Abstract
This study was done to characterize the natural course of C-peptide levels in patients with type 1 diabetes and identify distinguishing characters among patients with lower rates of C-peptide decline. A sample of 95 children with type 1 diabetes was analyzed to retrospectively track serum levels of C-peptide, HbA1c, weight, BMI, and diabetic complications for the 15 yr after diagnosis. The clinical characteristics were compared between the patients with low and high C-peptide levels, respectively. The average C-peptide level among all patients was significantly reduced five years after diagnosis (P < 0.001). The incidence of diabetic ketoacidosis was significantly lower among the patients with high levels of C-peptide (P = 0.038). The body weight and BMI standard deviation scores (SDS) 15 yr after diagnosis were significantly higher among the patients with low C-peptide levels (weight SDS, P = 0.012; BMI SDS, P = 0.044). In conclusion, C-peptide level was significantly decreased after 5 yr from diagnosis. Type 1 diabetes patients whose beta-cell functions were preserved might have low incidence of diabetic ketoacidosis. The declines of C-peptide level after diagnosis in type 1 diabetes may be associated with changes of body weight and BMI.
For many years, C-peptide was thought to be merely a derivate of insulin synthesis. C-peptide is co-secreted with insulin, but the half-life of C-peptide is about 10 times longer than that of insulin (1). Hence, serum C-peptide is used as a substitute marker for monitoring endogenous insulin production (2, 3).
In the past two decades, numerous studies demonstrated that C-peptide functions independently of insulin and controls the vascularity of many vital organs (4-7). In a long-term clinical observation, patients with type 1 diabetes who maintained minimal levels of C-peptide were less inclined to develop microvascular complications of the eyes, peripheral nerves, and kidneys compared with patients whose beta-cell functions were stopped completely (8). Studies of humans and animals show that infusions of synthetic C-peptide slow the progressive damage to the kidneys and peripheral nerves caused by type 1 diabetes (6, 7).
The preservation of C-peptide could be an important factor in the management of type 1 diabetes, but longitudinal C-peptide studies are currently sparse. During the first few years following diagnosis, the pattern of C-peptide decline is highly variable and is associated with several factors including the age at diagnosis, the degree of metabolic control, and immune status of the patient (8, 9). Much longer periods of observation, however, are necessary to fully understand the role of C-peptide in the management of type 1 diabetes.
We retrospectively investigated patients with type 1 diabetes who managed their disease for over 15 yr, and we examined the correlations between C-peptide levels and other clinical parameters. In addition, we compared the clinical parameters between patients with low C-peptide levels and those whose C-peptide levels were relatively preserved over the 15 yr following diagnosis.
We identified 95 patients (56 [59%] female and 39 [41%] male) who were diagnosed with type 1 diabetes as children and were followed for at least 15 yr after their diagnosis by researchers at the Childhood Diabetes Clinic of Severance Children's Hospital. Type 1 diabetes was defined by glucose level of more than 200 mg/dL, clinical characteristics, antibody positivity and decreased C-peptide level through the oral glucose tolerance test or the standard mixed meal tolerance test at initial visit. The ages of the patients at the time of diagnosis ranged from 1 to 16 yr. Most of the patients were treated with a conventional insulin regimen; the number of subjects receiving intensive insulin treatment 10 and 15 yr after their diagnosis was five and 35, respectively. We excluded patients with slowly progressive type 1 diabetes who were initially misdiagnosed as having type 2 diabetes, respectively.
