Abstract
The interactions between the tumor microenvironment and tumor cells determine the behavior of the primary tumors. Whether cancer-associated fibroblasts (CAF) have a tumor progressive or a protective role likely depends on the type of tumor cells and the CAF subpopulation. In the present study, we analyzed the prognostic significance of CAF subpopulations in colorectal cancer (CRC). CAF phenotypes were analyzed in 302 CRC patients by using antibodies against podoplanin (PDPN), α-smooth muscle actin (α-SMA), and S100A4. The relationship between the CAF phenotypes and 11 clinicopathological parameters were evaluated and their prognostic significance was analyzed from the disease-free and overall survival times. We observed that at the tumor invasive front, PDPN CAFs were present in 40% of the cases, and S100A4 or α-SMA CAFs were detected in all the cases. PDPN/S100A4 and α-SMA/S100A4 dual-stained CAFs were observed in 10% and 40% of the cases, respectively. The PDPN+ CAFs were associated with 6 favorable clinicopathological parameters and prolonged disease-free survival time. The PDPN-/α-SMAhigh CAFs were associated with 6 aggressive clinicopathological parameters and tended to exhibit shorter disease-free survival time. On the other hand, the PDPN-/S100A4high CAFs were associated with 2 tumor progression parameters, but not with disease prognosis. The PDPN+ CAF phenotype is distinct from the α-SMA or S100A4 CAFs in that it is associated with less aggressive tumors and a favorable prognosis, whereas the PDPN-/α-SMAhigh or PDPN-/S100A4high CAFs are associated with tumor progression in CRC. These findings suggest that CAFs can be a useful prognostic biomarker or potential targets of anti-cancer therapy in CRC.
The continuous cross-talk between cancer cells and their microenvironments plays an active role in tumor development, invasion and metastasis. The non-neoplastic stromal compartment is constituted by the cellular and extracellular matrix and cancer-associated fibroblasts (CAFs) are the most prominent cell type within the tumor stroma (1). Although the microenvironment usually promotes tumor progression, recent studies suggested the protective role of CAFs depending on the tumor types and the CAF subpopulation. CAFs that express α-smooth muscle actin (α-SMA) (2), vimentin (3), or fibroblast activation protein (FAP) (4) were associated with a shorter disease-free survival time in CRC. Although the microenvironment usually promotes tumor progression, under certain circumstances excess stroma might inhibit tumor invasion (5). A few studies have shown that CAFs play a role in preventing tumor progression and are related to a favorable prognosis. One particular report highlighting the beneficial effects of the fibroblastic reaction in a rat colon cancer model suggested that the fibrous encapsulation could lead to tumor regression (6). On the contrary, others have argued that such an encapsulation might benefit the tumor by reducing access to the host immune cells (7). These studies suggested that the effect of CAFs likely depends on the type of tumor cells and the CAF subpopulation.
Podoplanin (PDPN) is a 38-kDa mucin-type transmembrane glycoprotein with extensive O-glycosylation and high sialic acid content, and has been implicated in tumor progression (8). PDPN is upregulated on tumor cells in several cancer types, is often expressed at the leading invasive edge of tumors, and appears to play a role in epithelial-to-mesenchymal transition, invasion, and metastasis. PDPN is also upregulated by the CAFs in the stroma surrounding various tumors, including colorectal cancers (9). Although there is a lot of data on the tumor-promoting effects of PDPN+ CAFs, one particular study reported a contradictory protective role of PDPN+ CAFs in CRC (10). The investigators reported the associated PDPN+ CAFs with a favorable prognosis in CRC, and observed an enhanced cancer cell migration on the knockdown of PDPN in CAFs (10). Thus, further studies are needed to clarify the role of PDPN+ CAFs in CRC. CAFs expressing α-SMA, a myofibroblast marker, facilitated cancer progression in many different cancers including CRC. However, the relationship with other CAF markers has not yet been studied. In addition, the association of fibroblast-specific protein 1 (FSP1 or S100A4)-expressing CAFs with tumor progression and poor prognosis has been found in many different cancers (11, 12), but this has not been confirmed in CRC tissues.
