membranes against oxidative damage: The structural aspects. Arch Biochem Biophys. 1997. 371:301–307.
7. Sujak A, Okulski W, Gruszecki WI. Organisation of xanthophyll pigment lutein and zeaxanthin in lipid membranes formed with dipalmitoylphosphatidylcholine. Biochim Biophys Acta. 2000. 1509:255–263.
8. Yu HG, Choe GS, Heo JW, et al. Oxidative Damage induced by UV in Cultured Retinal Pigment Epithelial Cells. J korean Ophthal Society. 2003. 44:2890–2895.
9. Sadun AC, Sadun AA, Sadun LA. Solar retinopathy. A biophysical analysis. Arch Ophthalmol. 1984. 102:1510–1512.
10. Young RW. Solar radiation and age-related macular degeneration. Surv Ophthalmol. 1988. 32:252–269.
11. Van Kuijk FJ. Effect of ultraviolet light on the eye: role of protective glasses. Environ Health Perspect. 1991. 96:177–184.
12. Noell WK, Walker VS, Kang BS, Berman S. Retinal damage by light in rats. Invest Ophthalmol. 1966. 5:450–473.
13. Beatty S, Koh H, Phil M, et al. The role of oxidative stress in the pathogenesis of age-related macular degeneration. Surv Ophthalmol. 2000. 45:115–134.
14. A randomized, placebo-controlled, clinical trial of high-dose supplementation with vitamins C and E, beta carotene, and zinc for age-related macular degeneration and vision loss. Arch Ophthalmol. 2001. 119:1417–1436.
15. Antioxidant status and neovascular age-related macular degeneration. Arch Ophthalmol. 1993. 111:104–109.
16. Seddon JM, Ajani UA, Sperduto RD, et al. Dietary carotenoids, vitamins A, C, and E, and advanced age-related macular degeneration. JAMA. 1995. 272:1413–1420.
17. Hanneken A, Lin FF, Johnson J, Maher P. Flavonoids Protect Human Retinal Pigment Epithelial Cells from Oxidative-Stress-Induced Death. Invest Ophthalmol Vis Sci. 2006. 47:3164–3177.
18. Middleton E Jr, Kandaswami C, Theoharides TC. The effects of plant flavonoids on mammalian cells: Implications for inflammation, heart disease and cancer. Pharmacol Rev. 2000. 52:673–751.
19. Wang YC, Bachrach U. The specific anti-cancer activity of green tea (-)-epigallocatechin-3-gallate (EGCG). Amino Acids. 2002. 22:131–143.
20. Kimura M, Umegaki K, Kasuya Y, et al. The relation between single/double or repeated tea catechin ingestions and plasma antioxidant activity in humans. Eur J Clin Nutr. 2002. 56:1186–1193.
21. Buttemeyer R, Philipp AW, Schlenzka L, et al. Epigallocatechin gallate can significantly decrease free oxygen radicals in the reperfusion injury in vivo. Transplant Proc. 2003. 35:3116–3120.
22. Morley N, Clifford T, Salter L, et al. The green tea polyphenol (-)-epigallocatechin gallate and green tea can protect human cellular DNA from ultraviolet and visible radiation-induced damage. Photodermatol Photoimmunol Photomed. 2005. 21:15–22.
23. Nagai K, Jiang MH, Hada J, et al. (-)-Epigallocatechin gallate protects against NO stress-induced neuronal damage after ischemia by acting as an anti-oxidant. Brain Res. 2002. 956:319–322.
24. Xie D, Liu G, Zhu G, et al. (-)-Epigallocatechin-3-gallate protects cultured spiral ganglion cells from H2O2-induced oxidizing damage. Acta Otolaryngol. 2004. 124:464–470.
25. Mandel S, Weinreb O, Amit T, Youdim MB. Cell signaling pathways in the neuroprotective actions of the green tea polyphenol (-)-pigallocatechin-3-gallate : implications for neurodegenerative diseases. J Neurochem. 2004. 88:1555–1569.
26. Skrzydlewska E, Ostrowska J, Farbiszewski R, Michalak K. Protective effect of green tea against lipid peroxidation in the rat liver, blood serum and the brain. Phytomedicine. 2002. 9:232–238.
27. Yokozawa T, Nakagawa T, Kitani K. Antioxidative activity of green tea polyphenol in cholesterol-fed rats. J Agric Food Chem. 2002. 50:3549–3552.
28. Negishi H, Xu JW, Ikeda K, et al. Black and green tea polyphenols attenuate blood pressure increases in stroke-prone spontaneously hypertensive rats. J Nutr. 2004. 134:38–42.
29. Sutherland BA, Rahman RMA, Appleton I. Mechanisms of action of green tea catechins, with a focus on ischemia-induced neurodegeneration. J Nutr Biochem. 2006. 17:291–306.
30. Chung JH, Han JH, Hwang EJ, et al. Dual mechanisms of green tea extract (EGCG) induced cell survival in human epidermal keratinocytes. FASEB J. 2003. 17:1913–1915.
31. Zhang B, Osborne NN. Oxidative-induced retinal degeneration is attenuated by Epigallocatechin gallate. Brain Res. 2006. 1124:176–187.
32. Pietta PG. Flavonoids as antioxidants. J Nat Prod. 2000. 63:1035–1042.
33. Weiter JJ. Miller D, editor. Phototoxic changes in the retina. Clinical Light Damage to the Eye. 1987. New York: Springer;79–125.
34. Bynoe LA, Gottsch JD, Pou S, Rosea GM. Light-dependent generation of superoxide from human erythrocytes. Photochem Photobiol. 1992. 56(3):353–356.
35. Kielbassa C, Roza L, Epe B. Wavelength dependence of oxidative DNA damage induced by UV and visible light. Carcinogenesis. 1997. 18(4):811–816.
36. Osborne NN, Casson RJ, Wood JP, et al. Retinal ischemia: mechanisms of damage and potential therapeutic strategies. Prog Retin Eye Res. 2004. 23:91–147.
37. Izzotti A, Bagnis A, Sacca SC. The role of oxidative stress in glaucoma. Mutat Res. 2006. 612:105–114.
38. Tezel G. Oxidative stress in glaucomatous neurodegeneration : Mechanisms and consequences. Prog Retin Eye Res. 2006. 25:490–513.
39. Osborne NN, Ugate M, Chao M, et al. Neuroprotection in relation to retinal ischemia and relevance to glaucoma. Surv Ophthalmol. 1999. 43:S102–S128.
40. Flammer J, Orgul S. Optic nerve blood-flow abnormalities in glaucoma. Prog Retin Eye Res. 1998. 17:267–289.
41. Chen JH, Tipoe GL, Liong EC, et al. Green tea polyphenols prevent toxin-induced hepatotoxicity in mice by down-regulating inducible nitric oxide-derived prooxidants. Am J Clin Nutr. 2004. 80:742–751.
42. Tedeschi E, Suzuki H, Menegazzi M. Antiinflammatory action of EGCG, the main component of green tea, through STAT-1 inhibition. Ann N Y Acad Sci. 2002. 973:435–437.
43. Lorenz M, Wessler S, Follmann E, et al. A constituent of green tea, epigallocatechin-3-gallate, activates endothelial nitric oxide synthase by a phosphatidylinositol-3-OH-kinase-, cAMP-dependent protein kinase-, and Akt-dependent pathway and leads to endothelial-dependent vasorelaxation. J Biol Chem. 2004. 279:6190–6195.
44. Delaney Y, Walshe TE, O'Brien C. Vasospasm in glaucoma: Clinical and laboratory aspects. Optom Vis Sci. 2006. 83:406–414.