Abstract
The use of musculoskeletal ultrasound in rheumatology clinical practice has rapidly increased over the past decade. Ultrasound has enabled rheumatologists to diagnose, prognosticate and monitor disease outcome. Although international standardization remains a concern still, the use of ultrasound in rheumatology is expected to grow further as costs fall and the opportunity to train in the technique improves. We present a review of value of ultrasound, focusing on major applications of ultrasound in rheumatologic diseases.
The use of musculoskeletal ultrasound in rheumatology has increased dramatically over the past decade both as a result of technological developments and a desire to identify and treat inflammation early. High quality ultrasound machines with good spatial resolution have provided rheumatologists with a means of identifying inflammation and structural damage, monitoring disease and predicting therapeutic responses. Ultrasound has clear advantages over other imaging tools such as magnetic resonance imaging (MRI) in terms of better tolerability, an ability to scan multiple joints at one sitting and its ability to directly correlate clinical and imaging findings. Furthermore high resolution gray scale images combined with Doppler technique have made it possible to detect slow low volume blood flow, opening new avenues for detecting and grading inflammation in joints. Based on these developments and increasing interest, a special Ultrasound Task Force on behalf of Outcome Measures in Rheumatology Clinical Trial (OMERACT) organization was formed to develop standardized ways of acquiring images in addition to defining and scoring pathologies relevant to rheumatologists (1). This has provided a framework for collaborative studies on ultrasound assessment and measurement issues. In this review, we focus on major values of ultrasound in rheumatologic diseases.
After the discovery of the piezo-electric effect in crystals by the Pierre and Curie brothers in 1880, further research and development in piezo-electricity followed. In particular, after the Titanic sank on its maiden voyage in 1912, scientists spurred on the development of how to detect submerged objects. The threat of German submarines to Allied shipping in World War I stimulated more research into the development of ultrasound devices (2). The first recorded detection and subsequent sinking of a submarine using an ultrasound device (called a hydrophone at that time) occurred in the Atlantic in 1916 during World War I. The military use of ultrasound lead to the development of medical diagnostic ultrasound. Of note during World War II, rapid developments and refinements in military RADAR (Radio Detection and Ranging which used electromagnetic waves rather than ultrasound) was the precursor for subsequent medical ultrasound devices. In 1942, the Austrian neurologist-psychiatrist Karl Theodore Dussik was generally regarded as the first physician to use ultrasound for medical diagnosis (3). He attempted to locate brain tumors by measuring the transmission of an ultrasound beam through the skull. Later in 1956, Ian Donald from Scotland and Thomas Graham Brown from Glasgow made the world's first two dimensional contact scanner where the transducer can be moved manually over the patient's abdomen with a resultant 2-D image reproduced on an oscilloscope. This brought the capability of ultrasound to a wider audience which in turn stimulated its further growth.
The first report pertaining to musculoskeletal tissue was in 1958 by Karl Theodore Dussik; titled "Measurement of articular tissue with ultrasound" and published in the American Journal of Physical Medicine. In this ex-vivo study, the attenuation of ultrasound during its propagation through human articular cartilage was first measured. Since this study was not related directly to clinical imaging, the study of "Ultrasound B-Scanning in the differentiating of Baker's Cyst and Thrombophlebitis" in vivo in 1972 by Daniel McDonald and George Leopold is widely regarded as the first publication of clinically relevant musculoskeletal ultrasound (4). They described the use of a contact B-mode scanner to differentiate Backer's cysts from thrombophlebitis. In 1969, Nicolaas Bom from the Netherlands developed the real-time multi-element linear array scanner which evolved into the very sophisticated real-time scanners that are widely available today in musculoskeletal ultrasound.
Since the first gray scale ultrasound demonstration of synovitis of knee joint in rheumatoid arthritis (5), numerous studies have demonstrated the clinical utility of ultrasound in evaluating fluid, synovial hypertrophy in inflammatory arthritis (6, 7). This was largely due to the fact that synovitis is the fundamental pathology which is seen in all types of inflammatory arthritis. Gray scale ultrasound can depict a range of abnormalities from the minimally thickened synovium to severe hypertrophy with fluid, debris and villi (Fig. 1A). Ultrasound has shown its superiority over clinical examination in the detection and assessment of synovitis, drawing the most attention and support with regard to the clinical use of ultrasound in rheumatology (8). Furthermore, with the administration of microbubbles contrast agents to increase backscattering, the sensitivity for the detection of a thickened, hypervascular synovium can be increased, and better quantification of degree of inflammation can be achieved (9, 10). With regard to scoring of gray scale synovitis used in studies, different scoring systems have been used, ranging from binary (yes/no) to semiquantitative 4-point (0-3) grading (11, 12).
Assessment of synovial activity expressed by increased vascularity is extremely important to the diagnosis and evaluation of treatment effect. Traditionally, visualization of synovial inflammation has mainly been dominated by MRI. Recently, Doppler ultrasound has been preferred to MRI in clinical practice, since Doppler mode allows the visualization of microvascularity within joint cavity and periarticular tissue. Doppler can provide useful clinical information regarding the presence or absence of flow through the joint (Fig. 2), which correlates with ongoing disease activities. The microvascular activity detected by Doppler ultrasound correlated well with histology specimen in knee and hip joint (13, 14). Moreover, intra-observer and inter-observer reliability of still image interpretation is high for gray scale and Doppler (15). Several studies used Doppler for follow-up assessment after the treatment of corticosteroids and tumor necrosis factor antagonists (9, 16, 17), showing Doppler able to detect changes in synovial perfusion. In these studies, the power or colour Doppler signal was determined semi-quantitatively graded on a 0-3 scale (17, 18) or qualitatively.
A semiquantitative grading system in which vascularity is scored from 0 to 3 according to number or areas of vessel signals are widely used in clinical practice (19-21). However, the semi-quantitative scales are not a precise method of vascularity measurement and can be prone to intra-observer and inter-observer variation. Alternatively, a quantitative scale quantified by measuring the Doppler colour pixels in the region of interest have also been used to measure inflammatory activity. Sonographically measured disease activity was quantified by counting colour pixels of region of interest using different vascularity analysis computer softwares (16, 22, 23), colour fraction defined as the fraction of colour pixels in a region of interest (24) and the area under the time-intensity curve with intravenous ultrasound contrast agent (9). However, the sensitivity of Doppler can be varied according to Doppler variables set by the ultrasonographer, as well as ultrasound units from different manufacturers (25). The lack of consensus make Doppler difficult to apply in routine clinical practice for assessment of disease activity, thus there needs to be further agreement on classifying the vascularity.
