Abstract
Glial cells play a critical role in morphine tolerance, resulting from repeated administration of morphine. Both the development and the expression of tolerance are suppressed by the analgesic lamotrigine. This study investigated the relationship between the ability of lamotrigine to maintain the antinociceptive effect of morphine during tolerance development and glial cell activation in the spinal cord. In a rat model, morphine (15 µg) was intrathecally injected once daily for 7 days to induce morphine tolerance. Lamotrigine (200 µg) was co-administered with morphine either for 7 days or the first or last 3 days of this 7 day period. Thermal nociception was measured. OX-42 and GFAP immunoreactivity, indicating spinal microglial and astrocytic activation were evaluated on day 8. Tolerance developed after 7 days of intrathecal morphine administration; however, this was completely blocked and reversed by co-administration of lamotrigine. When lamotrigine was coinjected with morphine on days 5-7, the morphine effect was partially restored. Glial cell activation increased with the development of morphine tolerance but was clearly inhibited in the presence of lamotrigine. These results suggest that, in association with the suppression of spinal glial cell activity, intrathecally coadministered lamotrigine attenuates antinociceptive tolerance to morphine.
Morphine is the most widely used narcotic analgesic for relieving severe or chronic pain but its repeated administration leads to a characteristic tolerance (1). Although the clinical importance of opioid tolerance is controversial (2), laboratory studies of this phenomenon have advanced our understanding of analgesic mechanisms in general. On a cellular level, chronic opioid administration was shown to cause spinal changes involving the translocation and activation of protein kinase C and the production of nitric oxide (NO) (3). Additional mechanisms underlying opioid tolerance include the modulation of the N-methyl-d-aspartate (NMDA) (3) and 2-amino-3-hydroxy-5-methyl-4-isoxazole-proprionic acid (AMPA)/kainate receptors (4), and changes in the activities of dynorphin (5), calcitonin gene-related peptide (6), and cyclooxygenase (7).
Glial cells were originally considered to play merely a supporting role in the central nerve system (CNS) but they were eventually recognized as being critical to the development of morphine tolerance (8). Chronic morphine treatment has been shown to increase not only the reactivity (9) but also the migration (10) of spinal microglial cells, both of which are causally related to pathologic pain states since pharmacologic inhibition of glial activation prevents the development of pain (11). Thus, targeting glial cell activity is a promising strategy for the treatment of morphine insensitivity, including neuropathic pain, and the prevention of tolerance development.
Co-administration of a second drug with an opioid enhances the latter's antinociceptive effect and attenuates the development of tolerance (12). Lamotrigine was originally designed as an anticonvulsant but was subsequently shown to have analgesic activity as well, such that it is now used to treat different types of neuropathic pain. The precise mechanism of action of lamotrigine is unknown but in in vitro pharmacologic studies it was shown to inhibit voltage-sensitive sodium channels, leading to a stabilization of neuronal membranes and thereby modulating the presynaptic transmitter release of excitatory amino acids such as glutamate and aspartate (13). Intrathecal administration of 100 µg of lamotrigine produced a dose-dependent antihyperalgesic effect in a neuropathic model (chronic constriction injury) of hyperalgesia (14). Suppressive effects of lamotrigine on the development and expression of tolerance to morphine-induced antinociception in mice has also been demonstrated (15).
Pre- and post-synaptic neuronal membranes as well as glial cells express several different classes of glutamate receptors (16). An effect of lamotrigine on glial cells is suggested by its ability to reduce the extent of inflammation, neuronal damage, and death following epilepsy and/or traumatic brain injury (16-19). However, whether intrathecal lamotrigine is capable of altering glial cell activation in morphine-tolerant rats has not been examined.
In the present study, we asked whether intrathecally-administered lamotrigine maintains the antinociceptive effect of morphine during tolerance development by suppressing glial cell activation in the spinal cord and thus whether it could prevent the development and expression of chronic morphine tolerance.
The experiments were conducted in male Sprague-Dawley rats (weight 200-250 g) in a study performed using a protocol approved by the animal use and care committee. The animals were housed individually in a temperature-controlled vivarium and allowed to acclimate for 3 days in a 12/12 hr light/dark cycle.
