Abstract
The goal of this study was to evaluate the function of hyaluronic acid (HA) on the active oxygen release from polymorphonuclear leukocytes (PMNs) and the protective effect of bovine corneal endothelial cells (BCEC) from activated PMNs. We used HA with three different molecular weights (MW 700,000, 2,000,000, and 4,000,000) and five different concentrations (0, 0.1, 1, 2, and 3 mg/ml). We evaluated the amount of released superoxide from activated PMNs by using dismutase-inhibitable ferricytochrome C reduction. To compare the property and protective effect of HA with those of other viscoelastic substances, we used the same concentration of methylcellulose. HA suppressed superoxide release from PMNs and protected BCEC from activated PMNs in a dose-dependent, rather than a molecular weight-dependent, manner. The effect of HA reached almost a plateau at concentration above 2 mg/ml. However, methylcellulose, another viscoelastic substance, showed a similar effect. Therefore, it seems that the suppression of superoxide released from PMNs is not a property that is unique to HA, but is a general property of viscoelastic substances. Our results indicate that the action mechanism of HA proceeds not only through cell surface HA-receptor. We think that HA also acts as a physical barrier and/or a scavenger of superoxide.
Viscoelastic substances are now widely used in the field of ophthalmologic surgery to maintain anterior chamber depth during surgery and to protect corneal endothelial cells from mechanical injuries.1 The cause of corneal endothelial toxicity was thought to be oxygen free radicals from polymorphonuclear leukocytes (PMNs). Among the various kinds of viscoelastics, hyaluronic acids (HAs) of various molecular weights have been most frequently used. However, not only mechanical contact but also inflammation causes tissue injury by surgery. Inflammation during intraocular surgery may cause cataracts, corneal opacity and retinal edema. Some investigators2 have reported that by using HA instead of an air bubble during glaucoma filtering surgery, they could decrease the rate of cataract development after operation.
HA is a disaccharide polymer of glucuronic acid and N-acetylglucosamine. Because of its mechanical protective effect, it is used in cataract, glaucoma and ocular penetrating injury operations.1 Furthermore, it is known that HA inhibits the attachment of leukocytes to the intraocular lens and inhibits phagocytosis of macrophages.3,4 However, there is still considerable debate over its actions. Suzuki and Yamaguchi3 reported that HA suppressed active oxygen release and phagocytosis of macrophage in a molecular-dependent manner and Koich et al.4 reported that HA inhibited chemotaxis and phagocytosis of macrophage but not active oxygen release. However, there have been few studies regarding the possible properties as protectors of the corneal endothelium from the oxidative damage caused by free radicals.
The purpose of this study was to determine whether HA actually inhibits active-oxygen release from the leukocyte and if this effect is moleculardependent or dose-dependent. We also wanted to determine whether HA would protect cultured corneal endothelial cells from activated PMN by measuring the viability of bovine corneal endothelial cells (BCEC) in MTT assay.
Human peripheral blood was obtained in 20 ml quantities with a 20 U/ml heparin-coated syringe and then mixed with Hank's balanced salt solution (HBSS, GIBCO). Neutrophils were separated from red cells and other lymphocytes using Ficoll-Paque (Pharmacia & Upjohn, Biotech AB Uppsala, Sweden). After that, neutrophils were mixed with dextran (SIGMA, St. Louis, Mo, USA) and then preserved in an ice bag for 45 minutes. The upper fluid was removed and the remaining red blood cells were destroyed with hypotonic solution (0.2% NaCl). Purified neutrophils were obtained by centrifugation of the solution at 4℃ and 1000 rpm for 10 minutes with 10% fetal bovine serum (FBS, GIBCO).