We recorded the patients' sex, onset age, and family history. The family history included the history of all types of diabetes in any third-degree relatives. We collected data for serum fasting C-peptide levels at the time of diagnosis and 1, 3, 5, 10, and 15 yr after diagnosis. We used fasting C-peptide levels instead of postprandial or stimulated levels, because fasting levels were measured for the majority of the patients. The serum C-peptide levels were assessed by the radioimmunoassay technique using commercial kits (Daiichi, Tokyo, Japan). We also collected data for parameters such as HbA1c, triacylglycerol (TG), total cholesterol, high-density lipoprotein (HDL), and daily insulin dose. Our data included the incidences of diabetic complications such as diabetic retinopathy, peripheral neuropathy, albuminuria, and diabetic ketoacidosis (DKA). Diabetic retinopathy was diagnosed by an ophthalmologist, and peripheral neuropathy was confirmed by a neurologist with electromyography and nerve conduction study. Albuminuria was confirmed when the albumin excretion in 24-hr urine was more than 30 mg in repeated tests. DKA was diagnosed when glucose ≥ 250 mg/dL, pH ≤ 7.30, HCO3- ≤ 18 mEq/L, and the patient was positive for ketonuria. The weight and body mass index (BMI) standard deviation scores (SDS) of the patients were calculated by the Z-scores of the 2007 Korean National Growth Chart.
We analysed the declines in fasting C-peptide level and the clinical characteristics between the patients with low C-peptide levels (< 0.05 nM/L, Group A) and those with relatively preserved C-peptide levels (≥ 0.05 nM/L, Group B); we used the serum C-peptide levels 15 yr after diagnosis to assign the patients to the groups.
We analysed the data using the SAS program (version 9.1; SAS Institute, Cary, NC, USA). We used a linear mixed model to verify the demographic trends of the continuous variables over time respectively between the two groups of patients. We used chi-square tests or Fisher's exact tests to analyse the categorical data of the two groups. Statistical significance was determined as P < 0.05.
Among the 95 patients, family history of diabetes was present in 14 (15%). In the study, the average age of onset of type 1 diabetes among the patients was 7.7 yr. The average initial fasting C-peptide level was 0.187 nM/L. The average fasting C-peptide level was slightly higher than the initial level one year after diagnosis, and it slowly decreased thereafter. The average C-peptide level was significantly reduced from the initial level five years after diagnosis (P < 0.001, Table 1). When we compared the decline in the C-peptide levels between the patients in Group A (n = 76) and those in Group B (n = 19), we found that the difference in C-peptide levels between the two groups was significant 10 yr after diagnosis (P = 0.015). The C-peptide levels decreased rapidly five years after diagnosis in Group A, compared with relatively stable C-peptide levels in Group B during the same period (Fig. 1).
We found no significant differences between the groups in the age at diagnosis, sex, family history, daily required insulin doses per weight, and insulin treatment regimen. We found no significant differences between the groups in their average levels of HbA1c, TG, total cholesterol, and HDL over the 15 yr following diagnosis (Table 1).
DKA had a significantly lower incidence rate in Group B than in Group A (P = 0.038, Table 2). The incidence rates of diabetic retinopathy, peripheral neuropathy, and albuminuria were not significant different between the two groups. We found no significant correlation between any of the complications and onset age, sex, or family history.
We found that onset age, sex, family history, mean HbA1c, lipid profiles, and incidences of complications, except for DKA, were not correlated with the decline of C-peptide. Although the weight SDS (-0.38 ± 1.22) and BMI SDS (-0.83 ± 1.38) were initially low, both gradually increased over time. The overall changes in weight SDS and BMI SDS between groups A and B were not significantly different from one another in the linear mixed-model analysis. Pair-wise comparisons between the two groups at each time point, however, revealed significant differences 15 yr after diagnosis (weight SDS, P = 0.012; BMI SDS, P = 0.044; Fig. 2).
Some previous studies investigated the temporal variation in C-peptide levels to understand the natural course of C-peptide levels in type 1 diabetes. In the Diabetes Prevention Trial-Type 1 (DPT-1), sharp declines of stimulated C-peptide were observed during the six months prior to diagnosis (10, 11). This suggests that a large amount of beta-cell function is commonly lost in the peri-diagnostic period and could explain the low initial C-peptide levels found in the present study (12). The level of C-peptide secretion following diagnosis could be influenced by several factors, however, so the decline of C-peptide levels would not be expected to proceed uniformly (8, 9). In the present study, C-peptide secretion appeared to increase temporarily in the year following diagnosis, although the increase was not statistically significant. The trend of higher C-peptide levels in the first year might be a so-called "honeymoon" period of transient normalization of beta-cell function caused by diligent care following diagnosis (13). Serum C-peptide levels gradually decreased after the first year, and the decrease was significant 5 to 10 yr after the diagnosis.