For intrathecal drug administration, the rats were chronically implanted with catheters as previously described (20). Briefly, under sevoflurane anesthesia, the rats were placed in a stereotaxic head holder and the occipital muscles were separated from their attachment points, retracting the muscles caudally to expose the cisternal membrane at the base of the skull. Intrathecal catheters (PE-10 polyethylene tubing, Becton Dickinson, Sparks, MD, USA) were passed caudally from the cisterna magna to the spinal cord level of lumbar enlargement and then externalized through the skin. Proper intrathecal location was confirmed by a temporary motor block of both hind-limbs after the injection of 2% lidocaine 10 µL, followed by saline. Only animals with no evidence of neurologic deficit after the operation were studied. At least a 5 day recovery period was allowed before the animals were used in experiments.
Morphine sulfate (molecular weight, 668.76), lamotrigine (molecular weight, 256.09), and dimethyl sulfoxide (DMSO, minimum 99.5%) were purchased from Sigma, St. Louis, MO, USA. Lamotrigine was dissolved in DMSO, and morphine in 0.9% saline. The doses for intrathecal lamotrigine were selected according to a previous study (14, 21). All drugs were administered intrathecally with a microinjection syringe (Microliter™ #702, Hamilton Co., Reno, NV, USA) over a 60 sec interval in a volume of 10 µL, followed by a 10 µL saline flush. Investigators were blind to the administered drug.
Thermal nociception was assessed by measuring the latency of paw withdrawal in response to a radiant heat source (22). Rats were housed individually in Plexiglas chambers on an elevated glass platform, under which a radiant heat source (Ugo Basile, Milan, Italy) was applied to the plantar surface of the hind-paw through a glass plate maintained at 30℃. The heat source was turned off when the rat lifted its foot, and the time from the onset of radiant heat application to hind-paw withdrawal, defined as paw withdrawal latency (PWL), was recorded. The heat was maintained at a constant intensity and resulted in a stable PWL of approximately 10-12 sec in normal rats. A 20 sec cut-off avoided tissue damage. PWL was measured three times at 5 min intervals and the average values were calculated. The measured PWL was converted to a percentage of maximum possible effect (%MPE) according to the formula: %MPE = ([post-drug value-baseline value]/[cut-off value-baseline value]) × 100%.
Single intrathecal doses of 5 µg of morphine, 200 µg of lamotrigine, and a combination of both drugs were studied (n = 6 per subgroup). Measurements were taken before and 15, 30, 45, 60, 90, 120, and 180 min after an intrathecal dose of the drug(s).
Rats in the morphine group (M1-7 group, n = 6) received intrathecal injections of 15 µg of morphine once daily for 7 days. This dose was previously shown to produce tolerance over 7 days following initial maximal antinociception (23). To evaluate the effects of lamotrigine on the development of morphine tolerance, a dose of 200 µg was intrathecally co-injected with 15 µg of morphine once daily for 7 days (L1-7 group, n = 6). The offset of lamotrigine effect on morphine tolerance was determined by co-injecting the two drugs on days 1-3 followed by the daily injection of morphine alone on days 4-7 (L1-3 group, n = 6). Behavioral testing was performed before and 30 min after the drug administration.
Intrathecal morphine (15 µg) was given once daily for 4 days to induce tolerance. On the following 3 days, intrathecal lamotrigine (200 µg) was co-administered with the daily dose of morphine (L5-7 group, n = 6).
To evaluate microglial and astrocytic activation in the spinal cord after the development of morphine tolerance, the cells were stained with the microglial marker OX-42 and glial fibrillary acidic protein (GFAP), respectively. After the last behavioral assessment, the rats were deeply anesthetized with sevoflurane and perfused intracardially with heparinized normal saline, followed by 4% paraformaldehyde in phosphate-buffered saline (PBS) on day 8. The spinal cord around lumbar vertebrae L5 and L6 was removed and then post-fixed in the same fixative at room temperature for 4 hr. All specimens were cryoprotected in 30% (w/v) sucrose. The spinal cords were cut transversely using a cryostat into 20 µm floating sections, washed first in PBS and then 2 × 10 min in PBS containing 0.3% Triton X-100 (PBST), and finally treated with 0.3% hydrogen peroxide in PBST for at least 15 min to exhaust endogenous hydrogen peroxidase. Non-specific binding was blocked by incubating the sections in 3% normal goat serum plus 2% bovine serum albumin in PBST for 1 hr. Next, the sections were incubated at room temperature overnight with primary mouse monoclonal antibody (anti-OX-42, 1 µg/mL; Serotec, Oxford, UK) in PBST plus 3% normal goat serum. On the following day, they were washed 3 × 10 min with PBST and then incubated with goat anti-mouse biotinylated secondary antibody (1:1,000; Chemicon, Temecula, CA, USA) for 1 hr at room temperature. Following three washes with PBST, the floating sections were incubated for 1 hr with a 1:160 dilution of an avidin-biotin horseradish peroxidase complex (ABC complex; Pierce, IL, USA) in PBST. Antigens were visualized by treating the stained sections with a 1:1 solution of ammonium nickel sulfate (30 mg/mL in 0.1 M sodium acetate, pH 6.0) and diaminobenzidine (4 mg/mL in PBS) in the presence of 0.01% hydrogen peroxide. The sections were then spread flat on slides, air-dried, rinsed with distilled water for 1 min, and dehydrated first through an ethanol gradient (70% once, 95% twice, and 100% twice, 1.5 min each) and then in xylene (2 × 3 min). The processed sections were cover slipped with Permount mounting medium (Merck, Darmstadt, Germany). Images were acquired with a DXM1200 digital camera connected to an Eclipse E800 light microscope (Nikon, Melville, NY, USA).