With regard to Doppler modalities, both colour and power Doppler have been used. Power Doppler displays the total back scattered energy mainly from red blood cells instead of mean Doppler shift used in colour Doppler. Power Doppler does not measure blood velocity or direction but is more sensitive to detect microvascular low-velocity flow. Moreover, power Doppler is less angle dependent compared to colour Doppler and technologically does not aliase (26). Owing to these theoretical advantages, power Doppler has been better suited for assessing musculoskeletal diseases in rheumatology. However, these advantages of power Doppler have been disappearing in the newer high-end machines where the trend is that colour Doppler now is more sensitive than power Doppler (25).
Tendons are also frequently involved in a wide range of inflammatory and non-inflammatory rheumatic diseases. Ultrasound is one of the best imaging modalities for assessing tendons due to its high image resolution. When diseased, tendons may become hypoechogenic with loss of a fibrillar pattern, thicker, have internal Doppler signals and a thickened surrounding tendon sheath which may exhibit Doppler (Fig. 1B). Tendon itself can show loss of fine fibrillar echotexture, focal thickening and hypoechoic (27). Ultrasound has been reported to be more sensitive than MRI for the detection of tenosynovitis (28). However, in a study by Wakefield et al, the sensitivity and specificity of ultrasound were reported 0.15-0.44, and 0.98-0.99 respectively for tenosynovitis in finger joints of patients with early untreated rheumatoid arhtritis using MRI as the gold standard (29). This lower value may be related to the lack of standardization of definition of tenosynovitis at that time, which was developed later by OMERACT.
Ultrasound combined with power Doppler has been used to investigate the cause of shoulder pain in patients with rheumatoid arthritis. Ultrasound can help to differentiate glenohumeral synovitis with subdeltoid bursitis, tenosynovitis of biceps tendon as the cause of rheumatoid shoulder (30). A study showed overall good agreement between ultrasound and MRI with regard to synovitis, erosion and bursitis and cuff tear of shoulder joint in patients with rheumatoid arthritis (31). Ultrasound can also be used to assess non-inflammatory cause of shoulder pain. Through a recent meta-analysis study with sixty-two studies assessing 6066 shoulders, it was concluded that ultrasound can be regarded as an appropriate technique for the assessment of rotator cuff tears with an acceptable sensitivity and specificity (32). In the elbow, both lateral and medial epicondylitis are due to an overuse injury from repetitive movement of the forearm and the wrist resulting in stressing of the common extensor or flexor tendons. Ultrasound is also informative and accurate for the detection of clinical lateral and medial epicondylitis (33, 34). These results can provide convincing evidence of capability of ultrasound in depicting mechanical tendon diseases as well as inflammatory causes in rheumatology clinical practice. Although MRI can also detect low grade tendinopathies, the overall advantages of repeatability, non-invasiveness, wide availability and ability to examine the tendon dynamically have made ultrasound more valuable tool for detecting tendon diseases. Thus ultrasound should be regarded as the first imaging modality for tendon involvement in rheumatologic diseases.
Radiographically detected bone erosion is an important diagnostic criterion for rheumatoid arthritis (35) and its presence implies poor functional outcome. However, plain radiography can show only the erosions which are tangential to the X-ray beam direction. In contrast, ultrasound can visualize circumferentially around the bone, which makes it possible to detect more erosions than simple radiography where erosions may be lost in the two dimensional projection (Fig. 1C). The first large study demonstrating the superiority of ultrasound over radiography was reported in 2000 (36), showing its ability to detect 6.5 fold more erosions than radiography in early rheumatoid arthritis patients as well as 3.4 fold in late disease. With MRI considered the reference method, ultrasound has a slightly lower sensitivity than MRI but similar accuracy for detecting erosions (37, 38). With regard to scoring of erosions, although scoring system based on size (36) and semiquantitative scores (39) have been suggested, no consensus has been made in standardized scoring system. A wide variety of sizes and locations within joints with sometimes inaccessible acoustic window have made it difficult to standardize. In addition, erosions may be difficult to detect in the context of concomitant osteoarthritis.
Osteoarthritis is traditionally evaluated by conventional radiography in spite of the fact that radiography cannot depict articular cartilage. Ultrasound can overcome this barrier since it can visualize articular hyaline cartilage as a well defined anechoic band lacking internal echoes (40). Ultrasound can also show pathologic signs of articular cartilage in terms of thickness, transparency, sharpness and related osteophytes. Nevertheless, ultrasound for the assessment of osteoarthritis seems to have relatively less been focused on compared to rheumatoid arthritis. Recently, interest in the application of ultrasound in early and late osteoarthritis is emerging (40, 41).
The first ultrasound study of cartilage in rheumatology dates back to 1992, where Iagnocco et al. reported diminished knee cartilage thickness, which is the hallmark of osteoarthritis, in osteoarthritis patients, suggesting ultrasound as a useful non-invasive method to study articular cartilage (42). Then, several studies investigated ultrasonographic cartilage abnormalities, reporting loss of sharp contour and clarity with increased echogenecity in joints involved by osteoarthritis (43, 44). MRI can show precise cartilage loss more sensitively than radiography and can also provide distinct advantages over radiography for joint space narrowing and its location (45). However, its use for the assessment of osteoarthritis has been limited due to high cost and accessibility in clinical practice. Thus, ultrasound has been focused on as an alternative valid non-invasive reproducible imaging modality to detect and quantify cartilage damage in osteoarthritis. Reflecting these interests, it was reported that insonation angle and sound speed in cartilage should be taken into account for accurate thickness measure (46).
There has been increasing evidence that synovitis plays a significant role as a contributor in the disease pathogenesis (47). In osteoarthritic joints, synovitis with similar findings to rheumatoid arthritis has been shown (44), supporting major roles of synovitis in the pathogenesis of osteoarthritis.
Osteophytes appearing as elevated small step-up bony fragment seen in two perpendicular planes close to joint space can be detected by ultrasound (Fig. 3). Depending on its interposition, it may be difficult to differentiate from bony erosions and normal cortical irregularity. Ultrasound can detect more osteophytes compared with conventional radiography osteoarthritis of hand (48). In erosive osteoarthritis of hands, ultrasound also found more osteophytes than the radiography although X-ray may be better to detect the centrally placed erosions where the acoustic window is poor (49). Several studies have introduced semiquantitative scoring systems for osteoarthritis in the knee, hand and hip joint (40, 50, 51), though no consensus has been reached. Efforts in order to validate scoring systems are being undertaken by the OMERACT Ultrasound Task Force (52).
