Abstract
We investigated the fibrovascular ingrowth and fibrovascular tissue maturation of hydroxyapatite-coated, porous alumina sphere (Alumina sphere) in comparison with the hydroxyapatite sphere (HAp sphere) in rabbits. Alumina spheres and HAp spheres were implanted in the left orbits of 42 New Zealand white rabbits after enucleation. Fibrovascular ingrowth and maturation were graded from 1 to 5 at postoperative 1, 2, 3, 4, 8, 12 and 24 weeks. We defined 4 phases: postoperative 1-2 weeks as phase I, 3-4 weeks as phase II, 8-12 weeks as phase III, and 24 weeks as phase IV. The grade was analyzed at each phases. There was no significant difference in fibrovascular ingrowth and maturation between the two groups at all 4 phases, except phase II at which the Alumina sphere showed significantly lower maturation grade (p<0.05). We concluded that the Alumina sphere is an ideal orbital implant material and an ideal substitute for the HAp sphere in clinical practice.
Surgical anophthalmos by enucleation or evisceration due to eyeball rupture, absolute glaucoma, phthisis bulbi, or perforated corneal ulcer can bring out cosmetic and functional problems such as palpebral fissure asymmetry, enophthalmos, and prosthetic movement disorder. These patients can have lower quality of life and also difficulties in interpersonal relationships. In 1885, Mules first used glass sphere implants in the orbital cavity after evisceration, and various orbital implants such as tantalum, cartilage, bone, polyethylene, silicone, and dermal fat have been applicated.1 However, several problems such as infection, exposure, displacement of orbital implants, ptosis, enophthalmos, superior sulcus deformity and especially unsatisfactory motility of the prosthesis can occur.
Porous orbital implants have advantages of excellent motility and biocompatibility, low incidence of exposure, displacement and foreign body reaction. Fibrovascular ingrowth of the implant offers excellent movement and prevents infection, exposure and displacement. Recently, hydroxyapatite (HAp) is the most commonly used orbital implant material. HAp, hydrothermally synthesized sea coral, has multiple pores of 500 µm diameter which do not produce foreign body reaction in vivo and provide excellent fibrovascular ingrowth.2-3 HAp is the most popular orbital implant nowadays,4-5 since Perry used HAp in anophthalmic patients.6 Nevertheless, HAp has several disadvantages such as its gradual resorption over the years, inability to control appropriate pore size for better tissue ingrowth, tendency of exposure from abrasion of the overlying conjunctiva and Tenon's capsule, high cost, and the possible disruption of marine life ecosystems during the harvesting of coral.7
Alumina is a ceramic material that has been used in orthopedics and dentistry for a variety of prosthetic applications because of its excellent biocompatibility, fracture resistance, and lightweight. The HAp-coated ceramic sphere, invented in Korea as a new orbital implant, provides excellent biocompatibility and improves mechanical intensity.8
In this study, the HAp-coated alumina sphere was implanted in the orbit of rabbits and fibrovascular ingrowth was examined histopathologically and compared with the HAp sphere. The possibility of clinical application was evaluated.
Forty-two, female, New Zealand white rabbits averaging 3.7 kg in weight were housed in the animal care service of the School of Medicine, Yeungnam University, Taegu, Korea and were cared for according to the protocols set by the University's Animal Care Committee. After enucleation of the 42 left eyes of the 42 rabbits, the Alumina spheres were implanted in the orbit for the experimental group and the HAp spheres were used for the control group. Five rabbits were assigned to each experimental group to be harvested at 1, 2, 3, 4, 8, 12 and 24 weeks after implantation and one rabbit belonged to each control group to be harvested at corresponding intervals.
To investigate the consistency of the pore size and coated HAp thickness, we inspected one Alumina sphere and one HAp sphere under specular microscope. The pore size and coated thickness of HAp were consistent at 500-600 µm and the porosity was 90% in the Alumina sphere., compared to 300 µm and 60-70% in the case of the HAp sphere, respectively (Fig. 1). The pore size of the Alumina sphere was more consistent than that of the HAp sphere.