Glial responses were assessed in three randomly chosen sections prepared from the L5 spinal cord segments. The number of immunoreactive cells in the medial superficial dorsal horn (laminae I-III), where most of the positive cells were found after the treatments, were counted. The same microscopic field was photographed at a magnification of 100 × using a digital camera. The data were expressed as the number of positive cells/area/spinal section.
The behavioral data were expressed as the mean ± SEM. Statistical analyses were carried out using the one-way analysis of variance (ANOVA), comparing the treatment groups to the control group at the same time point, followed by a Tukey's test for multiple comparisons. The significance of the differences in the responses of the treatment groups compared to the respective preoperative baseline values was determined using one-way repeated-measures ANOVA followed by a Tukey's test for multiple comparisons. Statistical evaluation was performed with SigmaPlot® software version 11 (Systat Software, Inc., San Jose, CA, USA). P < 0.05 was considered statistically significant.
All the rats remained healthy and continued to gain weight throughout the experimental period. No motor dysfunction was observed in the rats that received morphine alone or in combination with lamotrigine.
In this study design, 54 rats were used in total (Table 1). In the study 1, acute effects of lamotrigine on morphine antinociception were investigated in 24 rats (six rats per group × four groups). In the study 2, effects of lamotrigine on the development of morphine tolerance were analyzed in 24 rats (six rats per group × four groups). In the study 3, effects of lamotrigine on established morphine tolerance was analyzed in 6 rats (six rats per group × one group).
Submaximal doses of intrathecal morphine (5 µg) produced peak antinociceptve effect against thermal stimulation (55.4 ± 5.2 %MPE) 30 min after intrathecal administration of morphine (Fig. 1). A 200 µg intrathecal dose of lamotrigine alone likewise produced peak antinociceptive effect against thermal stimulation (32.0 ± 5.7 %MPE) 30 min after administration (Fig. 1). When the two drugs were injected together at these doses, an increase in antinociception (98.9 ± 1.3 %MPE) peaking 30 min later was recorded. The combination of morphine and lamotrigine resulted in significantly larger antinociceptive responses than morphine alone (P<0.001) (Fig. 1). All responses returned to baseline by 180 min after drug injection. Visual inspection of the treated animals revealed no signs of motor impairment. Intrathecally administered vehicle (10% DMSO) had no effect (data not shown).
In the M1-7 group, the administration of intrathecal morphine (15 µg) produced maximal antinociception against thermal stimulation on day 1 (99.7±4.2 %MPE), with the effect decreasing to baseline levels by day 7 (18.6±4.8 %MPE). In the L1-7 group, the co-administration of intrathecal morphine (15 µg) and intrathecal lamotrigine (200 µg) completely blocked the decrease in morphine's effect throughout the entire 7 day period (90.1±3.6 %MPE on day 7, Fig. 2). In a subsequent experiment (L1-3 group), in which lamotrigine was co-administered with morphine only on days 1-3, maximal antinociception with morphine was still present on day 4 (92.1±3.2 %MPE) but the effect decreased over days 5-7 (55.6±3.7 %MPE on day 7, Fig. 2).