Using a 96-well plate, about 1 × 106 of the purified neutrophils were placed into each well along with 50 ul of HBSS. Into each well was then placed one of the three types of HA, molecular weight (MW) 700,000 (Viscoat®, Alcon, USA), MW 2,000,000 (Healon®, Pharmacia & Upjohn, Sweden), and MW 4,000,000 (Healon GV®, Pharmacia & Upjohn, Sweden) at four different concentrations (0.1, 1, 2, 3 mg/ml). We also employed two types of control groups: viscoelastic free wells and 2% hydroxymethylcellulose (Ocucoat®, Storz, USA) wells. The 2% hydroxymethylcellulose wells did not contain HA but had similar viscoelasticity to the HA wells. Twenty microliters of a 10 ng/ml phorbol myristate acetate solution (PMA, SIGMA), a chemoactive peptide for neutrophils, was applied into each well. Fifty microliters of cytochrome C (SIGMA) was applied into each well and the plates were incubated for 90 minute in a CO2 incubator. After incubation, the reduced form of cytochrome C was measured at 550 nm with a Microplate reader (MR 700, Dynatech laboratories, USA) with spectral filter. To avoid measurement of superoxide that was not derived from PMN, we prepared wells with superoxide dismutase (SOD, SIGMA). The inhibitory effect of viscoelastics was expressed as a percentage of inhibition as follows:
BCEC were prepared with modification as described by Crawford KM et al.5 Briefly, bovine eyes were obtained from a local abattoir, and the corneas were excised with an attached scleral ring and placed, endothelium side up, in a concave plastic cup. The endothelial surface was ringed and subsequently incubated at 37℃ for 90 minutes in a sterile solution of 20mM HEPES buffered Earle's balanced salt solution (EBSS,GIBCO). Endothelial cells were dislodged from Descemet's membrane by gentle scraping using a spatula with forceps. Dislodged cells were aspirated with pipette and added with RPMI 1640 media with 10% FBS. The cells were gently centrifuged (600g, 2 minutes), resuspended in 5 ml of medium supplement and incubated in 25-cm2 flasks at 37℃ in 95% air-5%CO2. Third-passage cells were inoculated in a 96- well plate, 1 × 105 per well, and cultured for three additional days for confluencing. Into each well, we applied human PMN, 1 × 106 per well, and then supplied one of the three types of HA, or methylcellulose, at the four different concentrations (0.1, 1, 2, 3 mg/ml). After PMN activation with PMA, PMNs were incubated for 90 minutes and then washed out with HBSS. The viability of the remaining BCECwas measured with an MTT (3-[4,5-dimethylthiazol-2-yl]-2,5-diphenyl tetrasodium bromide, SIGMA) assay.6 The protective effect of viscoelastics was expressed as a percentage of protection as follows:
All of these procedures were repeated three times. The results were analyzed with one-way ANOVA according to molecular weight and concentration and Duncan's pairwise comparison test was performed. To analyze for the dual effects of molecular weight and concentration, we used two-way ANOVA. We calculated the above statistics using a PC-statistical analysis system program (SAS, version 6.12).
The degree of superoxide release decreased in a concentration-dependent manner in all three types of HA and methylcellulose (p < 0.0001) (Table 1). However, the comparison according to molecular weight at each concentration showed no significant difference (p value of 0.1mg/ml concentration was 0.8495, that of 1 mg/ml concentration was 0.0733) between types of viscoelastics except for methylcellulose and 4,000,000 MW HA, both over 2 mg/ml concentration: the percentage inhibition of 2mg/ml concentration of methylcellulose and 4,000,000 MW HA was 78.23 ± 2.08 and 86.69 ± 7.45, respectively (p = 0.0001), and of 3mg/ml concentration was 75.00 ± 2.08 and 78.43 ± 6.27, respectively (p = 0.0001) (Table 1). In two-way ANOVA analysis that considered both the effects of molecular weight and concentration of HA, both were found to influence the results. Specifically, HA inhibited superoxide release dose-dependently at concentrations over 0.1 mg/ml (Fig. 1). The mean values of cytochrome C reduction in SOD wells and viscoelastic-free wells were equal. This revealed that the main source of superoxide was PMN.