We did not find any correlations between the variance of fasting C-peptide level and the variance of HbA1c level. The Diabetes Control and Complications Trial (DCCT), designed to compare intensive and conventional diabetes therapies, found that the intensive glucose-control group had lower HbA1c levels and a slower decline in stimulated C-peptide levels after diagnosis (14, 15). Most of the subjects in the present study were treated with a conventional insulin regimen; therefore we could not confirm the effect of intensive therapy on residual beta-cell function or C-peptide levels.
Many previous studies indicated that C-peptide slows the microvascular damage caused by the progression of type 1 diabetes (4-7). The capillary blood flow in the organs of type 1 diabetes patients could increase with C-peptide secretion or infusion (16). The incidence of type 1 diabetes complications such as peripheral neuropathy, diabetic retinopathy, and albuminuria, however, showed no significant difference between patients with low and relatively higher C-peptide levels in the present study. One previous study found that a fasting C-peptide level of at least 0.06 nM/L had a significant protective effect against the development of microvascular complications (17). Another study showed that prolonged insufficient secretion of C-peptide was associated with the development of microvascular complications (18). The correlation found in the present study between the elevated incidence of DKA and the decrement of C-peptide could be explained by the previously proven relationship between hyperglycemia and beta-cell dysfunction (19-21): the incidence of DKA could mean lower beta-cell mass with earlier depletion of C-peptide.
The weight SDS and the BMI SDS of the patients with low C-peptide levels increased gradually in our study. The weight SDS and BMI SDS of the patients with relatively preserved C-peptide levels, however, did not increase. Although the overall variances of weight change were not significantly different between the two groups in the linear mixed-model analysis, they were significantly different 15 yr after diagnosis. Weight gain is known as one of the major adverse effects of insulin therapy (22, 23). The weight gain caused by insulin treatment in type 1 diabetes could interact with decreased beta-cell function, and it is a known factor increasing insulin resistance in type 1 diabetes (24, 25). It is unclear whether the C-peptide decrement or the weight gain is more influential in causing insulin resistance. It is known, however, that insulin resistance is strongly associated with other metabolic complications such as hypertension, dyslipidemia, and atherosclerosis (26, 27). From this point of view, patients with type 1 diabetes who have preserved C-peptide secretion might gain beneficial effects on weight-gain-related complications after a long period of time.
This study has several limitations. First, we had to exclude the autoimmune factors of diabetes such as islet-cell antibodies, insulin autoantibodies, and autoantibodies to glutamic-acid decarboxylase from the analysis because of inconsistent measurements and a lack of data. Previous studies of autoimmune antibodies mainly focused on the onset of diabetes and not on the prognosis of type 1 diabetes (28, 29). Future studies of the effects of C-peptide on the progression of type 1 diabetes should include data for autoimmune antibodies. Second, our data include only fasting C-peptide concentrations without stimulated C-peptide levels. More accurate analysis of beta cell functions could be produced by comparing with the two types of data. Third, the number of patients in the relatively preserved C-peptide group was only 19, and the duration of the follow-up period was not enough. Long-term, multi-center studies with more patients would likely provide more insights.
In conclusion, we demonstrated that serum C-peptide levels significantly decreased 5 yr after diagnosis of type 1 diabetes. Patients whose beta-cell functions were preserved had fewer incidences of DKA, although we did not find significant effects on other complications of diabetes. Our results suggest that the declines in C-peptide levels and beta-cell function after diagnosis in type 1 diabetes are associated with changes of the body weight and BMI.
Figures and Tables
Fig. 1
Average fasting C-peptide levels of each of group A and B at the time of diagnosis and during serial follow-up over 15 yr. *P = 0.015 between groups A and B at the 15th year after diagnosis from a linear mixed model. Data are represented as mean ± SE. Black circle = group A, C-peptide level at 15th year < 0.05 nM/L; white circle = group B, C-peptide level at 15th year ≥ 0.05 nM/L.