In the L5-7 group, the chronic administration of morphine alone on days 1-4 resulted in a decrease in antinociception against thermal stimulation (54.7±4.5 %MPE on day 4, Fig. 2). However, the addition of lamotrigine on days 5-7 partially restored morphine's effect (68.7±5.1 %MPE on day 5, P=0.026 vs M1-7 group) such that antinociception on days 5-7 was significantly greater than obtained with morphine alone (P=0.042 on day 6, P=0.003 on day 7) (Fig. 2).
The immunoreactivity (IR) of OX-42 in the L5 spinal cord is described in the Fig. 3. In the control group, there were 31.5±2.3 OX-42-positive cells (under 200×magnification), whereas with once-daily intrathecal injections of 15 µg of morphine, OX-42 IR significantly increased to 257.7±13.3 cells (P<0.001 vs control group) after 7 days (M1-7 group). These findings implicate microglial activation in the effects of morphine. Following the co-administration of 200 µg of lamotrigine for 7 days (L1-7 group), there were significantly fewer stained cells (194.2±11.2, P=0.013 vs M1-7 group). When 200 µg of lamotrigine was coinjected with morphine on days 1-3 followed by daily morphine alone on days 4-7 (L1-3 group), there were 208.5±13.9 OX-42-positive cells. Conversely, the coadministration of 200 µg of lamotrigine and 15 µg of morphine on days 5-7 (L5-7 group) resulted in 168.3±10.4 OX-42-positive cells. In these three groups (L1-7, L1-3, and L5-7), although the numbers of OX-42-positive cells were significantly lower than those of the M1-7 group (P=0.013, P=0.045, P<0.001), they were significantly higher (P<0.001) than those of the control group.
The IR of GFAP in the L5 spinal cord is explained in the Fig. 4. In the control group, there were 39.3±3.3 GFAP-positive cells (under 200×magnification). However, with intrathecal injections of 15 µg of morphine once daily for 7 days (M1-7 group), GFAP IR significantly increased to 152.8±12.7 (P<0.001). These findings implicate astrocytic activation in the response to morphine. By contrast, following the co-administration of 200 µg of lamotrigine for 7 days (L1-7 group), the number of GFAP-positive cells significantly decreased to 110.3±8.7 (P=0.015 vs M1-7 group), respectively. When 200 µg of lamotrigine was coinjected with morphine for days 1-3 followed by daily morphine alone on days 4-7 (L1-3 group), the number of GFAP-positive cells was 94.5±7.4. In the group co-administered with 200 µg of lamotrigine with morphine on days 5-7 (L5-7 group), the corresponding cell number was 115.3±8.3. In these three groups (L1-7, L1-3, and L5-7), the numbers of GFAP-positive cells were significantly lower (P=0.015, P<0.001, P=0.044) than those of the M1-7 group, however, they were significantly higher (P<0.001) than the numbers of GFAP-positive cells of the control group.
This study shows that lamotrigine inhibits the development of antinociceptive tolerance to morphine (L1-7 group); however, morphine tolerance becomes apparent within 48 hr of lamotrigine discontinuation (L1-3 group), indicating the need for the continued presence of the analgesic to maintain opioid potency. Lamotrigine was shown to partially restore the potency of morphine in tolerant rats (L5-7 group). In parallel, OX-42 and GFAP IR increased with the development of morphine tolerance but was clearly inhibited in rats co-administered with lamotrigine. For the 2 antigens, significantly fewer positively stained cells were counted in rats receiving lamotrigine during the first 3 (L1-3 group) or last 3 days (L5-7 group) than in those given morphine alone (M1-7 group). These findings are consistent with the ability of lamotrigine to block as well as reverse the development and expression of morphine tolerance, as evidenced by the suppression of spinal glial cell activation.
Our observations correspond well with those of previous studies reporting the attenuation by anticonvulsant drugs of opioid tolerance (12). To the best of our knowledge, this study is the first to examine the relationship between intrathecal lamotrigine and glial cell activation in morphine-tolerant rats.
Morphine tolerance is mediated by glutamate action at spinal NMDA (3) and AMPA/kainite (4) receptors. Interactions between NMDA and opioid receptors could occur in both directions (24). Glutamate is the major excitatory neurotransmitter in the neuronal circuit and it plays a major role in pain modulation as well as opioid tolerance, dependence, and withdrawal (25). Down-regulation of glutamate transporter protein expression has been suggested to contribute to the development of morphine tolerance (26). Therefore, any condition that leads to NMDA receptor activation within the CNS could also modulate opioid receptors, thereby reducing the efficacy of opioid analgesia. In addition, repeated treatment with opioids could produce a condition imitating continuous nociceptive input through interactions between opioid and NMDA receptors (24, 27). The activation of AMPA receptors during chronic morphine treatment may contribute to analgesic tolerance. Since NMDA receptor antagonists are also effective in attenuating the development of morphine tolerance (28), the activation of more than one excitatory amino acid receptor system might mediate the development of morphine tolerance (5). It may also be the case that AMPA and NMDA receptors make similar but not identical contributions to tolerance development (5).