Crystal deposit diseases are a group of disorders characterized by the intra and extra-articular deposition of crystals. Monosodium urate (MSU) crystals as a consequence of raised serum uric acid level and calcium pyrophosphate dihydrate (CPPD) crystals are the most common forms. Ultrasound has become a useful diagnostic tool for gout and pseudogout. The highly sparkling reflectivity of MSU (Fig. 1D) and CPPD crystals can be easily detected with even minimal aggregates within cartilage and tendon sheaths. Once these crystals are deposited in the cartilage, the reflectivity of the cartilage is no longer dependent on the insonation angle of ultrasound beam (53).
The existence of a hyperechoic band over anechoic hyaline cartilage surface described as a double contour sign are seen in about 92% of gouty joints (Fig. 1E) (54). It has also been demonstrated recently that the sonographic double contour signs of deposition of MSU crystals can be reversed completely on follow-up if sustained normouricemia was achieved (55). In a pilot study in patients with asymptomatic hyperuricemia, the presence of a double contour sign or hyperechoic cloud area was predictive of the detection of MSU crystals (56). Moreover, ultrasound can help distinguish tophi and other subcutaneous nodules including rheumatoid nodules and lipoma. A tophus, which is composed of MSU crystals, is most often seen as a heterogeneous mass often with intermittent hyperechoic foci compared with rheumatoid nodules which are likely to be more homogeneous (57). In CPPD disease, the hyperechoic aggregates are likely to form a band within articular cartilage, which is the distinct ultrasound feature differentiating MSU crystals (53). The dense deposit of CPPD crystals ranging from punctate to large linear deposits can generate acoustic shadowing (58).
The use of ultrasound in spondyloarthropathy is mainly for the detection of enthesitis such as Achilles tendonitis and plantar fasciitis. The other indication is for the detection of peripheral joint arthritis, similar to rheumatoid arthritis (59). Affected entheseal sites show signs of gray scale abnormality characterized by loss of normal fibrillar structures with an increased thickness or intra-tendinal focal changes with or without Dopper signals (Fig. 1F). In a large scale study for peripheral enthesitis in patients with spondyloarthropathies, greater sensitivity for the detection of enthesitis over clinical examination was reported (60). Power Doppler can also display the degree of severity of enthesial involvement. In contrast, the role of MRI in the assessment of spondyloarthropathies has mainly been confined to assess axial involvement since MRI lacks sensitivity and specificity for peripheral enthesitis.
Different scoring systems have also been suggested for enthesisitis. Each assesses different sites and different scoring systems. The earlier ones are only gray scale with the more recent ones involving Doppler. The first of these reported in 2006 was the Glasgow Ultrasound Enthesitis Scoring System (GUESS) which assesses five entheses of lower limb with gray-scale findings (61). Another scoring system SEI (Sonographic Enthesitis Index) also uses gray scale findings but assess different entheseal sites (62). A more recent Madrid Sonographic Enthesis Index (MASEI) scoring system combined gray scale abnormalities and Doppler activity. However, a recent systematic literature review by the OMERACT Ultrasound Task Force concluded that there remains a lack of consensus of how to define and score enthesitis. One important remit is to develop a score which separates inflammation from damage. This is a priority area currently under review by the OMERACT group (63).
Diagnostic and therapeutic musculoskeletal interventions in rheumatology include a wide range of procedures such as aspiration of fluid from joints, bursa, tendon sheath and cystic lesions for diagnostic and therapeutic purpose as well as steroid injections into joint cavities and soft tissues. Real time visualization of the needle and injected steroid by ultrasound enables the reliable placement of the needle tip in the joint cavity, bursa, tendon sheaths and intra-tendon (Fig. 4) (64). However, some studies reported no significant difference in accuracy between the blind and ultrasound-guided method (65, 66), although these results are studies of the larger joints such as knee and shoulder. In the relatively small interphalangeal joints and difficult joints to be accessed due to complex anatomy such as wrist and ankle, accurate needle positioning can be a particular challenge (67). In these joints, sonographic needle guidance have demonstrated improved accuracy and better clinical outcomes compared with conventional palpation-guided blind methods (68, 69). Furthermore, Doppler can help the precise placement of the needle tip into the most inflamed intra-region, making the overall clinical outcomes better. A recent systematic review compared the accuracy of ultrasound-guided intra-articular injections with blind injections using palpation or anatomic landmarks, and confirmed that ultrasound-guidance raised the accuracy of injections independent of anatomic site (70).
Vasculitis is an autoimmune disease characterized by the inflammation of the vessel wall. The lumen of large arteries can be measured and information about vessel wall, pulsatility and blood flow characteristics can be obtained using duplex mode. In rheumatology, the first ultrasound report for the diagnosis of arteritis was for giant cell (temporal) arteritis in 1995 (71). Since then, giant cell arteritis has drawn the most interest in arterits in rheumatology (71, 72). Affected temporal arteries showed edema, stenosis or occlusion characterized by a hypoechoic halo around a narrowed lumen due to edema of arterial wall, which may make possible the diagnosis of temporal arteritis without performing a temporal-artery biopsy (73).
Since then, the scope of vasculitis evaluated with ultrasound in rheumatology has expanded to Wegener's granulomatosis (74), finger arteritis in systemic sclerosis (75) and Raynaud's phenomenon (76), in which ultrasound showed superiority to visualize the narrowed lumen with thicken arterial wall. High resolution ultrasound may aid to differentiate primary and secondary Raynaud's phenomenon by depicting the vessels as angiography. At present, ultrasound for the assessment of arteritis is expected to be a valuable tool for diagnostic workup and monitoring in rheumatology.
The normal ultrasound echogenicity of major salivary glands is homogeneous. As affected glands gradually become fibrotic, the parenchyma becomes inhomogeneous with multiple scattered small oval hypoechoic or anechoic areas with flow observed within the glands in Doppler (Fig. 5) (77, 78). These findings appear to have a high sensitivity and specificity for primary Sjögren syndrome (79). Ultrasound findings of parotid glands correlated well with MRI, showing a similar diagnostic accuracy compared to MRI (80) and sialography (81). Moreover, additional vascularity information in the glands obtained by spectral Doppler in terms of resistive index and pulsatility index can be helpful for the diagnosis of Sjögren syndrome (82). However, inconsistent ultrasonographic diagnostic sensitivity ranging 43%-93% and specificity ranging 64%-100% for Sjögren syndrome have been reported (81, 83, 84). These inconsistencies could be caused by lack of ultrasonographic classification criteria for Sjögren syndrome. Moreover, a different ultrasonographic scoring system based on morphologic changes observed in gray scale ultrasound has been suggested for primary Sjögren syndrome (79, 85). Despite these flaws, the overall diagnostic accuracy of an ultrasound scoring system for primary Sjögren syndrome was comparable to salivary scintigraphy and salivary gland biopsy (81). Since ultrasound can demonstrate valuable objective evidence of glandular involvement of Sjögren syndrome, it is regarded as an emerging imaging method of first choice in patients suspected of having sicca syndrome (86).
