Abstract
Although oxidized low-density lipoprotein (LDL) and lysophosphatidylcholine (LPC) have been proposed as important mediators of the atherosclerosis, the long-term contribution to the risk of cardiovascular disease (CVD) in hemodialysis patients has not been evaluated. This study investigated the relation between oxidized LDL and LPC levels with long term risk of CVD. Plasma oxidized LDL and LPC levels were determined in 69 Korean hemodialysis patients as a prospective observational study for 5 yr. During the observation period, 18 cardiovascular events (26.1%) occurred including 6 deaths among the hemodialysis patients. The low LPC level group (≤ 254 µM/L, median value) had much more increased risk of CVD compared to the high LPC level group (> 254 µM/L) (P = 0.01). However, serum levels of oxidized LDL were not significantly different between groups with and without CVD. In adjusted Cox analysis, previous CVD, (hazard ratio [HR], 5.68; 95% confidence interval [CI], 1.94-16.63, P = 0.002) and low LPC level (HR, 3.45; 95% CI, 1.04-11.42, P = 0.04) were significant independent risk factors for development of CVD. It is suggested that low LPC, but not oxidized LDL, is associated with increased risk of CVD among a group of Korean hemodialysis patients.
Patients with end-stage renal disease have a high incidence of cardiovascular disease (CVD). Traditional and non-traditional risk factors contribute to the atherosclerotic process in this population. Dyslipidemia could be one of the key factors for atherosclerosis and oxidized low-density lipoprotein (LDL) would present a critical step in the initiation and progression of atherosclerosis (1, 2).
Lysophosphatidylcholine (LPC) consists of a glycerin frame, one fatty acid and a phosphochloine. LPC is a major component of oxidized LDL and is generated by phospholipase A2-dependent hydrolysis of phosphatidylcholine (2). LPC modulates many biologic effects of oxidized LDL including induction of monocyte chemotaxis, expression of adhesion molecules and growth factors in endothelial cells, stimulation of smooth muscle cell proliferation, gene transcription and secretion of proinflammatory cytokines (2). In addition, oxidized phospholipids and LPC accumulate in atherosclerotic lesions in experimental animals, supporting their involvement in atherogenesis (3). Based on these observations, LPC has been considered to be an important mediator of the atherogenic actions of oxidized LDL.
Among dialysis patients, a number of lipid abnormalities have been identified, including low levels of high-density lipoprotein (HDL), elevated triglycerides levels, and normal or near-normal levels of total cholesterol and LDL (4, 5). Although several studies have shown increased concentrations of oxidized LDL (6), as well as LPC (7, 8) in patients with renal disease, the relative long-term contribution of oxidized LDL and LPC to CVD has not been evaluated in hemodialysis patients. The objective of present study was to address this issue.
Oxidized LDL and LPC levels were measured in two groups (hemodialysis and healthy controls). The subjects in the study were 69 patients on maintenance hemodialysis (32 men and 37 women, mean age 56.2 ± 12.1 yr) and 33 controls (16 men and 17 women, mean age 55.6 ± 12.2 yr). The two groups were comparable in age and gender. Patients were enrolled from Hallym University Kanganam Sacred Heart Hospital.
A prospective follow-up study of 69 chronic hemodialysis patients was conducted for 60 months (August 2004-July 2009) and cardiovascular morbidity and mortality were determined. Patients received three 4 hr sessions of hemodialysis weekly using bicarbonate-buffered dialysate. The dialysis membrane used in all patients was composed of polysulfone (Fresenius® F6HPS, Bad Homberg, Germany) with a surface area of 1.3 m2. Exclusion criteria were: less than 18 yr old, dialysis duration of less than 3 months, history of acute infection or systemic inflammatory disease within 3 months, malignancy or chronic liver disease. The mean hemodialysis duration was 40.6 months (range 3 to 172 months). Fifty-four patients (78.3%) undergoing dialysis had arteriovenous fistula. The underlying renal diseases consisted of diabetes mellitus (53.6%), hypertension (23.2%), chronic glomerulonephritis (14.5%), urologic disease (2.9%) and unknown (5.8%).
