Abstract
In this study, we explored the potentiality of human arginine decarboxylase (ADC) to enhance the survival of mesenchymal stem cells (MSCs) against unfavorable milieu of host tissues as the low survival of MSCs is the issue in cell transplantation therapy. To address this, human MSCs overexpressing human ADC were treated with H2O2 and the resultant intracellular events were examined. First, we examined whether human ADC is overexpressed in human MSCs. Then, we investigated cell survival or death related events. We found that the overexpression of human ADC increases formazan production and reduces caspase 3 activation and the numbers of FITC, hoechst, or propidium iodide positive cells in human MSCs exposed to H2O2. To elucidate the factors underlying these phenomena, AKT, CREB, and BDNF were examined. We found that the overexpression of human ADC phosphorylates AKT and CREB and increases BDNF level in human MSCs exposed to H2O2. The changes of these proteins are possibly relevant to the elevation of agmatine. Collectively, our data demonstrate that the overexpression of human ADC stimulates pro-survival factors to protect human MSCs against H2O2 toxicity. In conclusion, the present findings support that ADC can enhance the survival of MSCs against hostile environment of host tissues.
The arginine decarboxylase (ADC, EC 4.1.1.19) is an enzyme which synthesizes agmatine through L-arginine decarboxylation (1). ADC is ubiquitously found in most living organisms encompassing bacteria (2), plants (3), and animals (4). According to recent studies, ADC demonstrates various functions depending on each life form investigated. Some investigators report that ADC can enhance the resistance against environmental insults encompassing as high acidity and salt stress in bacteria and plants (5, 6). Others suggest that ADC exerts anti-proliferative effect in tumor cell (7) and neuroprotective effect against immobilization stress and chronic glucocorticoid exposure in animal (8, 9). As well, it is reported that mammalian ADC has the distinct characteristics compared to bacterial and plant ADC, in viewpoint of physiological responses and intracellular localization (10). Therefore, ADC is considered to be the multi-faceted protein in different life forms.
Mesenchymal stem cells (MSCs) are the typical adult stem cell which represents multi-potency, self-renewal, and broad tissue distribution (11). These MSCs possess biological and social advantages since they do not significantly produce immune rejection from host tissues and are free of many ethical problems (12, 13). Owing to these aspects, MSCs receive much attention as the promising therapy to treat several types of human diseases (14). However, there is a critical issue to be solved to maximize MSCs-derived therapeutic effects. After being transplanted, MSCs are unavoidably exposed to hostile conditions formed in host tissues (15), one of which is oxidative stress (16, 17). As a result, their survival is considerably low in transplanted sites, thereby reducing the therapeutic effects of MSCs transplantation. Therefore, a large number of researches have been performed to enhance the survival of MSCs against unfavorable milieu of host tissues (18-20).
In the present study, we explored the potentiality of ADC to facilitate MSCs survival against harsh surroundings of host tissues. As mentioned above, ADC renders life to struggle against endogenous and exogenous toxic insults. Based on this, it was hypothesized that ADC can improve MSCs survival against severe environment of host tissues. To address this, retroviral vector carrying human ADC gene was infected in human MSCs which were then exposed to H2O2. Then, several phenomena were examined including agmatine level, mitochondrial and plasma membrane integrity, caspase 3 activity, apoptotic DNA damage, and pro-survival factors such as AKT, CREB, and BDNF.
