Abstract
To investigate the effect of basic Fibroblast Growth Factor (bFGF) on fibrovascular ingrowth into porous polyethylene orbital implants (Medpor®) and to investigate any differences according to the method of administration. For the treated groups, after evisceration and Medpor® implantation, bFGF was administered by soaking Medpor® in the bFGF solution, and/or by injecting bFGF into the Medpor® 1 week after the operation. Implants were removed 4 weeks after the operation and examined for the degrees of fibrovascular ingrowth by light microscopy. The percentages of the cross-sectional area of the implant occupied by fibrovascular ingrowth and the numbers of proliferated vessels were significantly higher in the bFGF-treated groups (Mann Whitney test, p<0.05). Administration routes had no effect on the degree of fibrovascular ingrowth (Kruskal-Wallis test, p>0.05). bFGF promoted fibrovascular ingrowth into porous polyethylene orbital implants regardless of the route of administration. Therefore, bFGF might be helpful to prevent complications such as implant exposure.
In 1884, a glass sphere was first introduced by Mules to replace orbital volume after eyeball removal. In 1988 Perry1 introduced hydroxyapatite, a porous orbital implant, and in 1994 Karesh and Dresner2 introduced a porous polyethylene orbital implant. Porous orbital implants can provide excellent ocular motility owing to direct attachment of the extraocular muscle to the implant. Porous orbital implants can also be positioned securely in the orbit due to fibrovascular ingrowth into multiple small pores in the implant. At present the porous orbital implant is widely used because of its good ocular motility and low rate of complications, for example, implant extrusion or migration.2-4
The most significant complication of porous orbital implants is implant exposure.5-7 Implant exposure must be treated because of increased ocular discharge and the possibility of infection, but it is not always easy to treat adequately.5-7
Many factors are thought to cause porous orbital implant exposure. One of the important factors is delayed fibrovascular ingrowth into the porous orbital implant. Much evidence supports this view; exposures are significantly higher in cases of evisceration than in cases of enucleation.8 In cases of evisceration, the exposure rate can be lowered by posterior sclerotomies and a large scleral window.9,10 Histopathologic findings of exposed implants showed fibrovascularization limited to the periphery with moderate inflammatory reaction.8,11,12 Therefore, promoting fibrovascular ingrowth into implants will reduce the exposure rate of porous orbital implants.
On the other hand, perhaps the most promising merit of the porous orbital implant is that maximal ocular motility can be gained by inserting a motility coupling post into the implant and connecting it with prosthesis. To insert the motility coupling post safely without any complication, its placement should be performed after central vascularization of the porous orbital implant has occurred.13 Therefore, the rapid completion of fibrovascular ingrowth will permit anophthalmic patients an early rehabilitation.
There have been many attempts to promote fibrovascular ingrowth into orbital implants using growth factors.14-19 Some of these trials failed to show an effect of the growth factor on fibrovascular ingrowth. Other investigations demonstrated this effect but were limited to in-vitro systems.16,17,20 Yet other studies demonstrated this effect in in-vivo animal models but the routes of administration used are not practical methods in actual clinical situations.18,19
The purpose of the present study was to investigate the effect of basic Fibroblast Growth Factor (bFGF) on fibrovascular ingrowth into porous polyethylene orbital implants when bFGF is administered to the implant by soaking the implant in a bFGF solution or by injecting bFGF into the implant, both of which can easily be performed in the clinical setting. We also purposed to determine which method of administration is more effective at promoting fibrovascular ingrowth.
Thirty New Zealand white rabbits weighing 2.0~2.5 kg each were divided into five groups (six rabbits/group). In group 1, bFGF was administrated by soaking an implant in bFGF solution before implantation; in group 2, bFGF was administered by injecting it 1 week after implantation; in group 3, by soaking an implant in bFGF solution before implantation and injecting bFGF 1 week later; in group 4, phosphate buffered solution (PBS), as a control, was administrated by soaking an implant in PBS before implantation; and in group 5, the implant was soaked in PBS before implantation and PBS was injected 1 week later. Each rabbit underwent evisceration and implantation of a 14mm-diameter porous polyethylene orbital implant (Medpor®) into the right orbit.