The aim of the present study was to characterize the CAF subpopulations and to evaluate their roles in tumor protection or progression in CRC. We identified PDPN-, α-SMA-, or S100Aexpressing CAFs using immunohistochemistry (IHC) in CRC tissues and evaluated their relationship with the clinicopathological parameters and prognosis in CRC patients.
Paraffin-embedded tissues were obtained from the Department of Pathology of the Chungbuk National University Hospital. Three hundred and two CRC patients (mean age, 63.6 ± 11.1 yr; range 25-86 yr; male-to-female ratio, 181:121), who underwent complete resection (R0) and were followed for more than 5 yr, were enrolled in this study. The patients did not receive any chemotherapy or radiation therapy before surgery. The CRCs were located in the right colon in 69 cases, in the left colon in 70 cases, and in the rectum in 160 cases (Table 1).
For each tumor, medical records and tissue slides were re-evaluated consistently by one pathologist. Tumor size, cell grade, depth of tumor invasion, lymph node or distant metastasis, and microsatellite status (characterized in 146 cases) were assessed. Microsatellite status was evaluated by demonstrating the expression of specific microsatellite repeats by polymerase chain reaction (PCR). Briefly, PCR was performed using DNA extracted from resected CRC tissues about two mononucleotide repeats (BAT25, BAT26) and three dinucleotide repeats (D5S346, D2S123, D17S250). MSI High (MSI-H) indicates that 2 or more microsatellites show instability, MSI low (MSI-L) indicates only one microsatellite shows instability and microsatellite stable (MSS) indicates the absence of unstable microsatellites (13). We categorized the CRCs as MSI-H versus MSI-L or MSS (14). The stages were defined according to the TNM staging system based on the American Joint Committee on Cancer (AJCC) 7th edition (15). We also investigated invasion into lymphatics or vessels and perineural invasion. Types of tumor growth (expanding and infiltrative) and the presence of tumor budding (defined as the presence of clusters of less than 5 tumor cells (16)) were evaluated. The number of buds was counted in one field of the invasive margin that demonstrated intense tumor budding in a high power field (× 200 magnification). A field with ≥ 1 bud was considered positive.
After the slide review, 3-mm tissue micro-arrays (TMA) were reconstructed. Regions near the tumor center and near the invasive margin of CRC, which demonstrated the lowest degree of differentiation and had an abundance of mitotic cells, were chosen from the original blocks. An experienced pathologist marked these representative areas on hematoxylin and eosin (H&E)-stained slides from the selected paraffin blocks. Sections of 4 µm thickness were cut and IHC was performed using the BenchMark XT automated IHC stainer (Ventana Medical Systems, Inc., Tucson, AZ) and the Ventana Ultraview DAB Kit (Ventana Medical Systems) was used for detection. For IHC, the sections were deparaffinized using the EZ Prep solution and antigen retrieval was done using the CC1 standard automated process (pH 8.4 buffer containing Tris/Borate/EDTA). The DAB inhibitor (3% H2O2 endogenous peroxidase) was blocked for 4 min at 37℃. The slides were incubated with the respective primary antibodies for 30 min at 37℃, followed by incubation with the secondary antibody (Universal HRP Multimer) for 8 min at 37℃. Subsequently, the slides were treated with the DAB + H2O2 substrate for 8 min followed by counterstaining with hematoxylin and the bluing reagent at 37℃. The reaction buffer (pH 7.6 Tris buffer) was used as a wash solution. The primary antibodies used included: anti-podoplanin (Dako Cytomation, Glostrup, Denmark, 1:50), anti-α-SMA (Dako Cytomation, Glostrup, Denmark, 1:400), and anti-S100A4 (Dako Cytomation, Glostrup, Denmark, 1:800). Dual staining of podoplanin (cytoplasm, Red) plus S100A4 (nucleus, DAB/brown) and α-SMA (cytoplasm, Red) plus S100A4 (nucleus, DAB/brown) were also performed. For the dual staining, the slides were incubated with primary antibodies (S100A4) for 32 min at 37℃, followed by the secondary antibody of Universal HRP Multimer for 8 min at 37℃. The slides were then treated with the DAB substrate for 8 min at 37℃ followed by denaturation at 90℃ for 4 min. The slides were incubated with primary antibodies (anti-podoplanin or α-SMA) for 32 min at 37℃ and the secondary antibody of Universal AP Multimer for 12 min at 37℃. Slides were sequential treated with UV Red enhancer for 4 min at 37℃, UV Fast Red A plus UV Red naphthol for 8 min at 37℃, and UV Fast Red B for 8 min at 37℃, and finally counterstained using hematoxylin for 4 min and bluing reagent for 4 min at 37℃.