T-75 flasks and culture dishes (100 × 20 mm) were purchased from SPL Life Sciences (Seoul, Korea). 96-well plates were purchased from BD Biosciences (Franklin Lakes, NJ, USA). Dulbecco's modified Eagle medium (DMEM)-low glucose, fetal bovine serum (FBS), and penicillin/streptomycin were purchased from Life Technologies Korea (Seoul, Korea). Retroviral vector pLXSN expression system was purchased from Clontech (Palo Alto, CA, USA). Annexin V-FITC apoptosis detection kit, Lipofectamine 2000, G418, polybrene reagent, Hoechst 33258, propidium iodide, and 3-(4, 5-dimethylthiazol-2-yl)-2, 5-diphenyltetrazolium bromide (MTT) were purchased from Sigma (St. Louis, MO, USA). A rabbit polyclonal antibody to human ADC was purchased from ATGEN (Seoul, Korea). Polyvinylidene fluoride (PVDF) membrane was purchased from Millipore (Billerica, MA, USA). 4',6-diamidino-2-phenylindole (DAPI) was purchased from Vector Labs (Burlingame, CA, USA). Bicinchoninic acid (BCA) kit and enhanced chemiluminescent (ECL) Western blotting detection reagent were purchased from Thermo Fischer Scientific (Fremont, CA, USA). Rabbit polyclonal antibodies to AKT and cleaved caspase 3 and rabbit monoclonal antibodies to phosphorylated forms of AKT and CREB were purchased from Cell signaling technology (Beverly, MA, USA). A rabbit polyclonal antibody to BDNF, a mouse polyclonal antibody to β actin, and an anti-mouse or rabbit IgG antibody conjugated with horse radish peroxidasae (HRP) were purchased from Abcam (Cambridge, UK). A rabbit polyclonal antibody to caspase 3 and FITC conjugated second antibody were purchased from Millipore (Billerica, MA, USA). Unless specified, all other chemicals were purchased from Sigma (St. Louis, MO, USA).
Human bone marrow mesenchymal stem cells (MSCs) were obtained from Yonsei Cell Therapy center of Severance Hospital. Flow cytometry was performed to confirm the phenotypic characteristics of MSCs. MSCs were seeded in T-75 flasks and cultured at 37℃ in a humid incubator with 95% of O2 and 5% of CO2. The following day, the non-adherent cells were removed from the flasks and the adherent cells were fed with fresh Dulbecco's modified Eagle medium (DMEM)-low glucose supplemented with 10% FBS and 100 unit/mL penicillin and 100 µg/mL streptomycin. MSCs were then continuously maintained for 1-4 weeks. Medium was changed every second day. MSCs, of which passage number is over 5, were substantially used in the experiments. H2O2 was employed to reproduce oxidative stress condition, which is prevalent in damaged tissues (21). It has unique biochemical properties such as relatively long half-life and high solubility in both lipid and aqueous media (22). The control MSCs and MSCs infected with human ADC or empty vector were treated with 200 µM of H2O2 for 6 hr. This regimen for treatment was selected based on MTT data in the control MSCs exposed to H2O2, where the viability was approximately 50%-55%.
The retroviral vector carrying human ADC gene (gene bank accession number AY325129) was previously constructed by our group (23). For the experiments, PT67 packaging cells were infected with retroviral vector containing human ADC gene or empty pLXSN vector and then maintained in a humid incubator with 5% of CO2 at 37℃ for one week. Then, the medium containing retrovirus was filtered using 0.45 µm pore polysulfonic filter. For infection, human MSCs were cultured with the supernatants containing human ADC vector or empty pLXSN vector using polybrene reagent (6 µg/mL). After 24 hr of incubation, the medium was replaced by normal culture medium. The infected MSCs were maintained to perform the experiments for up to one week.
The expression of human ADC protein was investigated in control MSCs, pLXSN-MSCs and ADC-MSCs through immunocytochemistry. Samples were washed three times with PBS and fixed with 100% ethanol for 10 min on ice, and then washed three times with PBS. To increase permeability, samples were incubated with 0.025% Triton, and were blocked for 1 hr at room temperature with blocking solution. The samples were treated with anti-human ADC antibody for 3 hr at room temperature, which was diluted in the incubation buffer (1:500). Then, primary antibody was removed and samples were washed three times for 5 min each with PBS. Then, samples were treated with FITC labeled secondary antibody for 2 hr at room temperature, which was diluted in the incubation buffer (1:500). Finally, samples were stained with 4',6-diamidino-2-phenylindole (DAPI) 10 µL/mL for staining nucleus and then visualized under a confocal microscope (Zeiss LSM700; Zeiss, Thornwood, NY, USA).