Sjögren syndrome is frequently associated with a variety of B cell lymphomas and other lymphoproliferative disorders (87). Sometimes the differential diagnosis of ultrasonographic findings can be challenging, thus meticulous studies should be undertaken if concomitant lymphoma cannot be ruled out as well as the appreciation that ultrasound cannot always exclude more severe pathologies in which case other imaging may be employed (88).
Skeletal muscles appears relatively hypoechogenic under normal circumstances in contrast to echogenic fibroadipose septa and fascia. In rheumatology, idiopathic inflammatory muscle diseases including polymyositis, dermatomyositis and inclusion body myositis can affect skeletal muscles focally and diffusely. On ultrasound, affected muscle can show thickening and areas of hypoechogenicity different to the normal surrounding muscle. Increased Doppler activity within the affected muscles can be observed. Ultrasound-guided closed muscle biopsy can also provide a minimally invasive approach for the diagnosis of focal myositis (89). However, the role of ultrasound in the assessment of inflammatory muscle diseases in rheumatology have not yet been fully identified.
The use of ultrasound in rheumatological diseases may be limited by several factors. The ultrasound beam cannot penetrate bone, making it impossible to visualize intraosseous changes such as bone marrow edema. Additionally, the ultrasound beam is attenuated (absorbed, refracted, diffracted or lost as heat) the deeper into tissue it goes and so images are less clear when interrogating deeper structures even when low frequency probes are used. Moreover, joints with a limited acoustic window due to anatomical restriction such as the hip, and wrist may have a lower sensitivity to detect erosions and involvement of synovial pathologies. Although high intra-observer and inter-observer reliability have been reported (31, 90, 91), the detection of ultrasonographic abnormalities depends on the individual operator. However the studies from the OMERACT group have demonstrated that with standardization, operator dependency can be diminished. To become an expert, plenty of clinical experience and a long period of training is needed. EULAR (European League Against Rheumatism) and the ACR (American College of Rheumatology) are currently setting out curricula and standard operating procedures for the acquisition and reading of ultrasound images.
Three dimensional (3D) ultrasound provides an interesting aspect for the volumetric assessment of tissue blocks and quantification of region of interests. The saved images can be viewed in sagittal, coronal and axial planes and relatively operator independent compared to two dimensional (2D) ultrasound imaging. The 3D images had a good correlation with 2D images for detection of synovitis and bone erosion (92) and was able to delineate the shape of the synovium in the knee joint (93). The use of 3D ultrasound currently remains mainly in the research area due to relatively low image quality and additional cost.
Sonoelastography measures the strain of the tissues by analyzing ultrasound echo signals while the probe compresses or relaxes the tissue (94). The compression wave may be generated by the operator (compression elastography) or by the probe (shear wave elastography). In rheumatology, sonoelastography has shown promising results in detecting changes in Achilles tendons (95) (Fig. 6), and elbow ligaments (96) and has allowed the differentiation between rheumatoid nodules and gout tophi (97). In systemic sclerosis patients, sonoelastography can demonstrate reduction of strain caused by loss of elasticity in the dermis (98). Since sonoelastography has started to be applied in rheumatologic diseases recently, more comprehensive and extended studies are needed to establish the contribution of sonoelastography in rheumatologic diseases.
Another interesting advance is known as fusion imaging. Here the ultrasound image is fused with MRI or Computed Tomography (CT) image to combine the advantages of real time image acquisition by ultrasound with better depiction of bone and soft tissue lesions. This technique can be used to improve injection accuracy of joints with difficult access such as sacroiliac joint (99). However, the usefulness of fusion imaging in rheumatology should be decided in the future since there is lack of clinical research data.
Ultrasound is becoming an essential tool in the management of rheumatology patients through its gradual incorporation into routine clinical use in many countries and rheumatology centers. Evidence for the reliability, validity as well as clinical value of ultrasound is increasing with continuing studies of this modality. Future development in technology together with consensus of international and national educational programs will spur the wider application of ultrasound for various rheumatologic diseases, enabling it to become a powerful imaging tool for rheumatologists.
Figures and Tables
Fig. 1
Ultrasound images of joints and peri-articular tissue showing typical signs of common rheumatologic diseases. (A) Longitudinal dorsal scan of the tibiotalar joint. A large ankle joint effusion with synovial proliferation (arrows) is seen. (B) Transverse and longitudinal scan of 3rd extensor tendon at dorsum of metacarpophalangeal joint showing tendinopathy represented as swelling of tendon sheath (arrow) in both plane. (C) In the transverse anterior scan of shoulder at 90° internal rotation, bony erosion (arrow) is seen. (D) MSD crystals are deposited within tendon sheath, causing tenosynovitis of extensor tendons. The high sparkling reflectivity of MSD crystals can make it easier to be detected and can be differentiated from synovial proliferation. (E) The sonographic double contour sign (arrow) is seen by the deposition of MSD crystals in the cartilage surface layers of knee joint. It is also characterized by angle independency (not demonstrated). (F) Achilles tendon near calcaneal insertion shows focal increased thickness and loss of fibrillar structures (arrow).
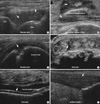
Fig. 2
Assessment of inflammatory activity can be achieved with power Doppler. (A, B) These are two longitudinal dorsal views through the wrist joint. Both show moderate levels of gray scale and power Doppler abnormalities consistent with active joint disease.
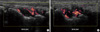
Fig. 3
Osteophyte usually appears as elevated small bony prominence (arrow) at the end of normal bone contour, close to joint space (A). It usually does not show Doppler signal (B).
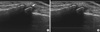
Fig. 4
Ultrasound guided intra-articular injection of 3rd proximal interphalageal joint of hand. (A) The tip and shaft of metallic needle (arrows) can be easily identified. (B) The crystalline steroid suspension can be observed on the screen as fine hyperechoic clouds or spots (arrow), which can increase the accuracy of injection.
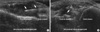
Fig. 5
Transverse view of the parotid gland in a patient with primary Sjögren syndrome. Affected glands can show parenchymal inhomogenecity with multiple oval shaped small hypoechoic changes (courtesy of Sandrine Jousse-Joulin).
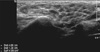
Fig. 6
Elastography of wrist joint. In the left image, longitudinal gray scale image over the ulnocarpal area in rheumatoid arthritis patient shows loss of fibrillar pattern and peritendinous synovial proliferation (arrows). In the right elastography image, tissue elasticity was displayed using colours. The hard tissue areas are seen as blue and soft tissue areas are seen as red colour. Swollen tendon regions in the gray scale are seen as red in the elastography (arrow), representing the decreased elasticity (hardness) of this region due to tendinopathies affected by rheumatoid arthritis.