Under anesthesia induced with intramuscular injection of ketamine hydrochloride 1 mg per kg of rabbit, the conjunctiva and Tenon's capsule were incised in circular fashion along the limbus and dissected from the sclera. The four rectus muscles were tagged with 6-0 black silk and detached from its insertion. After the optic nerve was severed, the eyeball was extracted and meticulous hemostasis was done. The 12 mm-diameter orbital implant was inserted and the four4 rectus muscles were sutured together to the central portion firmly with 6-0 black silk over the implant. Tenon's capsule and the conjunctiva were sutured in continuous fashion. Daily antibiotic injection and dressing with antibiotic ophthalmic ointment were done postoperatively. Extraction of the orbital implant was performed according to the protocols and light microscopic examination was carried out.
Extracted Alumina tissue was washed in running water for 2 hours and dehydrated twice in 70, 80, 90, and 100% alcohol for 1 hour. This process was carried out in the refrigerator under thermostatic control at 4℃. After metathesis in propylene oxide for 24 hours twice, sedimentation was done in 1:1 and 2:1 compounds of epon mixture and propylene oxide for 24 hours. The vacuum operation was performed repeatedly (at least 5 times) until the epon mixture absorbed to the specimens completely and then the specimens were stored at 35℃, and 45℃ for 24 hours. All specimens were fixated at 60℃ for more than 4 days in 70% ethanol solution.
We used specific cutter (Accutom-50, Rotopol-35, Struers Co. Denmark) because the Alumina sphere is not weakened with mollification or decalcification. The 70 µm-thick sliced specimens were grinded to less than 50 µm thickness with diamond wheel.
The HAp sphere specimens were fixated in 10% formalin for 24 hours, decalcified, and embedded in paraffin. The Alumina sphere specimens were stained with toluidine blue and the HAp specimens stained with hematoxylin and eosin.
Fibrovascular ingrowth was graded from one to five, with each grade being defined as 20% depth of fibrovascular tissue penetration from the margin to center (Fig. 2).
As the granulation tissue became matured with fibrovascular ingrowth, it showed less tissue edema and inflammatory cells and more fibrosis with time. Fibrovascular tissue maturation was graded from 1-5 (Table 1). Grade 1: young granular tissue, demonstrating severe tissue edema, infiltration of acute inflammatory cells, barely developed capillaries and fibroblasts. Grade 2: well-developed capillaries, decreasing tissue edema with chronic inflammatory cell infiltration and increased number of fibroblasts. Grade 3: further development of capillaries and appearance of collagen fibers owing to the excessive development of fibroblasts. Grade 4: capillaries start to decrease, tissue edema is rarely seen, and collagen fiber development is remarkable. Grade 5: all the granulation tissue is substituted by the collagen fibers (Figs. 3, 4).
Four phases were defined according to the as following definition. Phase 1 was defined as postoperative one to two weeks, phase 2 as postoperative three to four weeks, phase 3 as postoperative eight to twelve weeks, and phase 4 as postoperative twenty-four weeks. Data was analyzed by Fisher's exact test with statistical significance set at p<0.05.
In Alumina sphere, fibrovascular proliferation appeared in all the 1-week specimens, average grade of fibrovascular ingrowth was grade 1 and maturation grade was also grade 1 (Fig. 5). At 2 weeks, specimens-showed grade 2 fibrovascular ingrowth and grade 1 fibrovascular maturation (Fig. 6). While rapid progress in fibrovascular ingrowth was seen in the 3-week specimens, showing grade 5 in two of the five specimens, fibrovascular maturation grade remained grade 1 in all specimens except one (Fig. 7). Uniform fibrovascular ingrowth through the center was seen in three of the five specimens, averaging grade 4 at 4 weeks. However, fibrovascular maturation grade was still grade 1. At 8 weeks, uniform fibrovascular ingrowth from the margin to the center was seen in all specimens and four of five had grade 3 fibrovascular maturation (collagen fibers started to appear). Average fibrovascular ingrowth was grade 5 at 12 weeks and fibrovascular maturation was grade 5 in three of the five specimens. Fibrovascular ingrowth and maturation had reached grade 5 in all the 24-week specimens (Table 2).