In H2O2 treated and non-treated groups, 3-(4, 5-dimethylthiazol-2-yl)-2, 5-diphenyltetrazolium bromide (MTT) assay, which is based on the activity of mitochondrial dehydrogenase (24), was performed to estimate the number of viable cells. Briefly, MSCs were seeded (1.0 × 104 cells/200 µL) in 96-well plates. The cells were maintained in 96-well plates for 2 days and then were treated with H2O2 (200 µM for 6 hr). Then, the media were removed from each well and 200 µL of MTT solution was added into each well and the culture plates were incubated for 4 hr at 37℃. Then, dimethyl sulfoxide (DMSO) was added to dissolve formazan crystal in the mitochondria of living cell. The absorbance at 570 nm was measured with a spectrophotometer.
Hoechst 33258 and propidium iodide were employed to stain control MSCs, LXSN-MSCs and ADC-MSCs in this study. Hoechst 33258 enters both live and dead cells, but is distinctly accumulated in apoptotic nucleus. On the other hand, PI enters only dead cells and thus, is used to examine cell membrane integrity. All experimental groups were cultured in 24-well plates at a density of 3 × 104 cells/well for 2 days. After treatment of 200 µM of H2O2 for 6 hr, Hoechst 33258 was added to the culture medium (2-3 mg/mL) and then the samples were kept at 37℃ for 30 min. PI solution was then added (2-5 mg/mL) just before observation under the fluorescence microscope. PI/Hoechst-positive and PI/Hoechst-negatives were counted as dead or living cells, respectively.
The proportion of cells undergoing apoptosis was analyzed with Annexin V-FITC Apoptosis Detection Kit according to the manufacturer's protocol. In Brief, cells were harvested and resuspended in binding buffer (106 cells/mL), and then, cells were mixed with 5 µL of annexin V-FITC and 10 µL of propidium iodide (PI), followed by the incubation of cells at room temperature for 15 min in dark. Then, both FITC and PI fluorescence of cells were analyzed with flow cytometer.
The agmatine concentration in control MSCs and MSCs transfected with empty LXSN or human ADC gene carrying vector was measured by HPLC. The harvested cells were homogenized in 10% trichloracetic acid (TCA) and then, centrifuged at 12,000 rpm for 30 min. The supernatant was derivatized with o-phthaladehyde (OPA) and then, injected into the HPLC column with a fluorescence detector. The recovery of agmatine was calculated from the added external standard and the values are expressed as the µM concentration.
Cells from both H2O2 treated/non-treated groups were collected and the pellets were resuspended in lysis buffer (1% NP-40, 50 mM Tris [pH 7.5], 150 mM sodium chloride, 0.5% sodium deoxycholate, 0.1% sodium dodecyl sulfate (SDS), 1 mM EGTA, 5 mM EDTA, 1 mM PMSF, 1 mM Na orthovanadate, 5 mM Na fluoride, 1 µg/mL pepstatin, 2 µg/mL aprotinin, and 5 µg/mL leupeptin) for 2 hr and then centrifuged at 13,200 rpm for 30 min at 4℃. The supernatants were collected and protein concentration was determined with bicinchoninic acid (BCA) assay. The equal amounts of proteins (40 µg) were separated on 10%-12% SDS-polyacrylamide gels using an electrophoresis unit maintained at the constant voltage (100 V). Separated proteins were then electrotransfered onto PVDF membrane. Later, membranes were incubated with 0.1% bovine serum albumin (BSA) in Tris-buffered saline and 0.3% Tween-20 (TBS-T) to block nonspecific binding for 1 hr at room temperature. After blocking, the membranes were treated with specific primary antibodies including anti-phospho AKT, anti-phospho CREB, anti-caspase 3, anti-cleavage caspase 3, and anti-BDNF at 4℃. All of primary antibodies were diluted (1:1,000) in incubation buffers for treatment. After treatment, membranes were washed 3 times with TBST for every 5 min. Then, membranes were treated for 1 hr at room temperature with anti-mouse or rabbit IgG antibody conjugated with horse radish peroxidase (HRP) in the incubation buffers (1:3,000). Finally, blots were rinsed and proteins of interest were detected with enhanced chemiluminescent (ECL) kit and visualized with LAS 4000 image analyzer. Protein bands detected were quantified as the ratio of the target protein to beta actin using Image J software (National Institutes of Health, Bethesda, MD, USA).