Longitudinal data were collected for each patient at entry into the study and updated whenever a cardiovascular event occurred. The endpoints of the study were new fatal and non-fatal cardiovascular events requiring admission. Coronary artery disease, cerebrovascular disease and peripheral artery disease were defined as CVD.
Venous blood was collected after an overnight fasting before the start of the mid-week hemodialysis session. Samples for hemoglobin, blood urea nitrogen (BUN), creatinine, albumin, calcium, phosphate, C-reactive protein (CRP), parathyroid hormone and lipid profile were transferred to laboratory immediately. For the other parameters, the samples were centrifuged and frozen at -70℃ until examination. Total cholesterol, triglyceride and HDL cholesterol levels were measured by enzymatic methods. LDL cholesterol was calculated using Friedewald's formula. Oxidation of LDL was assessed by using an enzyme linked immunosorbent assay kit (Mercodia, Uppsala, Sweden). LPC concentrations were determined as described previously (9), based on the standard curve for 18:0 LPC. Patient serum samples were mixed with LPC assay reagents, and their changes in absorbance were assayed.
All values were expressed as mean ± standard deviations. Mann-Whitney U-test for categorical variables, chi-square test for continuous variables, and Spearman's coefficient for correlation analysis were performed for the statistical analysis. In a prospective follow-up study, the primary analysis used was a Cox regression analysis of CVD from the time of entry. We investigated the relationship between predictor variables, including oxidized LDL and LPC, and CVD. Survival analysis was performed using the Kaplan-Meier estimation. The log-rank test was used for univariate comparisons. Patients were censored when they were transferred to another dialysis center, switched to peritoneal dialysis, received a renal transplantation, or died on the final observation date (July 31, 2009). All reported P values are two-sided, and P < 0.05 was considered statistically significant.
The baseline clinical characteristics of 69 hemodialysis patients and 33 controls are shown in Table 1. Total cholesterol and LDL-cholesterol levels were significantly lower in hemodialysis patients than in controls (P < 0.001). Lipid profiles such as HDL, LDL, and LDL/HDL were not significantly different between the two groups. There were differences in serum creatinine, glucose, albumin, and phosphorus levels between two groups. Oxidized LDL level was lower in hemodialysis patients than in controls (P = 0.003) (Table 2). Hemodialysis patients also showed decreased LPC compared to controls (P < 0.001). The oxidized LDL/LDL, LPC/oxidized LDL and LPC/(LDL + HDL) were comparable in two groups.
In patients with hemodialysis and controls together, oxidized LDL was found to have significant positive correlations with total cholesterol (r = 0.75; P < 0.001), triglyceride (r = 0.38; P = 0.001), LDL-cholesterol (r = 0.66; P < 0.001), albumin (r = 0.26; P = 0.02) and LPC (r = 0.28; P = 0.004). LPC also had significant positive correlations with total cholesterol (r = 0.39; P < 0.001), triglyceride (r = 0.31; P = 0.006), LDL-cholesterol (r = 0.28; P = 0.01) and albumin (r = 0.25; P = 0.03) (Fig. 1).
In hemodialysis group, oxidized LDL concentration was not different between the patients with or without diabetes. But, LPC in dialysis patients with diabetes (252.6 ± 63.5 µM/L) was significantly lower than patients without diabetes (284.8 ± 65.2 µM/L, P = 0.04). There were 18 patients (26%) who had previous CVD. Serum lipid levels, oxidized LDL and LPC were not statistically different between the patients with or without previous CVD.
A total of 69 patients was evaluated for cardiovascular disease. Patients were observed over an average follow-up of 43 months (2-60 months). During the study period, 20 patients (29%) died, 12 patients were transferred to other centers, and five patients were transplanted. The primary objective of this study was to determine possible association between oxidized LDL or LPC and new fatal or non-fatal cardiovascular events. There were 18 cardiovascular events (26%) (12 coronary artery disease, 4 cerebrovascular disease and 2 peripheral vascular disease) including 6 deaths among the hemodialysis patients.