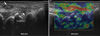
References
1. Wakefield RJ, Balint PV, Szkudlarek M, Filippucci E, Backhaus M, D'Agostino MA, Sanchez EN, Iagnocco A, Schmidt WA, Bruyn GA, et al. Musculoskeletal ultrasound including definitions for ultrasonographic pathology. J Rheumatol. 2005. 32:2485–2487.
2. Kane D, Grassi W, Sturrock R, Balint PV. A brief history of musculoskeletal ultrasound: 'from bats and ships to babies and hips'. Rheumatology (Oxford). 2004. 43:931–933.
3. Dussik KT. On the possibility of using ultrasound waves as a diagnostic aid. Z Neurol Psychiatr. 1942. 174:153–168.
4. McDonald DG, Leopold GR. Ultrasound B-scanning in the differentiation of Baker's cyst and thrombophlebitis. Br J Radiol. 1972. 45:729–732.
5. Cooperberg PL, Tsang I, Truelove L, Knickerbocker WJ. Gray scale ultrasound in the evaluation of rheumatoid arthritis of the knee. Radiology. 1978. 126:759–763.
6. Grassi W, Tittarelli E, Pirani O, Avaltroni D, Cervini C. Ultrasound examination of metacarpophalangeal joints in rheumatoid arthritis. Scand J Rheumatol. 1993. 22:243–247.
7. Backhaus M. Ultrasound and structural changes in inflammatory arthritis: synovitis and tenosynovitis. Ann N Y Acad Sci. 2009. 1154:139–151.
8. Jain M, Samuels J. Musculoskeletal ultrasound in the diagnosis of rheumatic disease. Bull NYU Hosp Jt Dis. 2010. 68:183–190.
9. Salaffi F, Carotti M, Manganelli P, Filippucci E, Giuseppetti GM, Grassi W. Contrast-enhanced power Doppler sonography of knee synovitis in rheumatoid arthritis: assessment of therapeutic response. Clin Rheumatol. 2004. 23:285–290.
10. Szkudlarek M, Court-Payen M, Strandberg C, Klarlund M, Klausen T, Østergaard M. Contrast-enhanced power Doppler ultrasonography of the metacarpophalangeal joints in rheumatoid arthritis. Eur Radiol. 2003. 13:163–168.
11. Scheel AK, Hermann KG, Kahler E, Pasewaldt D, Fritz J, Hamm B, Brunner E, Müller GA, Burmester GR, Backhaus M. A novel ultrasonographic synovitis scoring system suitable for analyzing finger joint inflammation in rheumatoid arthritis. Arthritis Rheum. 2005. 52:733–743.
12. Karim Z, Wakefield RJ, Quinn M, Conaghan PG, Brown AK, Veale DJ, O'Connor P, Reece R, Emery P. Validation and reproducibility of ultrasonography in the detection of synovitis in the knee: a comparison with arthroscopy and clinical examination. Arthritis Rheum. 2004. 50:387–394.
13. Walther M, Harms H, Krenn V, Radke S, Faehndrich TP, Gohlke F. Correlation of power Doppler sonography with vascularity of the synovial tissue of the knee joint in patients with osteoarthritis and rheumatoid arthritis. Arthritis Rheum. 2001. 44:331–338.
14. Walther M, Harms H, Krenn V, Radke S, Kirschner S, Gohlke F. Synovial tissue of the hip at power Doppler US: correlation between vascularity and power Doppler US signal. Radiology. 2002. 225:225–231.
15. Cheung PP, Dougados M, Gossec L. Reliability of ultrasonography to detect synovitis in rheumatoid arthritis: a systematic literature review of 35 studies (1,415 patients). Arthritis Care Res (Hoboken). 2010. 62:323–334.
16. Taylor PC, Steuer A, Gruber J, McClinton C, Cosgrove DO, Blomley MJ, Marsters PA, Wagner CL, Maini RN. Ultrasonographic and radiographic results from a two-year controlled trial of immediate or one-year-delayed addition of infliximab to ongoing methotrexate therapy in patients with erosive early rheumatoid arthritis. Arthritis Rheum. 2006. 54:47–53.
17. Fiocco U, Ferro F, Vezzù M, Cozzi L, Checchetto C, Sfriso P, Botsios C, Ciprian L, Armellin G, Nardacchione R, et al. Rheumatoid and psoriatic knee synovitis: clinical, grey scale, and power Doppler ultrasound assessment of the response to etanercept. Ann Rheum Dis. 2005. 64:899–905.
18. Naredo E, Möller I, Cruz A, Carmona L, Garrido J. Power Doppler ultrasonographic monitoring of response to anti-tumor necrosis factor therapy in patients with rheumatoid arthritis. Arthritis Rheum. 2008. 58:2248–2256.
19. Szkudlarek M, Court-Payen M, Strandberg C, Klarlund M, Klausen T, Ostergaard M. Power Doppler ultrasonography for assessment of synovitis in the metacarpophalangeal joints of patients with rheumatoid arthritis: a comparison with dynamic magnetic resonance imaging. Arthritis Rheum. 2001. 44:2018–2023.
20. Stone M, Bergin D, Whelan B, Maher M, Murray J, McCarthy C. Power Doppler ultrasound assessment of rheumatoid hand synovitis. J Rheumatol. 2001. 28:1979–1982.
21. Newman JS, Adler RS, Bude RO, Rubin JM. Detection of soft-tissue hyperemia: value of power Doppler sonography. AJR Am J Roentgenol. 1994. 163:385–389.
22. Hau M, Kneitz C, Tony HP, Keberle M, Jahns R, Jenett M. High resolution ultrasound detects a decrease in pannus vascularisation of small finger joints in patients with rheumatoid arthritis receiving treatment with soluble tumour necrosis factor alpha receptor (etanercept). Ann Rheum Dis. 2002. 61:55–58.
23. Fukae J, Kon Y, Henmi M, Sakamoto F, Narita A, Shimizu M, Tanimura K, Matsuhashi M, Kamishima T, Atsumi T, et al. Change of synovial vascularity in a single finger joint assessed by power doppler sonography correlated with radiographic change in rheumatoid arthritis: comparative study of a novel quantitative score with a semiquantitative score. Arthritis Care Res (Hoboken). 2010. 62:657–663.
24. Ellegaard K, Christensen R, Torp-Pedersen S, Terslev L, Holm CC, Kønig MJ, Jensen PS, Danneskiold-Samsøe B, Bliddal H. Ultrasound Doppler measurements predict success of treatment with anti-TNF-α drug in patients with rheumatoid arthritis: a prospective cohort study. Rheumatology (Oxford). 2011. 50:506–512.