The data are presented as mean ± SD. Statistical analysis of the data was made by one-way ANOVA using SPSS statistical package version 12.0. P values less than 0.05 were considered to be statistically significant and the significant differences were calculated at 95% confidence interval. All experiments were independently carried out at least three times.
The retroviral vectors carrying human arginine decarboxylase (ADC), which was previously constructed by our group (23), were employed to infect human mesenchymal stem cells (MSCs). Western blotting data show that ADC protein was greatly elevated in MSCs infected with vectors containing ADC gene (Fig. 1A). In particular, the increase of approximately 0.7 or 2.5 fold was respectively observed in those MSCs, compared with LXSN-MSCs under normal or H2O2 exposure condition (Fig. 1B). And the level of agmatine synthesized by ADC was approximately 2 fold raised in MSCs infected with vectors containing ADC gene under H2O2 exposure (Fig. 1C), implying the overexpressed ADC involved this elevation in those MSCs.
The overexpressed human arginine decarboxylase (ADC) preserved mitochondrial integrity in human mesenchymal stem cells (MSCs) treated with H2O2. The level of formazan was 56.4% ± 0.9% and 52.8% ± 1.5% of untreated control MSCs in control MSCs or LXSN-MSCs exposed to H2O2, respectively (Fig. 2A). However, its level significantly increased into 69.7% ± 2.7% of untreated control MSCs in ADC-MSCs treated with H2O2 (Fig. 2A). As well, the overexpressed ADC saved plasma membrane integrity in human MSCs exposed to H2O2. The propidium iodide (PI) positive cells clearly appeared in control MSCs and LXSN-MSCs treated with H2O2 (Fig. 2B). However, this damage was significantly alleviated in ADC-MSCs. In fact, the numbers of PI positive cells were reduced into 32.9% ± 1.3% of control MSCs in ADC-MSCs under H2O2 exposure. This protective effect of the overexpressed ADC was further investigated with FACS analysis. The distribution of FITC positive cells was obviously reduced in ADC-MSCs compared to control and LXSN MSCs under H2O2 exposure (Fig. 2C).
The overexpressed human arginine decarboxylase (ADC) suppressed apoptotic events in human mesenchymal stem cells (MSCs) exposed to H2O2. The level of pro-caspase 3 was not quite different between control MSCs and ADC-MSCs under normal or H2O2 exposure (Fig. 3A). However, the level of cleaved caspase 3 increased in control MSCs treated with H2O2, whereas its level obviously decreased in ADC-MSCs exposed to H2O2 (Fig. 3A). The similar trend also appeared in nuclear integrity. No difference of nuclear morphology was found between control MSCs and ADC-MSCs under normal condition (Fig. 3B). However, a lot of condensed nuclei were observed in control MSCs exposed to H2O2, while their numbers apparently decreased in ADC-MSCs exposed to H2O2 (Fig. 3B). This protective effect of the overexpressed human arginine decarboxylase (ADC) was further substantiated in human mesenchymal stem cells (MSCs) treated with H2O2. That is, the expressions of pro-survival proteins P-AKT, P-CREB, and BDNF obviously increased in ADC-MSCs, compared with control MSCs under H2O2 exposure (Fig. 4). However, their expressions were not differed between control MSCs and ADC-MSCs under normal condition (Fig. 4).
The current findings demonstrate that the overexpression of human arginine decarboxylase (ADC) stimulates pro-survival factors such as AKT, CREB, and BDNF to protect human mesenchymal stem cells (MSCs) against H2O2 toxicity. As commonly known, the low survival of MSCs transplanted is attributed to the unfavorable milieu of host tissues (25, 26). Accordingly, the improvement of MSCs survival following transplantation becomes the fundamental goal to secure the efficacy of cell therapy (27, 28). In this perspective, ADC seems to be applicable because it exerts several effects such as resistance against environmental insults (5, 6), anti-proliferation of tumors (7), and neuroprotection (9) in living organisms. In the current study, the potentiality of ADC was explored to enhance the survival of MSCs against hostile environment of host tissues.