For the identification of PDPN-, α-SMA-, and S100A4-expressing CAFs among α-SMA-positive smooth muscles of muscularis mucosa, muscularis propria and blood vessels, PDPN-positive lymphatic endothelial cells, and S100A4-expressing inflammatory cells, the distinct histological morphology was carefully examined. Muscularis mucosa and muscularis propria appeared as smooth muscle bundles and the blood vessels and lymphatic channels were identified by their vascular or lymphatic lumens. In addition, the CAFs appeared as spindle shaped stromal cells with long cytoplasmic extensions, which were different from the lymphocytes characterized by a small, round nucleus with scanty cytoplasm (Fig. 1).
The stained slides were semiquantitatively evaluated according to the method described previously (17); stromal staining was semiquantitatively assessed as 0 (absence of or weak immunostaining in < 1% of the tumor stroma), 1 (focal positivity in 1%-10% of stromal cells), 2 (positive immunostaining in 11%-50% of stromal cells, and 3 (51%-100% stromal staining). Assessment of the entire tumor was made at the tumor center and the invasive front, or areas of active tumor growth. For statistical analysis, PDPN+ CAFs were classified as negative (score 0) and positive (score 1-3), while α-SMA or S100A4 CAFs were classified as low expression (score 0 or 1) and high expression (score 2 or 3).
The patient outcomes were overall survival and disease-free survival as measured from the time of surgery and were estimated by the Kaplan-Meier method. The log-rank test and the Cox proportional hazard model were used to conduct univariate and multivariate analyses. The relationship between the CAF staining intensity (measured semiquantitatively) and the clinicopathological parameters were analyzed using the Fisher's exact test, the chi-square test, Student's t test, or the Mann-Whitney U test. All statistical tests were 2-sided, and statistical significance was accepted at P < 0.05. All analyses were performed using the SPSS version 12.0 (SPSS Inc., Chicago, IL, USA).
Representative pictures of PDPN, α-SMA, and S100A4 staining are shown in Fig. 1. All the study cases presented with CAFs expressing S100A4 or α-SMA in the stroma of both the tumor center and the invasive front, while 74% of PDPN+ CAFs were identified at the tumor center and 42% were at the invasive front among the cases examined. Dual-stained CAFs expressing PDPN plus S100A were less frequently identified (10%) than those expressing α-SMA plus S100A4 (40%) at the tumor invasive front. The immunoreactivity for PDPN was exclusively confined to the stromal fibroblasts of both the intra- and the peri-tumoral stroma, and was sparse in the stromal cells that surrounded the cancer cell budding in the tumor nests of the invasive front. On the other hand, CAFs expressing α-SMA or S100A4 demonstrated a similar pattern, both at the tumor center and at the invasive margin (Table 2).