Rabbits were anesthetized with an intramuscular injection of ketamine 25 mg/kg and xylazine 6 mg/kg and sterilized around the right eyeball with 5% betadine solution. A conjunctival incision was made along the limbus and the Tenon's layer was dissected from the eyeball until the insertions of the rectus muscles were exposed. The corneal midline was incised with a No-11 blade to bisect the whole cornea. All the eyeball contents were removed using an evisceration spoon. To minimize the effect of various growth factors in autogenous blood, every effort was made to avoid bleeding during the operative procedure and if observed, rapid compression or cauterization was applied to achieve complete hemostasis.
In group 1, a porous orbital implant was placed in a 10cc-syringe filled with bFGF solution diluted in PBS to the concentration of 2.5 µg/ml. Repetitive pulling and releasing of the piston made the bFGF solution infiltrate well into the porous orbital implant. This bFGF-soaked implant was inserted into the eviscerated scleral shell. The cornea was sutured with a 6-0 polyglactin interrupted suture. The Tenon's layer and conjunctiva were also sutured carefully with a 6-0 polyglactin interrupted suture whilst avoiding excess tension at the wound site. Tarsorrhaphy was applied using 4-0 black silk. In group 2, a porous orbital implant was placed in a 10cc-syringe filled with PBS solution. This PBS-soaked implant was then inserted into the eviscerated scleral shell. The cornea, Tenon's layer and conjunctiva were sutured in the same manner as in group 1. One week after the operation, 0.2 ml of bFGF solution (2.5 µg/ml) was injected into the center of the implant using a 26 gauge needle after anesthesia and sterilization as described for implant insertion. In group 3, a porous orbital implant was soaked in bFGF solution and inserted into the scleral shell in the same manner as in group 1. One week after the operation, 0.2 ml of bFGF solution (2.5 µg/ml) was injected into the center of the implant in the same manner as in group 2. In group 4, a porous orbital implant was soaked in PBS solution and inserted into the scleral shell. Finally, in group 5, a porous orbital implant was soaked in PBS solution, inserted into the scleral shell and 1 week after the operation, 0.2 ml of PBS solution was injected into the center of the implant.
A pilot study was conducted to determine the optimal time for examining the degree of fibrovascular ingrowth into the porous orbital implants. Twenty white rabbits were grouped (4/group) into the five classes and received the operation as described above. Four rabbits were sacrificed at 1, 2, 4, and 6 weeks after the operation respectively in each group. Tissue specimens of the removed implants were examined under a light microscope. Fibrovascular ingrowth was up to 58~98% (defined below) at 4 weeks after the operation and up to 80~100% at 6 weeks after the operation in all five groups. Therefore, we decided to examine tissue specimens 4 weeks after the operation.
At 4 weeks after the operation, the rabbits from all five groups were anesthetized as described for implant insertion. The orbital implant and surrounding sclera as well as the optic nerve were removed and fixed in 10% formalin solution. The optic nerve was left as long as possible during removal so that the direction of the implant could be determined. Formalin-fixed implants were bisected with a cutting plane just beside the optic nerve. After decalcification, the bisected implant was imbedded in paraffin and cut into 4 µm-thicknesses trying to pass through the optic nerve to ensure that sectioned tissue specimens represented the mid-plane of the implant.