25. Torp-Pedersen ST, Terslev L. Settings and artefacts relevant in colour/power Doppler ultrasound in rheumatology. Ann Rheum Dis. 2008. 67:143–149.
26. Rubin JM, Bude RO, Carson PL, Bree RL, Adler RS. Power Doppler US: a potentially useful alternative to mean frequency-based color Doppler US. Radiology. 1994. 190:853–856.
27. Grassi W, Filippucci E, Farina A, Cervini C. Sonographic imaging of tendons. Arthritis Rheum. 2000. 43:969–976.
28. Backhaus M, Kamradt T, Sandrock D, Loreck D, Fritz J, Wolf KJ, Raber H, Hamm B, Burmester GR, Bollow M. Arthritis of the finger joints: a comprehensive approach comparing conventional radiography, scintigraphy, ultrasound, and contrast-enhanced magnetic resonance imaging. Arthritis Rheum. 1999. 42:1232–1245.
29. Wakefield RJ, O'Connor PJ, Conaghan PG, McGonagle D, Hensor EM, Gibbon WW, Brown C, Emery P. Finger tendon disease in untreated early rheumatoid arthritis: a comparison of ultrasound and magnetic resonance imaging. Arthritis Rheum. 2007. 57:1158–1164.
30. Stegbauer J, Rump LC, Weiner SM. Sites of inflammation in painful rheumatoid shoulder assessed by musculoskeletal ultrasound and power Doppler sonography. Rheumatol Int. 2008. 28:459–465.
31. Bruyn GA, Naredo E, Möller I, Moragues C, Garrido J, de Bock GH, d'Agostino MA, Filippucci E, Iagnocco A, Backhaus M, et al. Reliability of ultrasonography in detecting shoulder disease in patients with rheumatoid arthritis. Ann Rheum Dis. 2009. 68:357–361.
32. Smith TO, Back T, Toms AP, Hing CB. Diagnostic accuracy of ultrasound for rotator cuff tears in adults: a systematic review and meta-analysis. Clin Radiol. 2011. 66:1036–1048.
33. Park GY, Lee SM, Lee MY. Diagnostic value of ultrasonography for clinical medial epicondylitis. Arch phys Med Rehabil. 2008. 89:738–742.
34. Tran N, Chow K. Ultrasonography of the elbow. Semin Musculoskelet Radiol. 2007. 11:105–116.
35. Arnett FC, Edworthy SM, Bloch DA, McShane DJ, Fries JF, Cooper NS, Healey LA, Kaplan SR, Liang MH, Luthra HS, et al. The American Rheumatism Association 1987 revised criteria for the classification of rheumatoid arthritis. Arthritis Rheum. 1988. 31:315–324.
36. Wakefield RJ, Gibbon WW, Conaghan PG, O'Connor P, McGonagle D, Pease C, Green MJ, Veale DJ, Isaacs JD, Emery P. The value of sonography in the detection of bone erosions in patients with rheumatoid arthritis: a comparison with conventional radiography. Arthritis Rheum. 2000. 43:2762–2770.
37. Døhn UM, Ejbjerg BJ, Court-Payen M, Hasselquist M, Narvestad E, Szkudlarek M, Møller JM, Thomsen HS, Østergaard M. Are bone erosions detected by magnetic resonance imaging and ultrasonography true erosions? a comparison with computed tomography in rheumatoid arthritis metacarpophalangeal joints. Arthritis Res Ther. 2006. 8:R110.
38. Szkudlarek M, Narvestad E, Klarlund M, Court-Payen M, Thomsen HS, Østergaard M. Ultrasonography of the metatarsophalangeal joints in rheumatoid arthritis: comparison with magnetic resonance imaging, conventional radiography, and clinical examination. Arthritis Rheum. 2004. 50:2103–2112.
39. Reynolds PP, Heron C, Pilcher J, Kiely PD. Prediction of erosion progression using ultrasound in established rheumatoid arthritis: a 2-year follow-up study. Skeletal Radiol. 2009. 38:473–478.
40. Naredo E, Acebes C, Möller I, Canillas F, de Agustín JJ, de Miguel E, Filippucci E, Iagnocco A, Moragues C, Tuneu R, et al. Ultrasound validity in the measurement of knee cartilage thickness. Ann Rheum Dis. 2009. 68:1322–1327.
41. Conaghan P, D'Agostino MA, Ravaud P, Baron G, Le Bars M, Grassi W, Martin-Mola E, Wakefield R, Brasseur JL, So A, et al. EULAR report on the use of ultrasonography in painful knee osteoarthritis: part 2: exploring decision rules for clinical utility. Ann Rheum Dis. 2005. 64:1710–1714.
42. Iagnocco A, Coari G, Zoppini A. Sonographic evaluation of femoral condylar cartilage in osteoarthritis and rheumatoid arthritis. Scand J Rheumatol. 1992. 21:201–203.
43. Grassi W, Filippucci E, Farina A. Ultrasonography in osteoarthritis. Semin Arthritis Rheum. 2005. 34:19–23.
44. D'Agostino MA, Conaghan P, Le Bars M, Baron G, Grassi W, Martin-Mola E, Wakefield R, Brasseur JL, So A, Backhaus M, et al. EULAR report on the use of ultrasonography in painful knee osteoarthritis: part 1: prevalence of inflammation in osteoarthritis. Ann Rheum Dis. 2005. 64:1703–1709.
45. Amin S, LaValley MP, Guermazi A, Grigoryan M, Hunter DJ, Clancy M, Niu J, Gale DR, Felson DT. The relationship between cartilage loss on magnetic resonance imaging and radiographic progression in men and women with knee osteoarthritis. Arthritis Rheum. 2005. 52:3152–3159.
46. Torp-Pedersen S, Bartels EM, Wilhjelm J, Bliddal H. Articular cartilage thickness measured with US is not as easy as it appears: a systematic review of measurement techniques and image interpretation. Ultraschall Med. 2011. 32:54–61.
47. Attur M, Samuels J, Krasnokutsky S, Abramson SB. Targeting the synovial tissue for treating osteoarthritis (OA): where is the evidence? Best Pract Res Clin Rheumatol. 2010. 24:71–79.
48. Keen HI, Wakefield RJ, Grainger AJ, Hensor EM, Emery P, Conaghan PG. Can ultrasonography improve on radiographic assessment in osteoarthritis of the hands? a comparison between radiographic and ultrasonographic detected pathology. Ann Rheum Dis. 2008. 67:1116–1120.