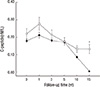
Fig. 2
Average body weight and BMI during follow-up period. (A) Body weight SDS with time progression in each of the two groups. *P = 0.012 between groups A and B at the 15th year after diagnosis from a linear mixed model. (B) BMI SDS with time progression in each of the two groups. †P = 0.044 between group A and B at the 15th year after diagnosis from a linear mixed model. Data are represented as mean ± SE. Black circle = group A, C-peptide level at 15th year < 0.05 nM/L; white circle = group B, C-peptide level at 15th year ≥ 0.05 nM/L.
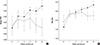
References
1. Matthews DR, Rudenski AS, Burnett MA, Darling P, Turner RC. The half-life of endogenous insulin and C-peptide in man assessed by somatostatin suppression. Clin Endocrinol (Oxf). 1985; 23:71–79.
2. Komulainen J, Knip M, Lounamaa R, Vähäsalo P, Karjalainen J, Sabbah E, Akerblom HK. Poor beta-cell function after the clinical manifestation of type 1 diabetes in children initially positive for islet cell specific auto-antibodies: the Childhood Diabetes in Finland Study Group: the Childhood Diabetes in Finland Study Group. Diabet Med. 1997; 14:532–537.
3. Kjems LL, Christiansen E, Vølund A, Bergman RN, Madsbad S. Validation of methods for measurement of insulin secretion in humans in vivo. Diabetes. 2000; 49:580–588.
4. Johansson BL, Borg K, Fernqvist-Forbes E, Kernell A, Odergren T, Wahren J. Beneficial effects of C-peptide on incipient nephropathy and neuropathy in patients with Type 1 diabetes mellitus. Diabet Med. 2000; 17:181–189.
5. Johansson BL, Sundell J, Ekberg K, Jonsson C, Seppänen M, Raitakari O, Luotolahti M, Nuutila P, Wahren J, Knuuti J. C-peptide improves adenosine-induced myocardial vasodilation in type 1 diabetes patients. Am J Physiol Endocrinol Metab. 2004; 286:E14–E19.
6. Samnegård B, Jacobson SH, Jaremko G, Johansson BL, Ekberg K, Isaksson B, Eriksson L, Wahren J, Sjöquist M. C-peptide prevents glomerular hypertrophy and mesangial matrix expansion in diabetic rats. Nephrol Dial Transplant. 2005; 20:532–538.
7. Ekberg K, Brismar T, Johansson BL, Lindström P, Juntti-Berggren L, Norrby A, Berne C, Arnqvist HJ, Bolinder J, Wahren J. C-peptide replacement therapy and sensory nerve function in type 1 diabetic neuropathy. Diabetes Care. 2007; 30:71–76.
8. Sjöberg S, Gunnarsson R, Gjötterberg M, Lefvert AK, Persson A, Ostman J. Residual insulin production, glycaemic control and prevalence of microvascular lesions and polyneuropathy in long-term type 1 (insulin-dependent) diabetes mellitus. Diabetologia. 1987; 30:208–213.
9. Törn C, Landin-Olsson M, Lernmark A, Palmer JP, Arnqvist HJ, Blohmé G, Lithner F, Littorin B, Nyström L, Scherstén B, et al. Prognostic factors for the course of beta cell function in autoimmune diabetes. J Clin Endocrinol Metab. 2000; 85:4619–4623.
10. Sosenko JM, Palmer JP, Greenbaum CJ, Mahon J, Cowie C, Krischer JP, Chase HP, White NH, Buckingham B, Herold KC, et al. Patterns of metabolic progression to type 1 diabetes in the Diabetes Prevention Trial-Type 1. Diabetes Care. 2006; 29:643–649.