Fibrovascular ingrowth margins were examined in H&E-stained pathologic specimens under a light microscope (Fig. 1A, B). A connective tissue enmeshed with spindle-shaped fibroblasts was considered as an ingrown fibrovascular tissue. A myxoid area with round-shaped cells and rare spindle-shaped fibroblasts, which was usually observed in the most inner area of the implant was not interpreted as an ingrown fibrovascular tissue.20 Specimens were then scanned and converted into high resolution digital images on a computer. The fibrovascular ingrowth margin was marked in scanned images. The percentage of the area occupied by fibrovascular ingrowth was calculated using Sigma Scan Pro® (Version 5.0, SPSS Inc.1999) software (Fig. 2). To assess the degree of vascularization within the proliferated fibrovascular tissue, proliferated vessels were counted after immunohistochemical staining for vascular endothelial cells using CD31 antibodies. Proliferated vessels observed within one 100x field under a light microscope were counted in five randomly-selected areas of fibrovascular ingrowth (Fig. 3A, B). The largest and smallest counts were abandoned and the remaining three median numbers were averaged.
Fibrovascular ingrowth area (%) and the number of proliferated vessels were compared statistically between the treated and control groups using the Kruskal-Wallis test and Mann-Whitney U test.
In all the five groups, no rabbits showed any evidence of implant exposure or infection at the time of implant removal. Fibrovascular ingrowth (%) values for all groups are summarized in Table 1, and were higher in groups 1, 2 and 3 than in groups 4 and 5 with statistical significance (Mann Whitney test, p<0.01). There were no significant differences among groups 1, 2 and 3 (Kruskal-Wallis test, p>0.05) or between groups 4 and 5 (Mann Whitney test, p>0.05).
The number of proliferated vessels in the fibrovascular ingrowth area for each group is summarized in Table 2. This number was higher in groups 1, 2 and 3 than that in group 4 without statistical significance (Mann Whitney test, p>0.05), and higher than that in group 5 with statistical significance (Mann Whitney test, p<0.05). No significant differences in this respect were found among groups 1, 2 and 3 (Kruskal-Wallis test, p>0.05) or between groups 4 and 5 (Mann Whitney test, p>0.05).
This study shows that bFGF promotes fibrovascular ingrowth into porous polyethylene orbital implants when an orbital implant is put in a bFGF solution. bFGF is a kind of protein that is deposited in the extracellular matrix.21 It has the ability to promote proliferation, migration and the angiogenesis of vascular endothelial cells in the chorioallantoic membrane of the developing chick.22 Moreover, bFGF promotes the proliferation of mesoderm-originated cells and acts as a potent mitogen in capillary endothelial cells.23 bFGF lacks the signal peptide sequence for transport into the endoplasmic reticulum and is directly released through mechanically induced membrane disruptions of endothelial cells.24 Increased levels of bFGF in vitreous specimens have been documented in patients with active proliferative retinopathy.25 It is also known as a potential mediator of intraocular angiogenesis.
There have been many reports about trials to promote fibrovascular ingrowth into orbital implants using growth factors.16,17,19,20 However, some of these trials have failed to show a positive effect of the growth factor on fibrovascular ingrowth,17,20 whereas other investigations demonstrated a positive effect but their work was limited to in-vitro systems.16,20 Yet others demonstrated positive effects in in-vivo animal models, but the routes of administration are unsuitable for clinical application.19
Nicaeus et al16 reported that a bFGF-treated porous polyethylene orbital implant placed in tissue culture wells plated with endothelial cells yields significantly increased endothelial cell proliferation. On the other hand, Rubin et al20 reported that fibrovascular ingrowth into porous polyethylene implants in rabbits is not affected by the addition of bFGF 3 and 6 weeks postoperatively; this negative result may have been due to the low concentration of bFGF used (0.06 ng/ml). Furthermore, in their study implants were fully penetrated by fibrovascular tissue after 3 and 6 weeks regardless of bFGF treatment. It seemed near impossible to measure the effect of bFGF accurately in their animal model since the velocity of ingrowth of fibrovascular tissue was too rapid even in the control group for the effect of bFGF to be evident in the treated group.
Woo et al17 also reported that bFGF has no effect on fibrovascular ingrowth when a bFGF-soaked porous polyethylene orbital implant of 12 mm diameter is implanted into the orbit of a rabbit. In their study the extent of fibrovascular ingrowth was about 40% at 1 week and 50~ 80% at 2 weeks postoperatively. Thus, it seems difficult to demonstrate the effect of bFGF in an animal model given such a rapid ingrowth rate if the effect of bFGF is small.