The addition of a second drug to morphine infusion is an effective strategy for attenuating morphine tolerance and maintaining antinociceptive efficacy, as demonstrated in chronic morphine-infused rats (29). Specifically, the intrathecal co-administration of amitriptyline (25), MK-801 (30), or ultra-low dose naloxone (8) with morphine effectively preserved the antiwnociceptive effect of the opioid. All these co-administered drugs have a modulating effect on spinal glutamatergic transmission, inhibiting both the increase in morphine-evoked excitatory amino acid responses and the up-regulation of glutamate transporter protein expression in different rat models. Consequently, they have been added to morphine infusions in order to preserve the antinociceptive effect, at least in part, by modulating spinal glutamatergic transmission.
Chronic morphine administration causes glial cell activation and produces pro-inflammatory cytokine expression in rat spinal cords (9, 31). In response to diverse noxious stimuli, activated astrocytes and microglia in the spinal cord release pro-inflammatory cytokines, including TNFα, IL-1β, and IL-6, which promote the release of other pain-related transmitters or substances (e.g., nitric oxide, prostaglandins, and excitatory amino acids) from primary afferent nerve terminals (32). Glial metabolic inhibitors, cytokine antagonists, the anti-inflammatory cytokine IL-10, and the IL-1 receptor antagonist IL-1ra have all been shown to attenuate the development of morphine tolerance and to prevent hyperalgesia and allodynia (9, 33). Spinal glial cell activation may be triggered through AMPA/kainate receptors (34) and substance P (35). Some pain-related transmitters or substances (e.g., nitric oxide, prostaglandins, ATP, neuropeptides and excitatory amino acids) may precipitate spinal glial cell activations (36-39).
Lamotrigine likely exerts its actions by blocking voltage-dependent sodium channels, thus stabilizing the presynaptic neuronal membrane and preventing the release of excitatory neurotransmitters, predominantly glutamate (40). By this mechanism, intrathecally administered lamotrigine could prevent the activation of the glutamate receptors involved in pain transmission and central sensitization, such as AMPA, NMDA, and metabotropic receptors (14). Thus, taken together, lamotrigine may suppress the spinal glial cell activation through inhibition of AMPA/kainate receptors, and pain-related transmitters or substances. It is also reported that lamotrigine induced functional modifications and affected some biochemical events in glial cells in vitro study (19). Therefore, it could be suggested that lamotrigine may produce some inhibitory effect to the glial cells by itself.
In conclusion, the results of this study suggest that intrathecally administered lamotrigine attenuates antinociceptive morphine tolerance through a mechanism that is, at least in part, associated with the suppression of spinal glial cell activation. Intrathecal lamotrigine or other inhibitors of glial cell activation could therefore be used to prevent the development or persistence of morphine tolerance. The combined administration of the two drugs could effectively alleviate morphine tolerance without severe side effects. Further studies are needed to identify the sites and mechanisms of lamotrigine actions. Also, Clinical investigations are needed to identify specific settings and patient populations in which the morphine tolerance could be developed or morphine tolerance is already appeared (for example, chronic cancer pain patients with metastases).
Figures and Tables
Fig. 1
The acute responses of thermal nociception (mean ± SEM) to intrathecal saline, morphine, lamotrigine, and morphine plus lamotrigine. All doses of morphine and lamotrigine are 5 µg and 200 µg, respectively. *P < 0.05 vs control group; †P < 0.05 vs morphine group; ‡P < 0.05 vs lamotrigine group. MPE, maximum possible effect.