49. Vlychou M, Koutroumpas A, Malizos K, Sakkas LI. Ultrasonographic evidence of inflammation is frequent in hands of patients with erosive osteoarthritis. Osteoarthritis Cartilage. 2009. 17:1283–1287.
50. Keen HI, Lavie F, Wakefield RJ, D'Agostino MA, Hammer HB, Hensor E, Pendleton A, Kane D, Guerini H, Schueller-Weidekamm C, et al. The development of a preliminary ultrasonographic scoring system for features of hand osteoarthritis. Ann Rheum Dis. 2008. 67:651–655.
51. Qvistgaard E, Torp-Pedersen S, Christensen R, Bliddal H. Reproducibility and inter-reader agreement of a scoring system for ultrasound evaluation of hip osteoarthritis. Ann Rheum Dis. 2006. 65:1613–1619.
52. Naredo E, Wakefield RJ, Iagnocco A, Terslev L, Filippucci E, Gandjbakhch F, Aegerter P, Aydin S, Backhaus M, Balint PV, et al. The OMERACT ultrasound task force: status and perspectives. J Rheumatol. 2011. 38:2063–2067.
53. Grassi W, Meenagh G, Pascual E, Filippucci E. "Crystal clear"-sonographic assessment of gout and calcium pyrophosphate deposition disease. Semin Arthritis Rheum. 2006. 36:197–202.
54. Thiele RG, Schlesinger N. Diagnosis of gout by ultrasound. Rheumatology (Oxford). 2007. 46:1116–1121.
55. Thiele RG, Schlesinger N. Ultrasonography shows disappearance of monosodium urate crystal deposition on hyaline cartilage after sustained normouricemia is achieved. Rheumatol Int. 2010. 30:495–503.
56. De Miguel E, Puig JG, Castillo C, Peiteado D, Torres RJ, Martín-Mola E. Diagnosis of gout in patients with asymptomatic hyperuricaemia: a pilot ultrasound study. Ann Rheum Dis. 2012. 71:157–158.
57. Nalbant S, Corominas H, Hsu B, Chen LX, Schumacher HR, Kitumnuaypong T. Ultrasonography for assessment of subcutaneous nodules. J Rheumatol. 2003. 30:1191–1195.
58. Ciapetti A, Filippucci E, Gutierrez M, Grassi W. Calcium pyrophosphate dihydrate crystal deposition disease: sonographic findings. Clin Rheumatol. 2009. 28:271–276.
59. Jain M, Samuels J. Musculoskeletal ultrasound in the diagnosis of rheumatic disease. Bull NYU Hosp Jt Dis. 2010. 68:183–190.
60. McGonagle D, Marzo-Ortega H, O'Connor P, Gibbon W, Pease C, Reece R, Emery P. The role of biomechanical factors and HLA-B27 in magnetic resonance imaging-determined bone changes in plantar fascia enthesopathy. Arthritis Rheum. 2002. 46:489–493.
61. Balint PV, Kane D, Wilson H, McInnes IB, Sturrock RD. Ultrasonography of entheseal insertions in the lower limb in spondyloarthropathy. Ann Rheum Dis. 2002. 61:905–910.
62. Alcalde M, Acebes JC, Cruz M, González-Hombrado L, Herrero-Beaumont G, Sánchez-Pernaute O. A sonographic enthesitic index of lower limbs is a valuable tool in the assessment of ankylosing spondylitis. Ann Rheum Dis. 2007. 66:1015–1019.
63. Gandjbakhch F, Terslev L, Joshua F, Wakefield RJ, Naredo E, D'Agostino MA. OMERACT Ultrasound Task Force. Ultrasound in the evaluation of enthesitis: status and perspectives. Arthritis Res Ther. 2011. 13:R188.
64. Schirmer M, Duftner C, Schmidt WA, Dejaco C. Ultrasonography in inflammatory rheumatic disease: an overview. Nat Rev Rheumatol. 2011. 7:479–488.
65. Wiler JL, Costantino TG, Filippone L, Satz W. Comparison of ultrasound-guided and standard landmark techniques for knee arthrocentesis. J Emerg Med. 2010. 39:76–82.
66. Rutten MJ, Maresch BJ, Jager GJ, de Waal Malefijt MC. Injection of the subacromial-subdeltoid bursa: blind or ultrasound-guided? Acta Orthop. 2007. 78:254–257.
67. Raza K, Lee CY, Pilling D, Heaton S, Situnayake RD, Carruthers DM, Buckley CD, Gordon C, Salmon M. Ultrasound guidance allows accurate needle placement and aspiration from small joints in patients with early inflammatory arthritis. Rheumatology (Oxford). 2003. 42:976–979.
68. Sibbitt WL Jr, Peisajovich A, Michael AA, Park KS, Sibbitt RR, Band PA, Bankhurst AD. Does sonographic needle guidance affect the clinical outcome of intraarticular injections? J Rheumatol. 2009. 36:1892–1902.
69. Koski JM. Ultrasound guided injections in rheumatology. J Rheumatol. 2000. 27:2131–2138.
70. Gilliland CA, Salazar LD, Borchers JR. Ultrasound versus anatomic guidance for intra-articular and periarticular injection: a systematic review. Phys Sportsmed. 2011. 39:121–131.
71. Schmidt WA, Kraft HE, Völker L, Vorpahl K, Gromnica-Ihle EJ. Colour Doppler sonography to diagnose temporal arteritis. Lancet. 1995. 345:866.
72. Nesher G, Shemesh D, Mates M, Sonnenblick M, Abramowitz HB. The predictive value of the halo sign in color Doppler ultrasonography of the temporal arteries for diagnosing giant cell arteritis. J Rheumatol. 2002. 29:1224–1226.
73. Schmidt WA, Kraft HE, Vorpahl K, Völker L, Gromnica-Ihle EJ. Color duplex ultrasonography in the diagnosis of temporal arteritis. N Engl J Med. 1997. 337:1336–1342.
74. Schmidt WA, Seipelt E, Molsen HP, Poehls C, Gromnica-ihle EJ. Vasculitis of the internal carotid artery in Wegener's granulomatosis: comparison of ultrasonography, angiography, and MRI. Scand J Rheumatol. 2001. 30:48–50.
75. Schmidt WA, Wernicke D, Kiefer E, Gromnica-Ihle E. Colour duplex sonography of finger arteries in vasculitis and in systemic sclerosis. Ann Rheum Dis. 2006. 65:265–267.
76. Schmidt WA, Krause A, Schicke B, Wernicke D. Color Doppler ultrasonography of hand and finger arteries to differentiate primary from secondary forms of Raynaud's phenomenon. J Rheumatol. 2008. 35:1591–1598.