PDPN+ CAFs at the tumor invasive front were inversely related to the pre-operative carcinoembryonic antigen (CEA) level (P = 0.020), tumor size (P = 0.039), T-stage (P = 0.002), and AJCC stage (P = 0.033). They were frequently observed in tumors that exhibited an expanding tumor border (P = 0.001), absence of tumor budding (P = 0.001), and high MSI (P = 0.010). The presence of PDPN- CAFs with high α-SMA expression at the tumor invasive front was associated with the pre-operative CEA level (P = 0.032), tumor size (P = 0.011), T-stage (P = 0.001), and AJCC stage (P = 0.020). These tumors frequently presented with an infiltrative tumor border (P = 0.004), presence of tumor budding (P = 0.001), and low MSI or microsatellite stable tumors (P = 0.010). In addition, CAFs with PDPN- and high S100A4 expression at the tumor invasive front were associated with lymphovascular invasion (P = 0.013) and the presence of tumor budding (P = 0.002) (Table 3).
During the follow up period of 58.6 months (mean), recurrence and death occurred in 58 (19.2%) patients and 59 (19.5%) patients, respectively. Univariate analysis revealed that PDPN+ CAFs at the tumor center were significantly associated with prolonged disease-free survival time (P = 0.042 by log rank test, Fig. 2A). Multivariate analysis revealed that the AJCC stage, perineural invasion, and PDPN- CAFs at the tumor center were independent risk factors of disease-free survival (Table 4). Notably, the PDPN+ CAFs at the invasive front demonstrated a prolonged disease-free survival time, although without statistical significance (Fig. 2B). Patients that expressed PDPN-/α-SMAhigh CAFs presented with a shorter disease-free survival time but it was statistically insignificant (Fig. 2C). The presence of PDPN-/S100A4high CAFs did not show any relationship with disease-free survival (Fig. 2D). In this study, there was no association between the CAF subtypes observed and overall survival.
CAFs are fibroblasts that reside within the tumor stroma and they include subpopulations that express α-SMA, vimentin, S100A4, FAP, fibroblast-associated antigen, and PDPN (18). CAFs may actively contribute to tumor growth and malignant behavior; they can support tumor epithelial proliferation, angiogenesis, and invasion. Desmoplasia at the tumor edge is associated with advanced disease stage (19), and a high tumor-stroma ratio is clearly related to poor prognosis (20). Furthermore, tumors with abundant myofibroblasts stained with α-SMA (2), vimentin (3), or FAP (4) were associated with a shorter diseasefree survival time in CRC. However, the CAF subpopulation in certain tumors was associated with less malignant tumors and a favorable prognosis. These data illustrate that the effect of CAFs likely depends on the type of tumor cells and the immune phenotypes of the CAFs.
This study demonstrates the heterogeneity of CAFs, thereby indicating the existence of different subpopulations of stromal fibroblasts in tumors based on IHC using various fibroblastic markers. PDPN+ CAFs, which were abundantly present at the tumor center rather than at the invasive margin, are associated with favorable clinicopathological parameters and can predict a prolonged disease-free survival time in CRC. On the other hand, PDPN-/α-SMAhigh CAFs were associated with malignant tumors and indicated a shorter disease-free survival time. In addition, PDPN-/S100A4high CAFs were associated with tumor budding and lymphovascular invasion.
PDPN, a mucin-type transmembrane glycoprotein, is expressed in lymphatic endothelial cells as well as in cancer cells and CAFs in many different tumors. It has been associated with poor outcomes in patients with lung adenocarcinoma (21), esophageal adenocarcinoma (22), oral squamous cell carcinoma (23), and invasive breast cancer (24). On the contrary, in cervical cancer, CAFs are associated with improved disease-specific survival and disease-free survival (25). In CRC, the patterns of PDPN expression and their prognostic significance differed based on the studies. One study reported that PDPN is absent in normal colonic epithelia but is expressed in CRC cells (9), while another study reported that it is not expressed in CRC cells (10). In this study, PDPN+ tumor cells were not identified in the CRC tissues. Additionally, the relationship between the presence of PDPN+ CAFs and prognosis differed in several studies. Kitano et al. (9) showed that these CAFs were associated with a poor prognosis in adenocarcinoma, including CRC, while Yamanashi et al. (10) have shown that PDPN+ CAFs correlate with a favorable prognosis in CRC. They reported that PDPN+ CAFs significantly correlated with a shallower depth of tumors and associated with a prolonged disease-free survival time. Additionally, they found that the invasion of CRC cells was augmented upon co-culture with fibroblasts in which podoplanin expression was reduced by siRNA. These results mirror our observations demonstrating the favorable effects of PDPN+ CAFs on disease-free survival in CRC.