In the HAp sphere, the 1-week specimens showed grade 1 fibrovascular ingrowth and maturation (Fig. 8). At 2 weeks, rapid progress in fibrovascular ingrowth to grade 3 and maturation to grade 2.5 was seen (Fig 9). From 3 to 12 weeks, some specimens started to show fibrovascular maturation grade 5, and at 24 weeks all specimens were grade 5 maturation (Fig. 10, Table 3).
Fibrovascular ingrowth appeared in all 1-week specimens and average fibrovascular ingrowth was grade 1 in both groups. At 3 weeks, both groups showed more rapid fibrovascular ingrowth than at 2 weeks. Fibrovascular ingrowth was grade 4 in the Alumina sphere and grade 5 in the HAp sphere. All specimens of Alumina sphere of 8 to 24 weeks showed uniform fibrovascular ingrowth from the margin to the center (grade 5).
Grade 1 fibrovascular maturation was demonstrated in both the Alumina and HAp spheres at 1 week. Average fibrovascular maturation grade at 2 weeks was 1.4 in the Alumina sphere and 3 in the HAp sphere. All of the Alumina spheres at 3 weeks showed grade 1 fibrovascular maturation except 1 specimen, while the HAp sphere showed rapid fibrovascular maturation to grade 5. No change in fibrovascular maturation was seen in the Alumina sphere until 4 weeks, but rapid fibrovascular maturation averaging grade 4.4 was achieved by 12 weeks. Fibrovascular maturation was completed to grade 5 in the Alumina spheres at 24 weeks.
In phase I (acute phase), fibrovascular ingrowth and maturation differed among each specimen ranging from grade 1-3, but there was no statistically significant difference in grades between the experimental and control groups (p>0.05). In phase II (subacute phase), fibrovascular ingrowth progressed to grade 4-5, while there was a variable range of maturation grade from 1-4. No significant difference in fibrovascular ingrowth was present between the two groups, but the maturation grade was significantly lower in the Alumina sphere than in the HAp sphere (p<0.05). Phase III (chronic phase) specimens started to show completed fibrovascular ingrowth and maturation, and the extent was not significantly different between the two groups (p>0.05). Completion of the fibrovascular ingrowth and maturation was seen in all specimens of both groups in phase IV (residual phase).
Various orbital implants to maintain the proper orbital volume and to improve the motility of the prosthesis have been reported. The most commonly used orbital implants are natural HAp spheres, which have excellent fibrovascular ingrowth and biocompatibility. However, they are expensive, must be imported. and can disrupt the marine ecosystem. There is a consequent need to develop new synthetic materials to substitute for the HAp sphere.9-10 Various synthetic HAp spheres of more economic cost have been developed, but they were heavier and had lower pore size consistency and poorer intertrabecular connections than HAp spheres. They were reported to have no advantage over natural HAp spheres in fibrovascularization.11-12
Karesh and Dresner used high density porous polyethylene (Medpor®, Porex Surgical Inc., USA) as the orbital implant material, which displayed fibrovascularization similar to the HAp spheres, fewer complications such as migration and exposure, and is commonly used nowadays. Nevertheless, porous polyethylene is also a high-cost import like the HAp spheres.