Recently, Kim et al19 reported that bFGF promotes fibrovascular ingrowth into porous polyethylene orbital implants after 4 weeks but not after 1 and 2 weeks postoperatively. They soaked the implant in bFGF-mixed hydron (polyhydroxyethylmethacrylate) solution for 5 minutes and then dried it for 8 minutes. However, this route of administration is not practical to use in the clinical situation since it needs a meticulous pretreatment procedure lasting over 8 hours.
Considering previous reports, and assuming that one possible cause of the failure to show a positive effect of the growth factor on fibrovascular ingrowth was the very rapid tissue-ingrowth in the control group of the rabbit model, we chose evisceration as a method of eyeball removal instead of enucleation because the rate of fibrovascular ingrowth into the orbital implant seems to be reduced due to implant wrapping with a scleral shell. We also chose soaking or injection as routes of bFGF administration because they are easily used in the clinical situation. During evisceration, no posterior sclerotomy was made to avoid contact between the implant and orbital vessels from which fibrovascular ingrowth originates. We used a larger implant (14 mm-diameter) than those used in previous reports to extend the time required for the fibrovascular ingrowth to reach the center of the implant. This large implant insertion was made possible by not excising the cornea.
No previous report has advised on adequate bFGF concentrations for porous orbital implants. In the present study, the bFGF concentration was decided at 2.5 µg/ml considering that; the 0.06 ng/ml bFGF concentration used by Rubin et al.20 was too low to produce an effect; the bFGF concentrations used in an experimental bone-graft incorporation rat model were in the 0.1~62.5 µg/ml range,26-28 and the bFGF concentration used in an experimental skin graft model to promote angiogenesis was 500~5000 ng.29
To assess the rate of fibrovascular ingrowth into a porous polyethylene orbital implant, the majority of previous reports averaged ingrowth rates at four different points.30,31 They divided the cross-sectional area of the implant into four zones (from surface to the center) and estimated the ingrowth rate using; zone 1: 44%, zone 2: 75%, zone 3: 94%, and zone 4: 100%. An averaged estimated growth rate from four 90°- apart points was regarded as a representative growth rate. In the present study, we converted the cross-sectioned surface of implants to a digital image and calculated the percentage area penetrated by fibrovascular tissue using Sigmascan Pro®. This software counts the number of pixels included in the prescribed territory on digital images, and provides a more accurate determination of the fibrovascular ingrowth area.
We confirmed that bFGF promotes fibrovascular ingrowth into porous polyethylene orbital implants; this ingrowth rate was higher in the bFGF-treated group than in the control group; and the number of proliferated vessels in the fibrovascular ingrowth area was higher in bFGF-treated groups than in the control groups. At first, rabbits treated with bFGF using both soaking and injection (group 3) were expected to show the greatest bFGF effect, but we were unable to find any significant differences of the ingrowth rates among the three bFGF-treated groups with various routes of administration (Table 1, 2). It seems that the additional injection of bFGF has no or a minimal additive effect on fibrovascular ingrowth. At this time we are unable to suggest why it has no effect on ingrowth.
In conclusion, bFGF promoted fibrovascular ingrowth in bFGF-soaked and/or bFGF-injected porous polyethylene orbital implants. When a porous polyethylene orbital implant is inserted, a concurrent bFGF administration can reduce implant exposure from delayed fibrovascular ingrowth, especially in patients who previously received radiation therapy. Furthermore, rapid fibrovascular ingrowth makes it possible to insert a motility coupling post in the early stage to achieve maximal ocular motility and shorten the rehabilitation period.