The retroviral vector expression system is an efficient tool to deliver genes of interest into target cells (29, 30). In this study, human MSCs (ADC-MSCs) were infected with the retroviral vector (LXSN) containing human ADC gene to overexpress human ADC protein. Western blotting was carried out to confirm the overexpression of human ADC protein (Fig. 1A). The data indicate that human ADC protein level is higher in ADC-MSCs, compared to control MSCs and LXSN-MSCs with or without H2O2 treatment, respectively (Fig. 1B). It is interesting that ADC protein is up-regulated even in control MSCs exposed to H2O2 although its level is lower than that of ADC-MSCs. Similarly, the increase of ADC protein has been reported in neurons under stress condition (8, 9). Thus, it is presumable that ADC plays the certain roles in the amelioration or exacerbation of stresses. To further confirm the overexpression of ADC, agmatine was analyzed with HPLC. As mentioned earlier, agmatine production is mediated by ADC (1). The data show that the robust elevation of agmatine was found in ADC-MSCs exposed to H2O2 (Fig. 1C). Overall, the current data demonstrated that human ADC was successfully overexpressed through retroviral vector expression system in human MSCs.
The present findings indicated that the overexpression of human ADC supports mitochondrial function (Fig. 2A) and suppresses apoptotic events (Fig. 3) in MSCs exposed to H2O2. MTT data show that formazan formation was approximately half of untreated group in control MSCs or LXSN-MSCs exposed to H2O2, respectively (Fig. 2A). However, formazan formation was significantly raised in ADC-MSCs, compared with untreated group under H2O2 exposure (Fig. 2A). Therefore, these data imply that the overexpression of human ADC preserves mitochondrial integrity in MSCs against H2O2 toxicity. The fluorescence micrographs show that the numbers of propidium iodide positive cells with condensed nuclei were elevated in control MSCs and LXSN-MSCs after H2O2 treatment (Fig. 2B, 3B). However, this pathology was significantly attenuated in ADC-MSCs. The similar phenomenon was also found in FACS analysis. The distribution of FITC fluorescent cells was greatly decreased in ADC-MSCs, compared with control and LXSN-MSCs under H2O2 exposure (Fig. 2C), suggesting that the overexpressed ADC inhibited apoptotic death of MSCs. To understand the implication of apoptotic protein in this pathology, caspase 3 into which apoptotic signals are converged (31), was examined. As expected, caspase 3 was activated in control MSCs and LXSN-MSCs exposed to H2O2. However, its activation was obviously inhibited in ADC-MSCs exposed to H2O2 (Fig. 3A). Therefore, current data suggest that the overexpression of human ADC suppresses the pro-apoptotic executioner in human MSCs treated with H2O2.
The current findings show that the overexpression of human ADC increases the phosphorylation of v-akt murine thymoma viral oncogene homolog (AKT) and cAMP response element binding protein (CREB) and the protein level of brain-derived neurotrophic factor (BDNF) in human MSCs exposed to H2O2 (Fig. 4). AKT (also known as PKB) is a serine/threonine protein kinase that plays the important roles in cell survival (32). Once activated, it phosphorylates CREB (33), which in turn, switches on the expression of BDNF (34). Conversely, BDNF per se phosphorylates AKT and CREB to promote cell survival against the insults (35). In support, all of AKT, CREB, and BDNF have shown to suppress the activation of caspase 3 and the occurrence of apoptotic death in various pathologic conditions (36-38). Importantly, one study demonstrates that BDNF is produced in MSCs (39). Therefore, considering all of these, it is probable that AKT, CREB, and BDNF pathway might contribute to the enhancement of survival in ADC-MSCs exposed to H2O2. The supporting explanation of it may be relevant to agmatine, since AKT is activated by agmatine (40). In this study, agmatine was greatly raised in ADC-MSCs exposed to H2O2 (Fig. 1C) and thus, this elevation of agmatine has supposedly influenced AKT, CREB, and BDNF. However, the details of underlying mechanism remain to be elucidated. Overall, current data suggest that the overexpression of human ADC activates cell survival-related proteins to enhance the survival of human MSCs against H2O2 toxicity.
Taken together, our data suggest that the overexpression of human ADC stimulates pro-survival factors to protect human MSCs against H2O2 toxicity. In conclusion, the present findings support the idea that human ADC is able to enhance the survival of human MSCs against hostile environment of host tissues.