Jordan et al13 have used spheric orbital implants (FCO Ophthalmics, Inc., France) made of alumina (aluminium oxide, Al2O3), which is commonly used as the bony substitute in the fields of orthopedics, dentistry, and otolaryngorhinology. They applied alumina in animal study and reported it to have similar biocompatibility and stability to the HAp spheres.13-14
The alumina, used as the component of the HAp-coated alumina spheres in this study, is distinguished from metal aluminium. Metal aluminium is the microelement existing in living organisms and the environment. A normal person digests about 2.5 mg of aluminium daily, absorbs it in the digestive system and excretes it through the kidneys. In patients with chronic renal failure, excessive aluminium can be accumulated in the brain and bone, causing problems. Ceramic alumina is chemically very stable, doesn't emit aluminium ions and doesn't cause problems in vivo.15 Alumina is the oxidated chemical of metal aluminium, has high inactivity and low tendency to cause inflammation in vivo, and is not biodegraded in vivo.16 Alumina readily binds protein molecules and forms a membrane to avoid the immune response. Labat et al17 reported that alumina has the superiority of more rapid proliferation of osteoblasts and fibroblasts than HAp spheres, and greater resistance from infection than porous polyethylenes.
Besides biocompatibility, the most important factor to be considered in selecting an orbital implant is rapid fibrovascularization to decrease the complications such as displacement, exposure and prolapse of the implants. The size and distribution of the pores in implants determine the rapidity of fibrovascular ingrowth. To a point, larger pore size, allows faster fibrovascularization, but at pore sizes above 700 µm, fibrovascular ingrowth is sluggish due to insufficient supporting structures, fragility increases due to decreased intensity and infection risk is high through the large pores.18-20 On the other hand, at pore sizes under 150 µm, the smooth surface decreases the abrasion of the overlying muscles, conjunctiva and Tenon's capsule, and exposure and prolapse of the implant occurs less frequently. There is the additional benefit of less opportunity of infection by AIDS or hepatitis virus because donor sclera or fascia is not necessary to cover the rough surface of the implants. However, small pore size can be a barrier to fibrovascularization.20
HAp has been known to promote tissue ingrowth and has been used as a bone graft substitutes. Many clinical reports support the advantages of HAp coatings including increased osteoconductive potential, and stronger bond to host bones. These benefits have led many orthopedic surgeons and dentists to use HAp-coated prostheses exclusively. However, the effect of HAp on fibroblasts, unlike that on osteoblasts, is not yet established. The HAp-coated porous alumina used in this study was manufactured to combine the merits of alumina and natural HAp, by maintaining the basic structure of alumina, yet add the excellent biocompatibility of HAp. Additional advantages of this implant are consistency in pore size and shape, excellent intertrabecular connections, high intensity, low body weight and manageableness. Besides, long-term follow up has demonstrated it to be economical and less biodegradable.20
Jordan et al21 reported that 12mm-diameter alumina sphere has more consistent pore size and weighs less than the HAp sphere of the same diameter. Magnetic resonance imaging, in vivo vascularization studies demonstrated enhancement of the implant to its center by 4 weeks after implantation in the rabbit. Histopathologically, fibrovascularization occurred uniformly throughout the implant by 4 weeks and cultured human fibroblasts grew more rapidly in the alumina sphere.12,22
The pore size and distribution of the implant are thought to be the important factors for fibrovascular ingrowth. In this study, the pore size of the Alumina sphere was more consistent and larger 500-600 µm in diameter than that of the HAp sphere at 300-500 µm. Fibrovascular tissue maturation was significantly slower in the alumina sphere than the HAp sphere at 3-4 weeks (p<0.05), but rapid maturation was achieved by 12 weeks. We think that the slow maturation at 3-4 weeks was possibly influenced by numerous variables such as surgical technique, and the structural and biochemical nature of HAp-coated alumina. This study had the limitation of the small sample size of the HAp sphere, and further research will therefore be required for clinical application.
Alumina sphere is an economically priced domestic product, and has the additional merits of high intensity, low body weight, and no biodegradability. The Alumina sphere demonstrated similar fibrovascular ingrowth to the HAp sphere, although slightly delayed, and had similar fibrovascular maturation to the HAp sphere. We suggest that the Alumina sphere has the potential to be an ideal orbital implant in clinical practice.