The subjects were divided two groups according to serum LPC levels at baseline (median value of LPC: 254 µM/L) (Table 3). The low LPC level group showed higher pulse pressure and decreased phosphorus level compared to the high LPC level group. Age, gender, blood pressure, total cholesterol, triglyceride, HDL, LDL, oxidized LDL, albumin and CRP levels were not significant different between two groups. The low LPC level group had much more increased risk of CVD compared to the high LPC level group (P = 0.01, Table 4). There was also significant difference in the probability of the cardiovascular events-free rate between two groups (log-rank test, P = 0.02, Fig. 2). The subjects were also divided two groups according to serum oxidized LDL levels at baseline (median value: 31.1 U/L). But, oxidized LDL levels were not significant different between groups with CVD and without CVD.
The results of Cox proportional hazard analysis for CVD are summarized in Table 5. In univariate analysis, previous history of cardiovascular events, low LPC level (≤254 µM/L), diabetes mellitus and duration of dialysis were found to be significantly risk factors for CVD. In adjusted Cox model, previous CVD (hazard ratio [HR], 5.68; 95% confidence interval [CI], 1.94-16.63, P = 0.002) and low LPC level (HR, 3.45; 95% CI, 1.04-11.42, P = 0.042) had significant independent risks for development of CVD. However, neither diabetes mellitus nor duration of dialysis was significantly associated with the risk of CVD in the study population.
The present study examined whether a serum level of LPC or oxidized LDL predicts CVD in a cohort of 69 hemodialysis patients who were followed up for 5 yr. We demonstrated that lower LPC and previous history of CVD are the major risk factors for CVD. To our knowledge, this is the first study to demonstrate that a decreased LPC concentration is an independent predictor of a higher risk of CVD in hemodialysis patients.
Our results showed that low LPC was associated with significantly higher risk of CVD, but the underlying mechanism for this phenomenon is presently unclear. LPC appears to be a proinflammatory mediator and may affect endothelial function resulting in vascular inflammation. In vitro studies have demonstrated that LPC has effects on vascular wall cells that could contribute to all stages of atherosclerosis (1). Messner et al. (10) recently provided evidence that LPC was elevated in human atherosclerotic tissue. Conversely, LPC is reported as being protective against vascular injury in animal model. A study shows that LPC pretreatment prevents lung injury by N-formyl-methionyl-leucyl-phenylalanine (fMLP)-induced neutrophils activation in an isolated perfused lung model (11). In another study, subcutaneous administration of LPC protected mice against sepsis after cecal ligation and puncture or intraperitoneal injection of Escherichia coli (12). These data suggest that LPC could prevent sepsis and microbial infections. Along the same lines, Drobnik et al. (13) demonstrated the plasma LPC levels were inversely correlated with mortality in sepsis patients. Based on these data, LPC may also exert pro- and anti-atherogenic roles, depending on the arterial cell type and oxidation/inflammation status (14).
Several studies have shown increased concentrations of LPC in hemodialysis patients (7) and with nephrotic syndrome (15). Only one study reported lower LPC values in patients with chronic renal failure (16). The present study demonstrated that LPC levels were obviously decreased in hemodialysis patients. In hemodialysis group, LPC concentration in patients with diabetes was significantly lower than patients without diabetes. It is not readily answered why hemodialysis patients had lower LPC concentrations. Another explanation has to do with the changes of the plasma enzyme lecithin-cholesterol acyltransferase in patients with end-stage renal disease (17). In the general population, higher serum cholesterol levels typically increase CVD risk. By comparison, in maintenance dialysis patients, the relationship of dyslipidemia with CVD and mortality is conflicting and often paradoxical. This may reflect the profound adverse effect of malnutrition and chronic inflammation upon mortality (18, 19). This observation suggests that LPC level has some linkage to the nutritional status.
