Abstract
Figures and Tables
Fig. 1

Fig. 2

Fig. 3
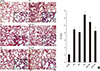
Journal List > J Korean Med Sci > v.29(4) > 1022525
In Bum Suh
https://orcid.org/http://orcid.org/0000-0001-7012-0305
Dae Wui Yoon
https://orcid.org/http://orcid.org/0000-0001-8875-2255
Won-Oak Oh
https://orcid.org/http://orcid.org/0000-0003-0156-3422
Eun Joo Lee
https://orcid.org/http://orcid.org/0000-0001-7884-9045
Kyung Hoon Min
https://orcid.org/http://orcid.org/0000-0003-0610-2182
Gyu Young Hur
https://orcid.org/http://orcid.org/0000-0001-5039-0199
Seung Heon Lee
https://orcid.org/http://orcid.org/0000-0002-7180-3877
Sung Yong Lee
https://orcid.org/http://orcid.org/0000-0002-8693-5792
Sang Yeub Lee
https://orcid.org/http://orcid.org/0000-0001-7565-1076
Chol Shin
https://orcid.org/http://orcid.org/0000-0002-2928-8576
Jae Jeong Shim
https://orcid.org/http://orcid.org/0000-0002-3095-1021
Kwang Ho In
https://orcid.org/http://orcid.org/0000-0003-3010-6423
Kyung Ho Kang
https://orcid.org/http://orcid.org/0000-0002-9778-1725
Je Hyeong Kim
https://orcid.org/http://orcid.org/0000-0002-8995-7460