In our study, PDPN- and α-SMAhigh CAFs were associated with advanced tumors and shorter disease-free survival times, although the association was not statistically significant. A previous study showed that tumors with abundant myofibroblasts stained with α-SMA (2) were associated with a shorter disease-free survival time in stage II and III CRC. The effect of α-SMA-expressing CAFs was less significant than that of PDPN+ CAFs in our study.
The usefulness of S100A4 as a marker for CAFs and the role of CAFs with an increased expression of S100A4 in CRC have not been clarified. Studies highlighting the clinical significance of fibroblast-specific protein 1 (FSP1 or S100A4)-expressing stroma have been reported (11, 12). A study by Sugimoto and colleagues demonstrated the presence of unique S100A4-expressing CAFs distinct from α-SMA positive myofibroblasts in mouse tumor stroma (26). One study illustrated that S100A4 identifies a unique population of fibroblasts with a minimal overlap with other markers for CAFs (26), while another study indicated that S100A4 is not specific to CAFs but is also expressed by many different kinds of cells, such as lymphocytes, macrophages, neutrophilic granulocytes, and endothelial cells (27). In our present study, we found an association between CAFs with a high S100A4 expression and the degree of lymphocyte infiltration (data not shown). PDPN-/S100A4high CAFs were found associated with lymphovascular invasion and tumor budding in tumor tissues, but not with patient prognosis. Based on these findings, we propose that S100A4-expressing CAFs may play a role in tumor progression, although distinguishing S100A4+ CAFs from other inflammatory cells in CRC is very difficult.
The location of CAFs in the tumor also differs based on the subpopulation. In this study, PDPN staining was more intense towards the tumor center than at the invasive edge, which was consistent with a previous study (10). Also, the presence of PDPN+ CAFs at the invasive front was associated with favorable clinicopathological parameters and PDPN+ CAFs at the tumor center was a favorable prognostic factor for disease-free survival time. These findings suggest that PDPN could be a protective barrier against cancer invasion. On the other hand, CAFs stained with S100A4 or α-SMA appeared uniform throughout the stroma, irrespective of their location in the tumor center or at the invasive edge, similar to previous observations with FAP+ CAFs (17).
Stromal fibroblasts within tumors are designated by only partly overlapping marker expression. The heterogeneity in marker expression may be explained in part by the diverse origins of CAFs (28). In our study, only 40%-50% and 10%-20% of cases presented CAFs with dual stained α-SMA/S100A4 and PDPN/S100A4, respectively. The heterogeneity observed in CAFs impedes the use of a single marker to identify all CAFs, and none of these markers by themselves are unique to CAFs (26).
MSS and MSI CRCs are increasingly being recognized as distinct entities, with significantly different pathological characteristics, behaviors, and prognosis. MSI was significantly associated with a reduced malignant potential (29). In accordance with these reports, we found that MSI-high CRCs were associated with PDPN+ CAFs, which have favorable properties in CRC, and MSS or MSI-low CRCs were associated with PDPN-/α-SMAhigh CAFs, which were associated with tumor progression.
Studies on the role of CAFs in human cancers have clinical significance because therapeutic strategies that target fibroblasts within the tumor stroma offer an alternate treatment option. The in vitro analyses following a therapeutic trial targeting fibroblast growth factor receptor signaling (30) have suggested a promising new approach in treating aggressive cancers. This study showed that different CAFs residing in the tumor stroma have opposing roles in tumor protection or tumor progression, and targeting specific subpopulations of CAFs is important for the development of effective treatments.
Notes
References
1. Pietras K, Ostman A. Hallmarks of cancer: interactions with the tumor stroma. Exp Cell Res. 2010; 316:1324–1331. PMID: 20211171.