The most common methods published for LPC measurement involve thin-layer chromatography followed by gas chromatography and a high-performance liquid chromatography. However, the application of these methods of LPC assay is now limited because they are complicated, time-consuming, required skilled techniques that are partly insensitive (9). Recently, the analytic possibilities for LPC measurement considerably improved. We use a new enzymatic method of LPC assay using lysophospholipase, glycerophosphorylcholine phosphodiesterase and choline oxidase. It is simple and measures total LPC in a sample. This method provides a good correlation when compared to a gas chromatography of fatty acid analysis for LPC in human (9).
Although a several studies have shown that circulating oxidized LDL levels were associated with stable coronary artery disease and acute coronary syndromes (20), there are conflicting findings in hemodialysis patients. A study showed that oxidized LDL titer was one of the principal risk factors for cardiovascular mortality in the population of hemodialysis patients (21). However, oxidized LDL is not associated with coronary artery calcification in maintenance hemodialysis patients (22). And, oxidized LDL is of limited clinical value in identifying young hemodialysis patients at risk for vascular complications (23). In our study, oxidized LDL is not different among groups with CVD and without CVD.
The primary strength of our study is based on the prospective design and longitudinal nature of the protocol. The limitation of the study is its relatively small sample size. Likewise the potential impacts of such risk factors as lipoprotein-associated phospholipase A2 and other oxidative stress markers were not tested. Another limitation of the present study is the possibility of violating the assumption of non-informative censoring due to inability to follow patients who transferred to other centers.
In conclusion, the study demonstrates that low level of LPC, but not oxidized LDL, is the principal risk factors for CVD in a population of Korean hemodialysis patients. Larger-scale longitudinal studies are needed to confirm our results and to explore this phenomenon in dialysis population.
Figures and Tables
Fig. 1
Correlation between total cholesterol, LDL-cholesterol, albumin and LPC. LDL, low-density lipoproteins; LPC, Lysophosphatidylcholine.
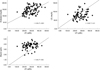
Fig. 2
Kaplan-Meier estimate of CVD-free survival in hemodialysis patients according to LPC levels. The test demonstrated a statistically significant value (log-rank test, P = 0.017).
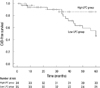
ACKNOWLEDGMENTS
The authors wish to acknowledge the contributions of the following individuals to this study: the nurses in dialysis centers in Kangnam Sacred Heart Hospital and nephrology staffs in Hallym University Medical Center. The authors thank professor Dong Hyun Kim of Department of Social and Preventive Medicine, College of Medicine, Hallym University for his work in statistical analysis. The authors have no conflicts of interest to disclose.
References
1. Matsumoto T, Kobayashi T, Kamata K. Role of lysophosphatidylcholine (LPC) in atherosclerosis. Curr Med Chem. 2007. 14:3209–3220.
2. Steinberg D, Parthasarathy S, Carew TE, Khoo JC, Witztum JL. Beyond cholesterol. Modifications of low-density lipoprotein that increase its atherogenicity. N Engl J Med. 1989. 320:915–924.
3. McIntyre TM, Zimmerman GA, Prescott SM. Biologically active oxidized phospholipids. J Biol Chem. 1999. 274:25189–25192.
4. Vaziri ND. Dyslipidemia of chronic renal failure: the nature, mechanisms and potential consequences. Am J Physiol Renal Physiol. 2006. 290:F262–F272.
5. Vaziri ND, Navab M, Fogelman AM. HDL metabolism and activity in chronic kidney disease. Nat Rev Nephrol. 2010. 6:287–296.
6. Maggi E, Bellazzi R, Falaschi F, Frattoni A, Perani G, Finardi G, Gazo A, Nai M, Romanini D, Bellomo G. Enhanced LDL oxidation in uremic patients: an additional mechanism for accelerated atherosclerosis? Kidney Int. 1994. 45:876–883.
7. Sasagawa T, Suzuki K, Shiota T, Kondo T, Okita M. The significance of plasma lysophospholipids in patients with renal failure on hemodialysis. J Nutr Sci Vitaminol. 1998. 44:809–818.