Figures and Tables
Fig. 1
Determination of fibrovascular ingrowth margins in H&E-stained pathologic specimens under a light microscope. (A) Low magnification view (10×) of the cross section of the implant. (B) Higher magnification view (100×) of the box area in Figure 1A. A connective tissue enmeshed with spindle-shaped fibroblasts can be seen on the left side of the picture. Its inner border was drawn with a dotted line. An inner myxoid area with round-shaped cells and rare spindle-shaped fibroblasts (the area right to the dotted line) was not interpreted as an ingrown fibrovascular tissue.
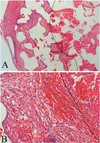
Fig. 3
Counting of proliferated vessels. (A) After immunohistochemical staining for vascular endothelial cells using CD31 antibodies, five areas were randomly selected for counting. (B) Proliferated vessels were evident in the proliferated fibrous tissue by endothelial cells staining with CD31 antibodies (×100). Vessels within one examining field at 100× were counted.
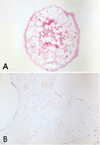
Table 1
Degree of fibrovascular ingrowth into the porous polyethylene orbital implants 4 weeks after implantation
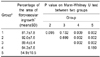
*Group 1: soaking of implant in bFGF before implantation
Group 2: soaking of implant in PBS before implantation and injecting bFGF 1 week later
Group 3: soaking of implant in bFGF before implantation and injecting bFGF 1 week later
Group 4: soaking of implant in PBS before implantation
Group 5: soaking of implant in PBS before implantation and injecting PBS 1 week later
†Kruskal-Wallis test, p<0.001
References
1. Perry AC. Integrated orbital implants. Adv Ophthalmic Plast Reconstr Surg. 1988. 8:75–81.
2. Karesh JW, Dresner SC. High-density porous polyethylene(Medpor) as a successful anophthalmic socket implant. Ophthalmology. 1994. 101:1688–1696.
3. Potter PD, Shields CL, Shields JA, Singh AD. Use of the hydroxyapatite ocular implant in the pediatric population. Arch Ophthalmol. 1994. 112:208–212.
4. Karcioglu ZA, Al-Mesfer SA, Mullaney PB. Porous polyethylene orbital implant in patients with retinoblastoma. Ophthalmology. 1998. 105:1311–1316.
5. Jordan DR, Brownstein S, Jolly SS. Abscessed hydroxyapatite orbital implants. A report of two cases. Ophthalmology. 1996. 103:1784–1787.
6. Karcioglu ZA. Actinomyces infection in porous polyethylene orbital implant. Graefes Arch Clin Exp Ophthalmol. 1997. 235:448–451.
7. Goldberg RA, Dresner SC, Braslow RA, et al. Animal model of porous polyethylene orbital implants. Ophthalmic Plast Reconstr Surg. 1994. 10:104–109.
8. Remulla HD, Rubin PA, Shore JW, et al. Complications of porous spherical orbital implants. Ophthalmology. 1995. 102:586–593.
9. Kostick DA, Linberg JV. Evisceration with hydroxyapatite implant. Surgical technique and review of 31 case reports. Ophthalmology. 1995. 102:1542–1549.
10. Yang JG, Khwarg SI, Wee WR, et al. Hydroxyapatite implantation with scleral quadrisection after evisceration. Ophthalmic Surg Lasers. 1997. 28:915–919.
11. Goldberg RA, Holds JB, Ebrahimpour J. Exposed hydroxyapatite orbital implants. Report of six cases. Ophthalmology. 1992. 99:831–836.
12. Sekundo W, Siefert P. Hydroxyapatite orbital implant. A light- and electron-microscopic and immunohistochemical study. Ophthalmology. 1998. 105:539–543.
13. Klapper SR, Jordan DR, Ells A, Grahovac S. Hydroxyapatite orbital implant vascularization assessed by magnetic resonance imaging. Ophthal Plast Reconstr Surg. 2003. 19:46–52.
14. Holck DE, Dutton JJ, Proia A, et al. Rate of vascularization of coralline hydroxyapatite spherical implants pretreated with saline/gentamicin, rTGF-2, and autogenous plasma. Ophthalmic Plast Reconstr Surg. 1998. 14:73–80.