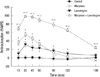
Fig. 2
The chronic responses of thermal nociception (mean ± SEM) to intrathecal saline, morphine, lamotrigine, and morphine plus lamotrigine (lamotrigine given on days 1-7, days 5-7, or days 1-3). All doses of morphine and lamotrigine are 15 µg and 200 µg, respectively. *P < 0.05 vs control group; †P < 0.05 vs M1-7 group. Control: intrathecal injection of saline (10 µL) once daily for 7 days. M1-7: intrathecal injection of morphine (15 µg) once daily for 7 days. L1-7: intrathecal coinjection of morphine (15 µg) and lamotrigine (200 µg) once daily for 7 days. L5-7: intrathecal injection of morphine (15 µg) once daily for 4 days, followed by intrathecal coinjection of morphine (15 µg) and lamotrigine (200 µg) on days 5-7. L1-3: intrathecal coinjection of morphine (15 µg) and lamotrigine (200 µg) once daily for 3 days, followed by daily morphine alone on days 4-7.
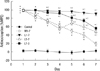
Fig. 3
Spinal immunoreactivity to OX-42 under 200 × magnification after a 7 day treatment with intrathecal saline, morphine, and lamotrigine, and the combinations thereof. The number of OX-42-positive cells increased with the development of morphine tolerance but was clearly inhibited by the co-administration of 200 µg of lamotrigine. There were significantly fewer OX-42-positive cells in rats co-administered with 200 µg of lamotrigine during the first or last 3 days than in the morphine alone group. *P < 0.05 vs control group; †P < 0.05 vs M1-7 group. Control: intrathecal injection of saline (10 µL) once daily for 7 days. M1-7: intrathecal injection of morphine (15 µg) once daily for 7 days. L1-7: intrathecal coinjection of morphine (15 µg) and lamotrigine (200 µg) once daily for 7 days. L1-3: intrathecal coinjection of morphine (15 µg) and lamotrigine (200 µg) once daily for 3 days, followed by daily morphine alone on days 4-7. L5-7: intrathecal injection of morphine (15 µg) once daily for 4 days, followed by intrathecal coinjection of morphine (15 µg) and lamotrigine (200 µg) on days 5-7.
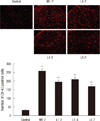
Fig. 4
Spinal immunoreactivity to glial fibrillary acidic protein (GFAP), as observed under 200 × magnification, after a 7 day treatment with intrathecal saline, morphine, and lamotrigine, and combinations thereof. The development of morphine tolerance was accompanied by an increase in the number of GFAP-positive cells, which was clearly inhibited by the coadministration of 200 µg of lamotrigine. There were significantly fewer GFAP-positive cells in the groups of rats co-administered 200 µg of lamotrigine during the first or last 3 days than in the morphine alone group. *P < 0.05 vs control group; †P < 0.05 vs M1-7 group. Control: intrathecal injection of saline (10 µL) once daily for 7 days. M1-7: intrathecal injection of morphine (15 µg) once daily for 7 days. L1-7: intrathecal coinjection of morphine (15 µg) and lamotrigine (200 µg) once daily for 7 days. L1-3: intrathecal coinjection of morphine (15 µg) and lamotrigine (200 µg) once daily for 3 days, followed by daily morphine alone on days 4-7. L5-7: intrathecal injection of morphine (15 µg) once daily for 4 days, followed by intrathecal coinjection of morphine (15 µg) and lamotrigine (200 µg) on days 5-7.
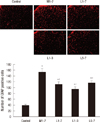
Table 1
Number of rats used in the experiments
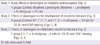
Control: intrathecal injection of saline (10 µL) once daily for 7 days. M1-7: intrathecal injection of morphine (15 µg) once daily for 7 days. L1-7: intrathecal coinjection of morphine (15 µg) and lamotrigine (200 µg) once daily for 7 days. L5-7: intrathecal injection of morphine (15 µg) once daily for 4 days, followed by intrathecal coinjection of morphine (15 µg) and lamotrigine (200 µg) on days 5-7. L1-3: intrathecal coinjection of morphine (15 µg) and lamotrigine (200 µg) once daily for 3 days, followed by daily morphine alone on days 4-7.
References
1. Starowicz K, Sieja A, Bilecki W, Obara I, Przewlocka B. The effect of morphine on MC4 and CRF receptor mRNAs in the rat amygdala and attenuation of tolerance after their blockade. Brain Res. 2003. 990:113–119.
2. Cortinez LI, Brandes V, Muñoz HR, Munoz HR, Guerrero ME, Mur M. No clinical evidence of acute opioid tolerance after remifentanil-based anaesthesia. Br J Anaesth. 2001. 87:866–869.