8. Vuong TD, Stroes ES, Willekes-Koolschijn N, Rabelink TJ, Koomans HA, Joles JA. Hypoalbuminemia increases lysophosphatidylcholine in low-density lipoprotein of normocholesterolemic subjects. Kidney Int. 1999. 55:1005–1010.
9. Kishimoto T, Soda Y, Matsuyama Y, Mizuno K. An enzymatic assay for lysophosphatidylcholine concentration in human serum and plasma. Clin Biochem. 2002. 35:411–416.
10. Messner MC, Albert CJ, McHowat J, Ford DA. Identification of lysophosphatidylcholine-chlorohydrin in human atherosclerotic lesions. Lipids. 2008. 43:243–249.
11. Lin P, Welch EJ, Gao XP, Malik AB, Ye RD. Lysophosphatidylcholine modulates neutrophil oxidant production through elevation of cyclic AMP. J Immunol. 2005. 174:2981–2989.
12. Yan JJ, Jung JS, Lee JE, Lee J, Huh SO, Kim HS, Jung KC, Cho JY, Nam JS, Suh HW, et al. Therapeutic effects of lysophosphatidylcholine in experimental sepsis. Nat Med. 2004. 10:161–167.
13. Drobnik W, Liebisch G, Audebert FX, Frohlich D, Gluck T, Vogel P, Rothe G, Schmitz G. Plasma ceramide and lysophosphatidylcholine inversely correlate with mortality in sepsis patients. J Lipid Res. 2003. 44:754–761.
14. Schmitz G, Ruebsaamen K. Metabolism and atherogenic disease association of lysophosphatidylcholine. Atherosclerosis. 2010. 208:10–18.
15. Thukkani AK, McHowat J, Hsu FF, Brennan ML, Hazen SL, Ford DA. Identification of alpha-chloro fatty aldehydes and unsaturated lysophosphatidylcholine molecular species in human atherosclerotic lesions. Circulation. 2003. 108:3128–3133.
16. Jia L, Chen J, Yin P, Lu X, Xu G. Serum metabonomics study of chronic renal failure by ultra performance liquid chromatography coupled with Q-TOF mass spectrometry. Metabolomics. 2008. 4:183–189.
17. Gillett MP, Obineche EN, Lakhani MS, Abdulle AM, Amirlak I, Al Rukhaimi M, Suleiman MN. Levels of cholesteryl esters and other lipids in the plasma of patients with end-stage renal failure. Ann Saudi Med. 2001. 21:283–286.
18. Iseki K, Yamazato M, Tozawa M, Takishita S. Hypocholesterolemia is a significant predictor of death in a cohort of chronic hemodialysis patients. Kidney Int. 2002. 61:1887–1893.
19. Kalantar-Zadeh K, Block G, Humphreys MH, Kopple JD. Reverse epidemiology of cardiovascular risk factors in maintenance dialysis patients. Kidney Int. 2003. 63:793–808.
20. Tsimikas S, Brilakis ES, Miller ER, McConnell JP, Lennon RJ, Kornman KS, Witztum JL, Berger PB. Oxidized phospholipids, Lp(a) lipoprotein, and coronary artery disease. N Engl J Med. 2005. 353:46–57.
21. Bayés B, Pastor MC, Bonal J, Foraster A, Romero R. Oxidative stress, inflammation and cardiovascular mortality in haemodialysis--role of seniority and intravenous ferrotherapy: analysis at 4 years of follow-up. Nephrol Dial Transplant. 2006. 21:984–990.
22. Kraśniak A, Drozdz M, Pasowicz M, Chmiel G, Michałek M, Szumilak D, Podolec P, Klimeczek P, Konieczyłska M, Wicher-Muniak E, et al. Factors involved in vascular calcification and atherosclerosis in maintenance haemodialysis patients. Nephrol Dial Transplant. 2007. 22:515–521.
23. Nissel R, Faraj S, Sommer K, Henning L, van der Giet M, Querfeld U. Oxidative stress markers in young hemodialysis patients: a pilot study. Clin Nephrol. 2008. 70:135–143.