In all three types of HA and methylcellulose, the results of the MTT assay showed that the protective effect of viscoelastics on BCEC from activated PMN occurred in a concentration-dependent manner. However, there was no significant difference in the types of viscoelastics in the one-way ANOVA analysis: percentage protection of methylcellulose, and HA of MW 700,000, 2,000,000 and 4,000,000, was 112.22 ± 4.19, 113.2 ± 1.83, 108.7 ± 0.26 and 112.2 ± 1.05, respectively (p = 0.3663) (Table 2). In the two-way ANOVA, both type and concentration of viscoelastics influenced the results. In the concentration analysis, the BCEC survival rate was positively correlated with viscoelastic concentration: percentage protection of methylcellulose, and HA of MW 700,000, 2,000,000, 4,000,000, at 1 mg/ml, was 161.67 ± 10.12, 131.9 ± 3.23, 131.9 ± 3.67 and 135.1 ± 9.29, respectively (p = 0.0002), and at 2 mg/ml was 192.04 ± 24.90, 130.8 ± 4.86, 131.7 ± 7.35 and 132.5 ± 4.50, respectively (p = 0.0001) (Table 2). However, the curve of survival plateaued at concentrations over 1 mg/ml (Fig. 2). In the type analysis, there was no significant difference in BCEC viability except for methylcellulose wells in which more BCEC survived than in other wells (Fig. 2).
Recently, HA has been actively researched. Some investigators have shown that HA, which is normally present in umbilical cord, vitreous and synovial fluid, was depolymerized in the vitreous cavity by active oxygen,7 inhibited arthritis in the synovial cavity,8-10 and also inhibited the migration and phagocytosis of macrophages and PMN and superoxide release from them.3,4 In our study, we also found that HA inhibited superoxide released from PMN activated by the chemoactive peptide, PMA.Furthermore, this phenomenon occurred in a concentration-dependent manner similar to that reported in previous studies by other investigators. However, unlike other studies,3,4 these effects of HA were not proportional to molecular weight and did not occur only with high-molecular-weight HA. The results of our BCEC study also showed that the effect of HA was dependent on concentration, not molecular weight.
The action mechanism of HA's inhibition of cell migration, phagocytosis and superoxide release was not clearly understood until now. The inhibition probably occurs through the HA receptor that is located in the cell membrane. In addition, it is known that the hyaluronic receptor is a glycoprotein which penetrates the cell membrane and attaches to cytoskeletal proteins; its structure and molecular weight are similar to the CD44 adhesion glycoprotein.11 Since CD44 is involved with cellular mechanisms in T-lymphocyte and monocyte,12,13 someinvestigators think that HA, especially high-molecular-weight HA, acts like anti-CD44 antibody, inhibiting cell phagocytosis and chemotaxis by influencing the intracellular cytoskeleton through its receptor.4 If the action of HA occurs only through its receptor, HA can only inhibit superoxide release from PMN, but cannot remove pre-existing active oxygen like other oxygen scavengers. However, Artola et al14 showed that HA and hydroxypropylmethylcellulose (HPMC) protected rabbit corneal endothelial cells from hydrogen peroxide which was injected into the anterior chamber. They explained this effect by arguing that either HA formed a mechanical barrier between the corneal endothelium and injected hydrogen peroxide, acted as a chemical scavenger, or some combination of the two.
In our study, although there were statistically significant effects of inhibition and protection of HA found by two-way ANOVA, a cross-effect between molecular weight and concentration was observed. One-way ANOVA and Duncan's multiple pairwise comparison test were therefore performed. Table 1 and 2 show that HA inhibited the release of superoxide from PMN in a concentration-dependent manner and that the protection of BCEC resulted from this inhibition. In Figures 1 and 2, we can see that the above effects were statistically significant at concentration over 1 mg/ml. However, we could not find the proportional effect of molecular weight as in Suzuki and Yamaguchi's study.3 Furthermore, methylcellulose showed nearly the same or even better results in its inhibitory and protective effects as HA. From our results, we think that HA may not play a role through its receptor, but instead may behave as a mechanical barrier between either chemoactive peptide and PMN, or between superoxide and corneal endothelial, or act as an active-oxygen scavenger. We also can not rule out the possibility that two or all of these actions occur at the same time. Further studies of HA and its receptor will be necessary to understand its action mechanism.
Figures and Tables
Fig. 1
Amount of superoxide release (percentage score of reduced cytochrome C) depending on the concentration of viscoelastic substances. The inhibitory effects of superoxide release were influenced by both the molecular weight and concentration of hyaluronic acid. Specifically, hyaluronic acid inhibited superoxide release in proportion to its concentration over 0.1 mg/ml.