3. Mayer DJ, Mao J, Holt J, Price DD. Cellular mechanisms of neuropathic pain, morphine tolerance, and their interactions. Proc Natl Acad Sci U S A. 1999. 96:7731–7736.
4. Kest B, McLemore G, Kao B, Inturrisi CE. The competitive alpha-amino-3-hydroxy-5-methylisoxazole-4-propionate receptor antagonist LY293558 attenuates and reverses analgesic tolerance to morphine but not to delta or kappa opioids. J Pharmacol Exp Ther. 1997. 283:1249–1255.
5. Vanderah TW, Ossipov MH, Lai J, Malan TP Jr, Porreca F. Mechanisms of opioid-induced pain and antinociceptive tolerance: descending facilitation and spinal dynorphin. Pain. 2001. 92:5–9.
6. Powell KJ, Ma W, Sutak M, Doods H, Quirion R, Jhamandas K. Blockade and reversal of spinal morphine tolerance by peptide and non-peptide calcitonin gene-related peptide receptor antagonists. Br J Pharmacol. 2000. 131:875–884.
7. Powell KJ, Hosokawa A, Bell A, Sutak M, Milne B, Quirion R, Jhamandas K. Comparative effects of cyclo-oxygenase and nitric oxide synthase inhibition on the development and reversal of spinal opioid tolerance. Br J Pharmacol. 1999. 127:631–644.
8. Lin SL, Tsai RY, Shen CH, Lin FH, Wang JJ, Hsin ST, Wong CS. Co-administration of ultra-low dose naloxone attenuates morphine tolerance in rats via attenuation of NMDA receptor neurotransmission and suppression of neuroinflammation in the spinal cords. Pharmacol Biochem Behav. 2010. 96:236–245.
9. Raghavendra V, Rutkowski MD, DeLeo JA. The role of spinal neuroimmune activation in morphine tolerance/hyperalgesia in neuropathic and sham-operated rats. J Neurosci. 2002. 22:9980–9989.
10. Horvath RJ, DeLeo JA. Morphine enhances microglial migration through modulation of P2X4 receptor signaling. J Neurosci. 2009. 29:998–1005.
11. Marchand F, Perretti M, McMahon SB. Role of the immune system in chronic pain. Nat Rev Neurosci. 2005. 6:521–532.
12. Chavooshi B, Saberi M, Pournaghash Tehrani S, Bakhtiarian A, Ahmadiani A, Haghparast A. Vigabatrin attenuates the development and expression of tolerance to morphine-induced antinociception in mice. Pharmacol Biochem Behav. 2009. 93:155–159.
13. Lees G, Leach MJ. Studies on the mechanism of action of the novel anticonvulsant lamotrigine (Lamictal) using primary neurological cultures from rat cortex. Brain Res. 1993. 612:190–199.
14. Klamt JG. Effects of intrathecally administered lamotrigine, a glutamate release inhibitor, on short- and long-term models of hyperalgesia in rats. Anesthesiology. 1998. 88:487–494.
15. Habibi-Asl B, Hassanzadeh K, Vafai H, Mohammadi S. Development of morphine induced tolerance and withdrawal symptoms is attenuated by lamotrigine and magnesium sulfate in mice. Pak J Biol Sci. 2009. 12:798–803.
16. Kugaya A, Sanacora G. Beyond monoamines: glutamatergic function in mood disorders. CNS Spectr. 2005. 10:808–819.
17. Perisic T, Zimmermann N, Kirmeier T, Asmus M, Tuorto F, Uhr M, Holsboer F, Rein T, Zschocke J. Valproate and amitriptyline exert common and divergent influences on global and gene promoter-specific chromatin modifications in rat primary astrocytes. Neuropsychopharmacology. 2010. 35:792–805.
18. Kim DS, Kim JE, Kwak SE, Choi HC, Song HK, Kimg YI, Choi SY, Kang TC. Up-regulated astroglial TWIK-related acid-sensitive K+ channel-1 (TASK-1) in the hippocampus of seizure-sensitive gerbils: a target of anti-epileptic drugs. Brain Res. 2007. 1185:346–358.
19. Pavone A, Cardile V. An in vitro study of new antiepileptic drugs and astrocytes. Epilepsia. 2003. 44:34–39.
20. Yaksh TL, Rudy TA. Chronic catheterization of the spinal subarachnoid space. Physiol Behav. 1976. 17:1031–1036.
21. Jun IG, Park JY, Choi YS, Kim TH. Intrathecal lamotrigine blocks and reverses antinociceptive morphine tolerance in rats. Korean J Anesthesiol. 2009. 56:687–692.