15. Sires BS, Holds JB, Kincaid MC, Reddi AH. Osteogenin-enhanced bone-specific differentiation in hydroxyapatite orbital implants. Ophthalmic Plast Reconstr Surg. 1997. 13:244–251.
16. Nicaeus TE, Tolentino MJ, Adamis AP, Rubin PAD. Sucralfate and basic fibroblast growth factor promote endothelial cell proliferation around porous alloplastic implants in vitro. Ophthalmic Plast Reconstr Surg. 1996. 12:235–239.
17. Woo KI, Kim JM, Chang HR, et al. The Effect of Growth Factors and Surgical Procedures on Fibrovascular Ingrowth into Anophthalmic Socket Implant of Porous Polyethylene. J Korean Ophthalmol Soc. 2000. 41:2727–2731.
18. Choi YS, Yim HB, Choi WC. The Effect of Vascular Endothelial Growth Factor on Vascularization in Porous Polyethylene Orbital Implant (Medpor®). J Korean Ophthalmol Soc. 2003. 44:1180–1187.
19. Kim KR, Jung CS, Chung SK. The Effect of Basic Fibroblast Growth Factor on the Vascularization of Porous Polyethylene Orbital Implant (Medpor®). J Korean Ophthalmol Soc. 2002. 43:2258–2264.
20. Rubin PA, Nicaeus TE, Warner MA, Remulla HD. Effect of sucralfate and basic fibroblast growth factor on fibrovascular ingrowth into hydroxyapatite and porous polyethylene alloplastic implants using a novel rabbit model. Ophthalmic Plast Reconstr Surg. 1997. 13:8–17.
21. Vlodavsky I, Folkman J, Sullivan R, et al. Endothelial cell-derived basic fibroblast growth factor: Synthesis and deposition into subendothelial extracellular matrix. Pro Natl Acad Sci USA. 1987. 84:2292–2296.
22. Shing Y, Folkman J, Haudenschild C, et al. Angiogenesis is stimulated by a tumor-derived endothelial cell growth factor. J Cell Biochem. 1985. 29:275–287.
23. Abraham JA, Mergia A, Whang JL, et al. Nucleotide sequence of a bovine clone encoding the angiogenic protein, basic fibroblast growth factor. Science. 1986. 233:545–548.
24. McNeil PL, Muthukrishnan L, Warder E, D'Amore PA. Growth factors are released by mechanically wounded endothelial cells. J Cell Biol. 1989. 109:811–822.
25. Sivalingam A, Kenney J, Brown GC, et al. Basic fibroblast growth factor levels in the vitreous of patients with proliferative diabetic retinopathy. Arch Ophthalmol. 1990. 108:869–872.
26. Wang JS, Aspenberg P. Basic fibroblast growth factor promotes bone ingrowth in porous hydroxyapatite. Clin Orthop. 1996. 333:252–260.
27. Wang JS, Aspenberg P. Basic fibroblast growth factor increases allograft incorporation. Bone chamber study in rats. Acta Orthop Scand. 1994. 65:27–31.
28. Wang JS, Aspenberg P. Basic fibroblast growth factor infused at different times during bone graft incorporation. Titanium chamber study in rats. Acta Orthop Scand. 1996. 67:229–236.
29. Lees VC, Fan TPD. A freeze-injured skin graft model for the quantitative study of basic fibroblast growth factor and other promoters of angiogenesis in wound healing. Br J Ophthalmol. 1994. 47:349–359.
30. Rubin PA, Popham JK, Bilyk JR, Shore JW. Comparison of fibrovascular ingrowth into hydroxyapatite and porous polyethylene orbital implants. Ophthalmic Plast Reconstr Surg. 1994. 10:96–103.
31. Bigham WJ, Stanley P, Cahill JM Jr, et al. Fibrovascular ingrowth in porous ocular implants: the effect of material composition, porosity, growth factors, and coatings. Ophthalmic Plast Reconstr Surg. 1999. 15:317–325.