11. Sosenko JM, Palmer JP, Rafkin-Mervis L, Krischer JP, Cuthbertson D, Matheson D, Skyler JS. Glucose and C-peptide changes in the perionset period of type 1 diabetes in the Diabetes Prevention Trial-Type 1. Diabetes Care. 2008; 31:2188–2192.
12. Palmer JP, Fleming GA, Greenbaum CJ, Herold KC, Jansa LD, Kolb H, Lachin JM, Polonsky KS, Pozzilli P, Skyler JS, et al. C-peptide is the appropriate outcome measure for type 1 diabetes clinical trials to preserve beta-cell function: report of an ADA workshop, 21-22 October 2001. Diabetes. 2004; 53:250–264.
13. Yki-Järvinen H, Koivisto VA. Natural course of insulin resistance in type I diabetes. N Engl J Med. 1986; 315:224–230.
14. The relationship of glycemic exposure (HbA1c) to the risk of development and progression of retinopathy in the diabetes control and complications trial. Diabetes. 1995; 44:968–983.
15. Effect of intensive therapy on residual beta-cell function in patients with type 1 diabetes in the diabetes control and complications trial: a randomized, controlled trial: the Diabetes Control and Complications Trial Research Group. Ann Intern Med. 1998; 128:517–523.
16. Wallerath T, Kunt T, Forst T, Closs EI, Lehmann R, Flohr T, Gabriel M, Schäfer D, Göpfert A, Pfützner A, et al. Stimulation of endothelial nitric oxide synthase by proinsulin C-peptide. Nitric Oxide. 2003; 9:95–102.
17. Panero F, Novelli G, Zucco C, Fornengo P, Perotto M, Segre O, Grassi G, Cavallo-Perin P, Bruno G. Fasting plasma C-peptide and micro- and macrovascular complications in a large clinic-based cohort of type 1 diabetic patients. Diabetes Care. 2009; 32:301–305.
18. Vasic D, Walcher D. C-peptide: a new mediator of atherosclerosis in diabetes. Mediators Inflamm. 2012; 2012:858692.
19. Unger RH, Grundy S. Hyperglycaemia as an inducer as well as a consequence of impaired islet cell function and insulin resistance: implications for the management of diabetes. Diabetologia. 1985; 28:119–121.
20. Leahy JL, Cooper HE, Deal DA, Weir GC. Chronic hyperglycemia is associated with impaired glucose influence on insulin secretion: s study in normal rats using chronic in vivo glucose infusions. J Clin Invest. 1986; 77:908–915.
21. Eizirik DL, Korbutt GS, Hellerström C. Prolonged exposure of human pancreatic islets to high glucose concentrations in vitro impairs the beta-cell function. J Clin Invest. 1992; 90:1263–1268.
22. Carlson MG, Campbell PJ. Intensive insulin therapy and weight gain in IDDM. Diabetes. 1993; 42:1700–1707.
23. Weight gain associated with intensive therapy in the diabetes control and complications trial: the DCCT Research Group. Diabetes Care. 1988; 11:567–573.
24. Leslie RD, Taylor R, Pozzilli P. The role of insulin resistance in the natural history of type 1 diabetes. Diabet Med. 1997; 14:327–331.
25. Greenbaum CJ. Insulin resistance in type 1 diabetes. Diabetes Metab Res Rev. 2002; 18:192–200.
26. Reaven GM. Banting lecture 1988: role of insulin resistance in human disease. Diabetes. 1988; 37:1595–1607.
27. DeFronzo RA, Ferrannini E. Insulin resistance: a multifaceted syndrome responsible for NIDDM, obesity, hypertension, dyslipidemia, and atherosclerotic cardiovascular disease. Diabetes Care. 1991; 14:173–194.
28. Greenbaum CJ, Sears KL, Kahn SE, Palmer JP. Relationship of beta-cell function and autoantibodies to progression and nonprogression of subclinical type 1 diabetes: follow-up of the Seattle Family Study. Diabetes. 1999; 48:170–175.
29. Achenbach P, Bonifacio E, Koczwara K, Ziegler AG. Natural history of type 1 diabetes. Diabetes. 2005; 54:S25–S31.