Figures and Tables
Fig. 1
The retroviral overexpression of human ADC in human MSCs and the measurement of agmatine with HPLC. (A) Bands of human ADC protein in control MSCs, LXSN-MSCs, and ADC-MSCs with or without H2O2 treatment. The photograph is a representative from 4 blots with the similar results. (B) Quantification of ADC protein bands demonstrated in Fig. 1A. Asterisks indicate the significant difference at P value less than 0.05 when compared to control and LXSN-MSCs. Data represent the mean ± standard deviation (SD) for 4 independent experiments. (C) Quantification of agmatine in control MSCs, LXSN-MSCs, and ADC-MSCs with or without H2O2 treatment. Asterisk indicates the significant difference at P value less than 0.05 when compared to control MSCs exposed to H2O2. Data represent the mean ± standard deviation (SD) for 4 independent experiments.
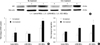
Fig. 2
Cell viability in control MSCs, LXSN-MSCs, and ADC-MSCs following H2O2 treatment. (A) Formazan formation. (B) Cell membrane integrity. Each photograph is a representative from 4 fields of microscope. The arrow indicates cells, where plasma membrane is damaged. Scale bar represents 200 µm. (a) Control MSCs, (b) LXSN-MSCs, (c) ADC-MSCs. (d) The quantitative graph showing the total number of propidium iodide (PI) positive cells in all experimental groups. Asterisk indicates the significant difference at P value less than 0.05 when compared to LXSN-MSCs exposed to H2O2. (C) Flow cytometric analysis. The data show that the distribution of cells undergoing apoptosis is reduced in ADC-MSCs compared to control MSCs and LXSN-MSCs under H2O2 exposure. Each panel is a representative from 4 independent experiments with the similar results.
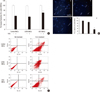
Fig. 3
Apoptotic events in control MSCs, LXSN-MSCs, and ADC-MSCs following H2O2 treatment. (A) Caspase 3 activation. Western blotting data show that H2O2 treatment increases active form of caspase 3 in control MSCs and LXSN-MSCs. However, such an activation of caspase 3 was alleviated in ADC-MSCs exposed to H2O2. The less or no activation of caspase 3 was observed in control MSCs, LXSN-MSCs, and ADC-MSCs without H2O2 treatment. Each photograph is a representative from 4 blots with the similar results. (B) Nuclear morphology. Each photograph is a representative from 4 fields of microscope. Scale bar represents 200 µm. The data indicate that nuclear condensation (arrow) is lessened in ADC-MSCs compared to control MSCs and LXSN-MSCs following H2O2 treatment.
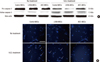
Fig. 4
AKT, CREB, and BDNF in control MSCs, LXSN-MSCs, and ADC-MSCs following H2O2 treatment. Western blotting was performed to examine AKT p-AKT, CREB, p-CREB, and BDNF in control MSCs, LXSN-MSCs, and ADC-MSCs before and after H2O2 treatment. Results show that the levels of p-AKT, p-CREB and BDNF were greatly increased in ADC-MSCs following H2O2 treatment, compared to those of control MSCs and LXSN-MSCs. Each photograph is a representative from 4 blots with the similar results.
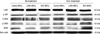
References
1. Morris SM Jr. Enzymes of arginine metabolism. J Nutr. 2004. 134:2743S–2747S.
2. Cunin R, Glansdorff N, Piérard A, Stalon V. Biosynthesis and metabolism of arginine in bacteria. Microbiol Rev. 1986. 50:314–352.
3. Borrell A, Culianez-Macia FA, Altabella T, Besford RT, Flores D, Tiburcio AF. Arginine decarboxylase is localized in chloroplasts. Plant Physiol. 1995. 109:771–776.
4. Morrissey J, McCracken R, Ishidoya S, Klahr S. Partial cloning and characterization of an arginine decarboxylase in the kidney. Kidney Int. 1995. 47:1458–1461.
5. Kasinathan V, Wingler A. Effect of reduced arginine decarboxylase activity on salt tolerance and on polyamine formation during salt stress in Arabidopsis thaliana. Physiol Plant. 2004. 121:101–107.
6. Alvarez-Ordóñez A, Fernández A, Bernardo A, López M. Arginine and lysine decarboxylases and the acid tolerance response of Salmonella Typhimurium. Int J Food Microbiol. 2010. 136:278–282.