2. Tsujino T, Seshimo I, Yamamoto H, Ngan CY, Ezumi K, Takemasa I, Ikeda M, Sekimoto M, Matsuura N, Monden M. Stromal myofibroblasts predict disease recurrence for colorectal cancer. Clin Cancer Res. 2007; 13:2082–2090. PMID: 17404090.
3. Ngan CY, Yamamoto H, Seshimo I, Tsujino T, Man-i M, Ikeda JI, Konishi K, Takemasa I, Ikeda M, Sekimoto M, et al. Quantitative evaluation of vimentin expression in tumour stroma of colorectal cancer. Br J Cancer. 2007; 96:986–992. PMID: 17325702.
4. Cheng JD, Dunbrack RL Jr, Valianou M, Rogatko A, Alpaugh RK, Weiner LM. Promotion of tumor growth by murine fibroblast activation protein, a serine protease, in an animal model. Cancer Res. 2002; 62:4767–4772. PMID: 12183436.
5. Grégoire M, Lieubeau B. The role of fibroblasts in tumor behavior. Cancer Metastasis Rev. 1995; 14:339–350. PMID: 8821094.
6. Martin MS, Caignard A, Hammann A, Pelletier H, Martin F. An immunohistological study of cells infiltrating progressive and regressive tumors induced by two variant subpopulations of a rat colon cancer cell line. Int J Cancer. 1987; 40:87–93. PMID: 3298078.
7. Liotta LA, Rao CN, Barsky SH. Tumor invasion and the extracellular matrix. Lab Invest. 1983; 49:636–649. PMID: 6317982.
8. Kono T, Shimoda M, Takahashi M, Matsumoto K, Yoshimoto T, Mizutani M, Tabata C, Okoshi K, Wada H, Kubo H. Immunohistochemical detection of the lymphatic marker podoplanin in diverse types of human cancer cells using a novel antibody. Int J Oncol. 2007; 31:501–508. PMID: 17671675.
9. Kitano H, Kageyama S, Hewitt SM, Hayashi R, Doki Y, Ozaki Y, Fujino S, Takikita M, Kubo H, Fukuoka J. Podoplanin expression in cancerous stroma induces lymphangiogenesis and predicts lymphatic spread and patient survival. Arch Pathol Lab Med. 2010; 134:1520–1527. PMID: 20923309.
10. Yamanashi T, Nakanishi Y, Fujii G, Akishima-Fukasawa Y, Moriya Y, Kanai Y, Watanabe M, Hirohashi S. Podoplanin expression identified in stromal fibroblasts as a favorable prognostic marker in patients with colorectal carcinoma. Oncology. 2009; 77:53–62. PMID: 19556810.
11. Schulte J, Weidig M, Balzer P, Richter P, Franz M, Junker K, Gajda M, Friedrich K, Wunderlich H, Östman A, et al. Expression of the E-cadherin repressors Snail, Slug and Zeb1 in urothelial carcinoma of the urinary bladder: relation to stromal fibroblast activation and invasive behaviour of carcinoma cells. Histochem Cell Biol. 2012; 138:847–860. PMID: 22820858.
12. Vered M, Dayan D, Yahalom R, Dobriyan A, Barshack I, Bello IO, Kantola S, Salo T. Cancer-associated fibroblasts and epithelial-mesenchymal transition in metastatic oral tongue squamous cell carcinoma. Int J Cancer. 2010; 127:1356–1362. PMID: 20340130.
13. Boland CR, Thibodeau SN, Hamilton SR, Sidransky D, Eshleman JR, Burt RW, Meltzer SJ, Rodriguez-Bigas MA, Fodde R, Ranzani GN, et al. A National Cancer Institute Workshop on Microsatellite Instability for cancer detection and familial predisposition: development of international criteria for the determination of microsatellite instability in colorectal cancer. Cancer Res. 1998; 58:5248–5257. PMID: 9823339.