22. Hargreaves K, Dubner R, Brown F, Flores C, Joris J. A new and sensitive method for measuring thermal nociception in cutaneous hyperalgesia. Pain. 1988. 32:77–88.
23. Cui Y, Liao XX, Liu W, Guo RX, Wu ZZ, Zhao CM, Chen PX, Feng JQ. A novel role of minocycline: attenuating morphine antinociceptive tolerance by inhibition of p38 MAPK in the activated spinal microglia. Brain Behav Immun. 2008. 22:114–123.
24. Mao J, Price DD, Mayer DJ. Mechanisms of hyperalgesia and morphine tolerance: a current view of their possible interactions. Pain. 1995. 62:259–274.
25. Tai YH, Wang YH, Wang JJ, Tao PL, Tung CS, Wong CS. Amitriptyline suppresses neuroinflammation and up-regulates glutamate transporters in morphine-tolerant rats. Pain. 2006. 124:77–86.
26. Sung CS, Wen ZH, Chang WK, Chan KH, Ho ST, Tsai SK, Chang YC, Wong CS. Inhibition of p38 mitogen-activated protein kinase attenuates interleukin-1beta-induced thermal hyperalgesia and inducible nitric oxide synthase expression in the spinal cord. J Neurochem. 2005. 94:742–752.
27. Mao J, Price DD, Mayer DJ. Experimental mononeuropathy reduces the antinociceptive effects of morphine: implications for common intracellular mechanisms involved in morphine tolerance and neuropathic pain. Pain. 1995. 61:353–364.
28. Elliott K, Kest B, Man A, Kao B, Inturrisi CE. N-methyl-D-aspartate (NMDA) receptors, mu and kappa opioid tolerance, and perspectives on new analgesic drug development. Neuropsychopharmacology. 1995. 13:347–356.
29. Wong CS, Hsu MM, Chou R, Chou YY, Tung CS. Intrathecal cyclooxygenase inhibitor administration attenuates morphine antinociceptive tolerance in rats. Br J Anaesth. 2000. 85:747–751.
30. Wen ZH, Chang YC, Cherng CH, Wang JJ, Tao PL, Wong CS. Increasing of intrathecal CSF excitatory amino acids concentration following morphine challenge in morphine-tolerant rats. Brain Res. 2004. 995:253–259.
31. Johnston IN, Milligan ED, Wieseler-Frank J, Frank MG, Zapata V, Campisi J, Langer S, Martin D, Green P, Fleshner M, et al. A role for proinflammatory cytokines and fractalkine in analgesia, tolerance, and subsequent pain facilitation induced by chronic intrathecal morphine. J Neurosci. 2004. 24:7353–7365.
32. Watkins LR, Milligan ED, Maier SF. Glial proinflammatory cytokines mediate exaggerated pain states: implications for clinical pain. Adv Exp Med Biol. 2003. 521:1–21.
33. Song P, Zhao ZQ. The involvement of glial cells in the development of morphine tolerance. Neurosci Res. 2001. 39:281–286.
34. Noda M, Nakanishi H, Nabekura J, Akaike N. AMPA-kainate subtypes of glutamate receptor in rat cerebral microglia. J Neurosci. 2000. 20:251–258.
35. Rasley A, Bost KL, Olson JK, Miller SD, Marriott I. Expression of functional NK-1 receptors in murine microglia. Glia. 2002. 37:258–267.
36. De Leo JA, Tawfik VL, LaCroix-Fralish ML. The tetrapartite synapse: path to CNS sensitization and chronic pain. Pain. 2006. 122:17–21.
37. Pogatzki EM, Gebhart GF, Brennan TJ. Characterization of Adelta- and C-fibers innervating the plantar rat hindpaw one day after an incision. J Neurophysiol. 2002. 87:721–731.
38. Scholz J, Woolf CJ. The neuropathic pain triad: neurons, immune cells and glia. Nat Neurosci. 2007. 10:1361–1368.
39. Watkins LR, Milligan ED, Maier SF. Glial activation: a driving force for pathological pain. Trends Neurosci. 2001. 24:450–455.
40. Leach MJ, Marden CM, Miller AA. Pharmacological studies of lamotrigine, a novel potential antiepileptic drug: II. Neurochemical studies on the mechanism of action. Epilepsia. 1986. 27:490–497.