7. Wolf C, Brüss M, Hänisch B, Göthert M, von Kügelgen I, Molderings GJ. Molecular basis for the antiproliferative effect of agmatine in tumor cells of colonic, hepatic, and neuronal origin. Mol Pharmacol. 2007. 71:276–283.
8. Zhu MY, Wang WP, Huang J, Regunathan S. Chronic treatment with glucocorticoids alters rat hippocampal and prefrontal cortical morphology in parallel with endogenous agmatine and arginine decarboxylase levels. J Neurochem. 2007. 103:1811–1820.
9. Zhu MY, Wang WP, Huang J, Feng YZ, Regunathan S, Bissette G. Repeated immobilization stress alters rat hippocampal and prefrontal cortical morphology in parallel with endogenous agmatine and arginine decarboxylase levels. Neurochem Int. 2008. 53:346–354.
10. Regunathan S, Reis DJ. Characterization of arginine decarboxylase in rat brain and liver: distinction from ornithine decarboxylase. J Neurochem. 2000. 74:2201–2208.
11. Parekkadan B, Milwid JM. Mesenchymal stem cells as therapeutics. Annu Rev Biomed Eng. 2010. 12:87–117.
12. Ryan JM, Barry FP, Murphy JM, Mahon BP. Mesenchymal stem cells avoid allogeneic rejection. J Inflamm (Lond). 2005. 2:8.
13. Strauer BE, Kornowski R. Stem cell therapy in perspective. Circulation. 2003. 107:929–934.
14. Hodgkinson CP, Gomez JA, Mirotsou M, Dzau VJ. Genetic engineering of mesenchymal stem cells and its application in human disease therapy. Hum Gene Ther. 2010. 21:1513–1526.
15. Zhu W, Chen J, Cong X, Hu S, Chen X. Hypoxia and serum deprivation-induced apoptosis in mesenchymal stem cells. Stem Cells. 2006. 24:416–425.
16. Yao EH, Yu Y, Fukuda N. Oxidative stress on progenitor and stem cells in cardiovascular diseases. Curr Pharm Biotechnol. 2006. 7:101–108.
17. Ko E, Lee KY, Hwang DS. Human umbilical cord blood-derived mesenchymal stem cells undergo cellular senescence in response to oxidative stress. Stem Cells Dev. 2012. 21:1877–1886.
18. Chen HY, Zhang X, Chen SF, Zhang YX, Liu YH, Ma LL, Wang LX. The protective effect of 17β-estradiol against hydrogen peroxide-induced apoptosis on mesenchymal stem cell. Biomed Pharmacother. 2012. 66:57–63.
19. Li S, Bian H, Liu Z, Wang Y, Dai J, He W, Liao X, Liu R, Luo J. Chlorogenic acid protects MSCs against oxidative stress by altering FOXO family genes and activating intrinsic pathway. Eur J Pharmacol. 2012. 674:65–72.
20. Ebert R, Ulmer M, Zeck S, Meissner-Weigl J, Schneider D, Stopper H, Schupp N, Kassem M, Jakob F. Selenium supplementation restores the antioxidative capacity and prevents cell damage in bone marrow stromal cells in vitro. Stem Cells. 2006. 24:1226–1235.
21. Giustarini D, Dalle-Donne I, Tsikas D, Rossi R. Oxidative stress and human diseases: origin, link, measurement, mechanisms, and biomarkers. Crit Rev Clin Lab Sci. 2009. 46:241–281.
22. Satoh T, Sakai N, Enokido Y, Uchiyama Y, Hatanaka H. Free radical-independent protection by nerve growth factor and Bcl-2 of PC12 cells from hydrogen peroxide-triggered apoptosis. J Biochem. 1996. 120:540–546.
23. Moon SU, Kwon KH, Kim JH, Bokara KK, Park KA, Lee WT, Lee JE. Recombinant hexahistidine arginine decarboxylase (hisADC) induced endogenous agmatine synthesis during stress. Mol Cell Biochem. 2010. 345:53–60.