14. Umar A, Boland CR, Terdiman JP, Syngal S, de la Chapelle A, Rüschoff J, Fishel R, Lindor NM, Burgart LJ, Hamelin R, et al. Revised Bethesda Guidelines for hereditary nonpolyposis colorectal cancer (Lynch syndrome) and microsatellite instability. J Natl Cancer Inst. 2004; 96:261–268. PMID: 14970275.
15. American Joint Committee on Cancer. Manual for staging of cancer. 7th ed. Berlin: Springer;2009.
16. Wang LM, Kevans D, Mulcahy H, O'Sullivan J, Fennelly D, Hyland J, O'Donoghue D, Sheahan K. Tumor budding is a strong and reproducible prognostic marker in T3N0 colorectal cancer. Am J Surg Pathol. 2009; 33:134–141. PMID: 18971777.
17. Henry LR, Lee HO, Lee JS, Klein-Szanto A, Watts P, Ross EA, Chen WT, Cheng JD. Clinical implications of fibroblast activation protein in patients with colon cancer. Clin Cancer Res. 2007; 13:1736–1741. PMID: 17363526.
18. McAnulty RJ. Fibroblasts and myofibroblasts: their source, function and role in disease. Int J Biochem Cell Biol. 2007; 39:666–671. PMID: 17196874.
19. Nakayama H, Enzan H, Miyazaki E, Naruse K, Kiyoku H, Hiroi M. The role of myofibroblasts at the tumor border of invasive colorectal adenocarcinomas. Jpn J Clin Oncol. 1998; 28:615–620. PMID: 9839502.
20. Mesker WE, Junggeburt JM, Szuhai K, de Heer P, Morreau H, Tanke HJ, Tollenaar RA. The carcinoma-stromal ratio of colon carcinoma is an independent factor for survival compared to lymph node status and tumor stage. Cell Oncol. 2007; 29:387–398. PMID: 17726261.
21. Kawase A, Ishii G, Nagai K, Ito T, Nagano T, Murata Y, Hishida T, Nishimura M, Yoshida J, Suzuki K, et al. Podoplanin expression by cancer associated fibroblasts predicts poor prognosis of lung adenocarcinoma. Int J Cancer. 2008; 123:1053–1059. PMID: 18546264.
22. Tong L, Yuan S, Feng F, Zhang H. Role of podoplanin expression in esophageal squamous cell carcinoma: a retrospective study. Dis Esophagus. 2012; 25:72–80. PMID: 21895849.
23. Foschini MP, Leonardi E, Eusebi LH, Farnedi A, Poli T, Tarsitano A, Cocchi R, Marchetti C, Gentile L, Sesenna E, et al. Podoplanin and E-cadherin expression in preoperative incisional biopsies of oral squamous cell carcinoma is related to lymph node metastases. Int J Surg Pathol. 2013; 21:133–141. PMID: 23349470.
24. Schoppmann SF, Berghoff A, Dinhof C, Jakesz R, Gnant M, Dubsky P, Jesch B, Heinzl H, Birner P. Podoplanin-expressing cancer-associated fibroblasts are associated with poor prognosis in invasive breast cancer. Breast Cancer Res Treat. 2012; 134:237–244. PMID: 22350732.
25. Dumoff KL, Chu CS, Harris EE, Holtz D, Xu X, Zhang PJ, Acs G. Low podoplanin expression in pretreatment biopsy material predicts poor prognosis in advanced-stage squamous cell carcinoma of the uterine cervix treated by primary radiation. Mod Pathol. 2006; 19:708–716. PMID: 16528371.
26. Sugimoto H, Mundel TM, Kieran MW, Kalluri R. Identification of fibroblast heterogeneity in the tumor microenvironment. Cancer Biol Ther. 2006; 5:1640–1646. PMID: 17106243.
27. Boye K, Maelandsmo GM. S100A4 and metastasis: a small actor playing many roles. Am J Pathol. 2010; 176:528–535. PMID: 20019188.
28. Ostman A, Augsten M. Cancer-associated fibroblasts and tumor growth: bystanders turning into key players. Curr Opin Genet Dev. 2009; 19:67–73. PMID: 19211240.