HA70: Hyaluronic Acid, molecular weight 700,000
HA200: Hyaluronic Acid, molecular weight 2,000,000
HA400: Hyaluronic Acid, molecular weight 4,000,000
*P < 0.05 compare to control concentration (0 mg/ml) by Duncan's multiple pairwise comparison test.
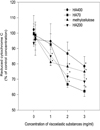
Fig. 2
Viability of bovine corneal endothelial cells (percentage score of MTT assay) depending on the concentration of viscoelastic substances. There was no significant difference in BCEC viability except for methylcellulose wells in which more BCEC survived than in other wells.
HA70: Hyaluronic Acid, molecular weight 700,000
HA200: Hyaluronic Acid, molecular weight 2,000,000
HA400: Hyaluronic Acid, molecular weight 4,000,000
*P < 0.05 compare to control concentration (0 mg/ml) by Duncan's multiple pairwise comparison test.
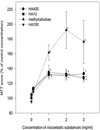
Table 1
Inhibitory effect of viscoelastics according to their types and concentrations (percentage, mean ± standard deviation) on PMN superoxide release.
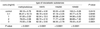
References
1. Liesegang TJ. Viscoelastic substances in ophthalmology. Surv Ophthalmol. 1990. 34:268–293.
2. Ayako A, Michael EY. Posttrabeculectomy anterior subcapsular cataract formation induced by anterior chamber air. Ophthalmic Surg. 1993. 24:314–319.
3. Suzuki Y, Yamaguchi T. Effect of hyaluronic acid on macrophage phagocytosis and active oxygen release. Agents Actions. 1993. 38:32–37.
4. Koichi T, Masahito T, Sachigy S, Hiromi N, Yoki M. Effects of high-molecular-weight hyaluronates on the functions of guinea pig polymorphonuclear leukocytes. Seminars in Arthritis and Rheumatism. 1993. 2:4–8.
5. Crawford KM, Ernst SA, Meyer RF, MacCallumDK . Na/K-ATPase pump sites in cultured bovine corneal endothelium of varying cell density at confluence. Invest Ophthalmol Vis sci. 1995. 36:1317–1326.
6. Wagner M, Benson MT, Rennie IG, MacNeil S. Intracellular regulation of retinal pigment epithelial cell attachment to extracellular matrix proteins. Curr Eye Res. 1995. 14:374–384.
7. Ueno N. Changes in vitreous structure caused by oxygen free radicals. Nippon Ganka Gakkai Zasshi. 1995. 99:1342–1360.
8. Saari H, Konttinen YT, Friman C, Sorsa T. Differential effects of reactive oxygen species on native synovial fluid and purified human umbilical cord hyaluronate. Inflammation. 1993. 17:403–415.
9. Schenck P, Schneider S, Miehlke R, Prehm P. Synthesis and degradation of hyaluronate by synovia from patients with rheumatoid arthritis. J Rheumatol. 1995. 22:400–405.
10. Fragonas E, Degrassi A, Kvam C, Matulova M, Pollesello P, Zanetti F, Vittur F. Oxygen-derived free radical (ODFR) action on hyaluronic acid (HA), on two HA ester derivatives, and on the metabolism of articular chondrocytes. Exp Cell Res. 1995. 218:79–86.
11. Lacy BE, Underhill CB. The hyaluronate receptor is associated with actin filaments. J Cell Biol. 1987. 105:1395–1404.
12. Miyake K, Underhill CB, Lesley J. Hyaluronate can function as a cell adhesion molecule and CD44 participates in hyaluronate recognition. J Exp Med. 1990. 172:69–75.
13. Culty M, Miyake K, Kincade PW. The hyaluronate receptor is a member of the CD44 (H-CAM) family of cell surface glycoproteins. J Cell Biol. 1990. 111:2765–2774.
14. Alberto A, Jorge LA, Juan LB, Jose MR. Protective properties of viscoelastic substances (sodium hyaluronate and 2% hydroxymethylcellulose) against experimental free radical damage to the corneal endothelium. Cornea. 1993. 12:109–114.