24. Denizot F, Lang R. Rapid colorimetric assay for cell growth and survival: modifications to the tetrazolium dye procedure giving improved sensitivity and reliability. J Immunol Methods. 1986. 89:271–277.
25. Müller-Ehmsen J, Krausgrill B, Burst V, Schenk K, Neisen UC, Fries JW, Fleischmann BK, Hescheler J, Schwinger RH. Effective engraftment but poor mid-term persistence of mononuclear and mesenchymal bone marrow cells in acute and chronic rat myocardial infarction. J Mol Cell Cardiol. 2006. 41:876–884.
26. Burst VR, Gillis M, Pütsch F, Herzog R, Fischer JH, Heid P, Müller-Ehmsen J, Schenk K, Fries JW, Baldamus CA, et al. Poor cell survival limits the beneficial impact of mesenchymal stem cell transplantation on acute kidney injury. Nephron Exp Nephrol. 2010. 114:e107–e116.
27. Mohammadzadeh M, Halabian R, Gharehbaghian A, Amirizadeh N, Jahanian-Najafabadi A, Roushandeh AM, Roudkenar MH. Nrf-2 overexpression in mesenchymal stem cells reduces oxidative stress-induced apoptosis and cytotoxicity. Cell Stress Chaperones. 2012. 17:553–565.
28. Li Z, Wei H, Liu X, Hu S, Cong X, Chen X. LPA rescues ER stress-associated apoptosis in hypoxia and serum deprivation-stimulated mesenchymal stem cells. J Cell Biochem. 2010. 111:811–820.
29. Ausubel FM, Brent R, Kingston RE, Moore DD, Seidman JG, Smith JA, Struhl K. Current protocols in molecular biology. 1995. New York: John Wiley & Sons.
30. Coffin JM, Hughes SH, Varmus HE. Retroviruses. 1997. Plainview: Cold Spring Harbor Laboratory Press.
31. Porter AG, Jänicke RU. Emerging roles of caspase-3 in apoptosis. Cell Death Differ. 1999. 6:99–104.
32. Manning BD, Cantley LC. AKT/PKB signaling: navigating downstream. Cell. 2007. 129:1261–1274.
33. Du K, Montminy M. CREB is a regulatory target for the protein kinase Akt/PKB. J Biol Chem. 1998. 273:32377–32379.
34. Riccio A, Ahn S, Davenport CM, Blendy JA, Ginty DD. Mediation by a CREB family transcription factor of NGF-dependent survival of sympathetic neurons. Science. 1999. 286:2358–2361.
35. Xia Y, Wang CZ, Liu J, Anastasio NC, Johnson KM. Brain-derived neurotrophic factor prevents phencyclidine-induced apoptosis in developing brain by parallel activation of both the ERK and PI-3K/Akt pathways. Neuropharmacology. 2010. 58:330–336.
36. Matsui T, Li L, del Monte F, Fukui Y, Franke TF, Hajjar RJ, Rosenzweig A. Adenoviral gene transfer of activated phosphatidylinositol 3'-kinase and Akt inhibits apoptosis of hypoxic cardiomyocytes in vitro. Circulation. 1999. 100:2373–2379.
37. Nagai-Kusuhara A, Nakamura M, Mukuno H, Kanamori A, Negi A, Seigel GM. cAMP-responsive element binding protein mediates a cGMP/protein kinase G-dependent anti-apoptotic signal induced by nitric oxide in retinal neuro-glial progenitor cells. Exp Eye Res. 2007. 84:152–162.
38. Makar TK, Trisler D, Sura KT, Sultana S, Patel N, Bever CT. Brain derived neurotrophic factor treatment reduces inflammation and apoptosis in experimental allergic encephalomyelitis. J Neurol Sci. 2008. 270:70–76.
39. Chen X, Li Y, Wang L, Katakowski M, Zhang L, Chen J, Xu Y, Gautam SC, Chopp M. Ischemic rat brain extracts induce human marrow stromal cell growth factor production. Neuropathology. 2002. 22:275–279.
40. Santhanam AV, Viswanathan S, Dikshit M. Activation of protein kinase B/Akt and endothelial nitric oxide synthase mediates agmatine-induced endothelium-dependent relaxation. Eur J Pharmacol. 2007. 572:189–196.