Figures and Tables
Fig. 1
Scanning electron micrograph of hydroxyapatite-coated alumina sphere and hydroxyapatite sphere.
(A) Hydroxyapatite-coated alumina sphere shows porosity of 90% and pore size of 500-600 µm.
(B) Hydroxyapatite sphere shows porosity of 60-70% and pore size of 300-500 µm.
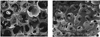
Fig. 3
Fibrovascular maturation grades of the hydroxyapatite-coated alumina sphere.
(A) Maturation grade 1 shows edematous young granulation tissue and little fibroblast proliferation (Toluidine blue stain, ×200).
(B) Maturation grade 2 shows moderate fibroblast proliferation and decreased edema. (Toluidine blue stain, ×400).
(C) Maturation grade 3 shows early stage of collagen fiber lay-down. (Toluidine blue stain, ×200).
(D) The granulation tissue is composed of collagen fibers in almost all areas in grade 5. (Toluidine blue stain, ×200).
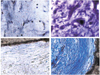
Fig. 4
Fibrovascular maturation grades of the hydroxyapatite sphere (hematoxylin-eosin stain, ×200).
(A) Maturation grade 2 shows fibroblast proliferation and capillary development in the edematous stroma. A few neutrophils are present.
(B) Collagen fiber lay-down is initiated in grade 3.
(C) Marked increase of collagen is noted in grade 4.
(D) In grade 5, there is compact growth of collagen fibers.
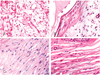
Fig. 5
Hydroxyapatite-coated alumina sphere at postoperative 1 week. (A) Fibrovascular ingrowth accounts for about 20% of the radius of implant in grade 1 (periphery of the implant, Toluidine blue stain, ×40). (B) Fibroblasts begin to proliferate in grade 1 (Toluidine blue stain, ×200).
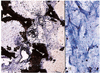
Fig. 6
Hydroxyapatite-coated alumina sphere at postoperative 2 weeks. (A) Fibrovascular ingrowth accounts for about 40% of the radius of implant in grade 2 (periphery of the implant, Toluidine blue stain, ×40). (B) Fibroblast proliferation is considered as grade 2 (Toluidine blue stain, ×200).
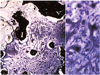
Fig. 7
Hydroxyapatite-coated alumina sphere at postoperative 3 weeks.
(A) Fibrovascular proliferation reaches the central portion of the implant (center of the implant, Toluidine blue stain, ×40).
(B) The implant shows initial stage of collagen lay-down, representing maturation grade 3 (Toluidine blue stain, ×200).
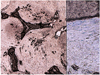
Fig. 8
Hydroxyapatite sphere at postoperative 1 week.
(A) Fibrovascular ingrowth accounts for about 20% of the radius of implant in grade 1 (periphery of the implant, Hematoxylin-eosin stain, ×40).
(B) Moderate fibroblast proliferation and considerable capillary proliferation indicates grade 2 (Hematoxylin-eosin stain, ×200).
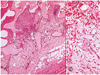
Fig. 9
Hydroxyapatite sphere at postoperative 2 weeks.
(A) Fibrovascular ingrowth accounts for about 60% of the radius of implant in grade 3 (periphery of the implant, Hematoxylin-eosin stain, ×40).
(B) The implant shows the initial stage of collagen fiber lay-down, representing grade 3 maturation (Hematoxylin-eosin stain, ×200).
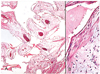
Fig. 10
Hydroxyapatite sphere at postoperative 3 weeks.
(A) The central portion of the implant is filled with fibrovascular proliferation, representing grade 5 (center of the implant, Hematoxylin-eosin stain, ×40).
(B) The granulation tissue is composed of collagen fibers in almost all areas in grade 5 (Hematoxylin-eosin stain, ×200).