77. Niemelä RK, Takalo R, Pääkkö E, Suramo I, Päivänsalo M, Salo T, Hakala M. Ultrasonography of salivary glands in primary Sjogren's syndrome: a comparison with magnetic resonance imaging and magnetic resonance sialography of parotid glands. Rheumatology (Oxford). 2004. 43:875–879.
78. Steiner E, Graninger W, Hitzelhammer J, Lakits A, Petera P, Franz P, Gritzmann N. Color-coded duplex sonography of the parotid gland in Sjögren's syndrome. Rofo. 1994. 160:294–298.
79. Hocevar A, Ambrozic A, Rozman B, Kveder T, Tomsic M. Ultrasonographic changes of major salivary glands in primary Sjogren's syndrome: diagnostic value of a novel scoring system. Rheumatology (Oxford). 2005. 44:768–772.
80. Makula E, Pokorny G, Kiss M, Vörös E, Kovács L, Kovács A, Csernay L, Palkó A. The place of magnetic resonance and ultrasonographic examinations of the parotid gland in the diagnosis and follow-up of primary Sjögren's syndrome. Rheumatology (Oxford). 2000. 39:97–104.
81. Milic VD, Petrovic RR, Boricic IV, Marinkovic-Eric J, Radunovic GL, Jeremic PD, Pejnovic NN, Damjanov NS. Diagnostic value of salivary gland ultrasonographic scoring system in primary Sjogren's syndrome: a comparison with scintigraphy and biopsy. J Rheumatol. 2009. 36:1495–1500.
82. Chikui T, Yonetsu K, Izumi M, Eguchi K, Nakamura T. Abnormal blood flow to the submandibular glands of patients with Sjögren's syndrome: Doppler waveform analysis. J Rheumatol. 2000. 27:1222–1228.
83. De Vita S, Lorenzon G, Rossi G, Sabella M, Fossaluzza V. Salivary gland echography in primary and secondary Sjögren's syndrome. Clin Exp Rheumatol. 1992. 10:351–356.
84. Yonetsu K, Takagi Y, Sumi M, Nakamura T, Eguchi K. Sonography as a replacement for sialography for the diagnosis of salivary glands affected by Sjögren's syndrome. Ann Rheum Dis. 2002. 61:276–277.
85. Milic VD, Petrovic RR, Boricic IV, Radunovic GL, Pejnovic NN, Soldatovic I, Damjanov NS. Major salivary gland sonography in Sjögren's syndrome: diagnostic value of a novel ultrasonography score (0-12) for parenchymal inhomogeneity. Scand J Rheumatol. 2010. 39:160–166.
86. Salaffi F, Carotti M, Iagnocco A, Luccioli F, Ramonda R, Sabatini E, De Nicola M, Maggi M, Priori R, Valesini G, et al. Ultrasonography of salivary glands in primary Sjögren's syndrome: a comparison with contrast sialography and scintigraphy. Rheumatology (Oxford). 2008. 47:1244–1249.
87. McCurley TL, Collins RD, Ball E, Collins RD. Nodal and extranodal lymphoproliferative disorders in Sjogren's syndrome: a clinical and immunopathologic study. Hum Pathol. 1990. 21:482–492.
88. Bialek EJ, Jakubowski W, Zajkowski P, Szopinski KT, Osmolski A. US of the major salivary glands: anatomy and spatial relationships, pathologic conditions, and pitfalls. Radiographics. 2006. 26:745–763.
89. Nair JR, Nijjar M, Chiphang A, Binymin KA. Ultrasound-guided closed muscle biopsy: a useful tool for rheumatologists : case report: recurrent focal myositis of the gastrocnemius muscle. Rheumatol Int. 2011. doi: 10.1007/s00296-011-2229-8.
90. Micu MC, Serra S, Fodor D, Crespo M, Naredo E. Inter-observer reliability of ultrasound detection of tendon abnormalities at the wrist and ankle in patients with rheumatoid arthritis. Rheumatology (Oxford). 2011. 50:1120–1124.
91. Dougados M, Jousse-Joulin S, Mistretta F, d'Agostino MA, Backhaus M, Bentin J, Chalès G, Chary-Valckenaere I, Conaghan P, Etchepare F, et al. Evaluation of several ultrasonography scoring systems for synovitis and comparison to clinical examination: results from a prospective multicentre study of rheumatoid arthritis. Ann Rheum Dis. 2010. 69:828–833.
92. Filippucci E, Meenagh G, Delle Sedie A, Salaffi F, Riente L, Iagnocco A, Scirè CA, Montecucco C, Bombardieri S, Valesini G, et al. Ultrasound imaging for the rheumatologist: XX. sonographic assessment of hand and wrist joint involvement in rheumatoid arthritis: comparison between two- and three-dimensional ultrasonography. Clin Exp Rheumatol. 2009. 27:197–200.
93. Ju JH, Kang KY, Kim IJ, Yoon JU, Kim HY, Park SH. Three-dimensional ultrasonographic application for analyzing synovial hypertrophy of the knee in patients with osteoarthritis. J Ultrasound Med. 2008. 27:729–736.
94. Gao L, Parker KJ, Lerner RM, Levinson SF. Imaging of the elastic properties of tissue: a review. Ultrasound Med Biol. 1996. 22:959–977.
95. De Zordo T, Fink C, Feuchtner GM, Smekal V, Reindl M, Klauser AS. Real-time sonoelastography findings in healthy Achilles tendons. AJR Am J Roentgenol. 2009. 193:W134–W138.
96. De Zordo T, Lill SR, Fink C, Feuchtner GM, Jaschke W, Bellmann-Weiler R, Klauser AS. Real-time sonoelastography of lateral epicondylitis: comparison of findings between patients and healthy volunteers. AJR Am J Roentgenol. 2009. 193:180–185.
97. Sconfienza LM, Silvestri E, Bartolini B, Garlaschi G, Cimmino MA. Sonoelastography may help in the differential diagnosis between rheumatoid nodules and tophi. Clin Exp Rheumatol. 2010. 28:144–145.
98. Iagnocco A, Kaloudi O, Perella C, Bandinelli F, Riccieri V, Vasile M, Porta F, Valesini G, Matucci-Cerinic M. Ultrasound elastography assessment of skin involvement in systemic sclerosis: lights and shadows. J Rheumatol. 2010. 37:1688–1691.
99. Klauser A, De Zordo T, Feuchtner G, Sögner P, Schirmer M, Gruber J, Sepp N, Moriggl B. Feasibility of ultrasound-guided sacroiliac joint injection considering sonoanatomic landmarks at two different levels in cadavers and patients. Arthritis Rheum. 2008. 59:1618–1624.