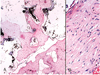
References
1. Durham DG. The new ocular implants. Am J Ophthalmol. 1949. 32:79–89.
2. Shields CL, Shields JA, De Potter P. Hydroxyapatite orbital implant after enucleation: experience with initial 100 consecutive cases. Arch Ophthalmol. 1992. 110:333–338.
3. Rubin PA, Nicaeus TE, Warner MA, Remulla HO. Effect of sucralfate and basic fibroblast growth factor on fibrovascular ingrowth into hydroxyapatite and porous polyethylene alloplastic implants using novel rabbit model. Ophthal Plast Reconstr Surg. 1997. 13:8–17.
4. Byrd WA. Coraline hydroxyapatite orbital implant. Ophthal Practice. 1991. 9:262–266.
5. Cutton JJ. Coraline hydroxyapatite as an ocular implant. Ophthalmology. 1991. 98:370–377.
6. Perry AR. Advances in enucleation. Ophthal Plast Reconstr Surg. 1991. 4:173–182.
7. Roy DM, Linnehan SK. Hydroxyapatite formed from coral skeletal carbonate by hydrothermal exchange. Nature. 1974. 247:220–222.
8. You CK, Oh SH, Kim SY. Hydroxyapatite coated porous alumina as a new orbital implant. Key Engineering Materials. 2003. 240–242. 563–566.
9. Hornblass A, Biesman B, Eviatar J. Current techniques of enucleation: a summary of 5439 intraorbital implants and a review of the literature. Ophthal Plast Reconstr Surg. 1995. 11:77–88.
10. Fricain J, Baquei C, Dupuy B. Resorption of corals implanted in diffusion chambers. J Mater Sci Mater Med. 1995. 6:680–684.
11. Mawn LA, Jordan DR, Gilberg S. Scanning electron microscopic examination of porous orbital implants. Can J Ophthalmol. 1998. 33:203–209.
12. Jordan DR, Mawn LA, Brownstein S, et al. The bioceramic orbital implant: a new generation of porous implants. Ophthal Plast Reconstr Surg. 2000. 16:347–355.
13. Jordan DR, Gilberg S, Mawn LA. The bioceramic orbital implant. Experience with 107 implants. Ophthal Plast Reconstr Surg. 2003. 19:128–135.
14. Bose MO, Avers RJ, Rieger MR, Duckworth JE. Submerged alumina dental root implants in humans: five-year evaluation. J Prosthet Dent. 1989. 61:594–601.
15. Sclatter C. Biomedical aspects of aluminum. Med Lav. 1992. 83:470–474.
16. Heimke G, Griss P, Jentschura G, Werner E. Bioinert and bioactive ceramics in orthopaedic surgery. Mechanical properties of biomaterials. 1980. Toronto: John Willey & Sons;207–215.
17. Labat B, Chamson A, Frey J. Effects of γ-alumina and hydroxyapatite coatings on the growth and metabolism of human osteoblasts. J Biomed Mater Res. 1995. 29:1397–1401.
18. Rubin PA, Popham JK, Bilyk JR, Shore JW. Comparison of fibrovascular ingrowth into hydroxyapatite and porous polyethylene orbital implants. Ophthal Plast Reconstr Surg. 1994. 10:96–103.
19. Rubin PA, Bilyk JR, Shore JW. Orbital reconstruction using porous polyethylene sheets. Ophthalmology. 1994. 101:1697–1708.
20. Seong YS, Lee SY, Kim SJ. Morphological study of a new orbital implant: hydroxyapatite-coated porous alumina in rabbit. J Korean Ophthalmol Soc. 2001. 42:1354–1361.
21. Jordan DR, Brownstein S, Gilberg S, et al. Hydroxyapatite and calcium phosphate coatings on aluminium oxide orbital implants. Can J Ophthalmol. 2001. 37:7–13.
22. Mawn LA, Jordan DR, Gilberg S. Scanning electron microscopic examination of porous orbital implants. Can J Ophthalmol. 1998. 33:203–209.