29. Pino MS, Kikuchi H, Zeng M, Herraiz MT, Sperduti I, Berger D, Park DY, Iafrate AJ, Zukerberg LR, Chung DC. Epithelial to mesenchymal transition is impaired in colon cancer cells with microsatellite instability. Gastroenterology. 2010; 138:1406–1417. PMID: 20026115.
30. Brennen WN, Isaacs JT, Denmeade SR. Rationale behind targeting fibroblast activation protein-expressing carcinoma-associated fibroblasts as a novel chemotherapeutic strategy. Mol Cancer Ther. 2012; 11:257–266. PMID: 22323494.
Fig. 1
Immunohistochemistry for different markers of cancer associated fibroblasts (CAFs) in colorectal carcinomas (×400). (A) Podoplanin (PDPN) expression was not observed in the normal stromal cells, except for the lymphatic vessels (arrow). (B) α-SMA expression in smooth muscle cells (arrow) and vessels (empty arrow). (C) S100A4 expression in inflammatory cells (arrow). (D) α-SMA-positive myofibroblasts appeared as spindle shaped stromal cells with long cytoplasmic extensions. (E) S100A4-positive CAF (arrow) in the tumor stroma surrounding the carcinoma cells. (F) Dual stained CAFs with PDPN (empty arrow) and S100A4 (arrow). (G) Dual stained CAFs with α-SMA (empty arrow) and S100A (arrow).
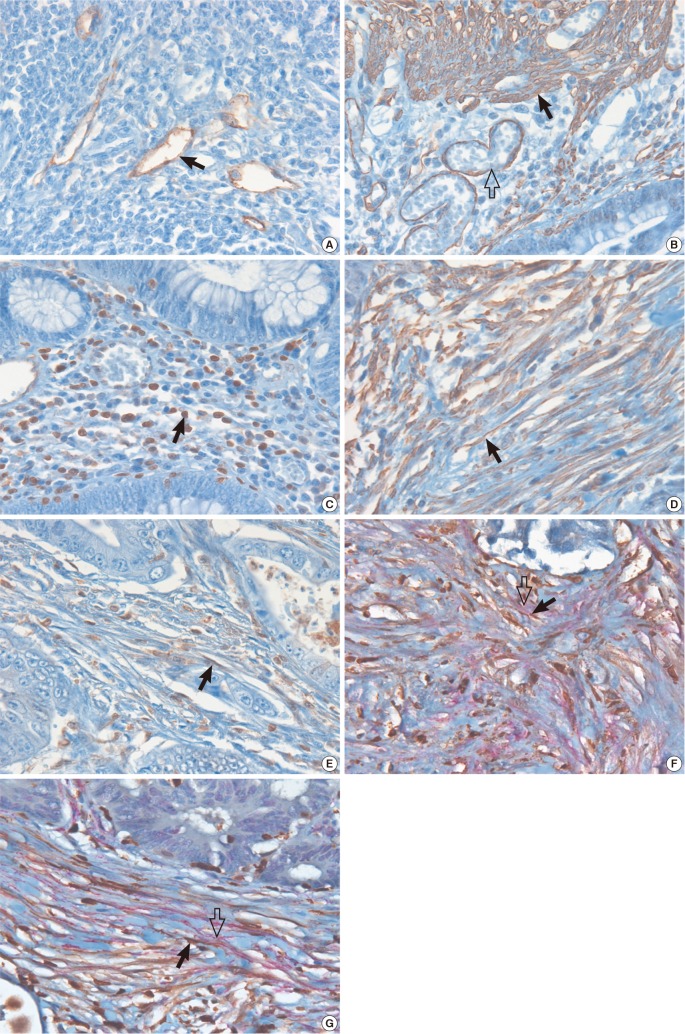
Fig. 2
Disease-free survival curves based on the CAF subpopulation, with PDPN+ at the tumor center (A), PDPN+ at the invasive front (B), PDPN-/α-SMAhigh (C), and PDPN-/S100A4high (D) in colorectal cancers.
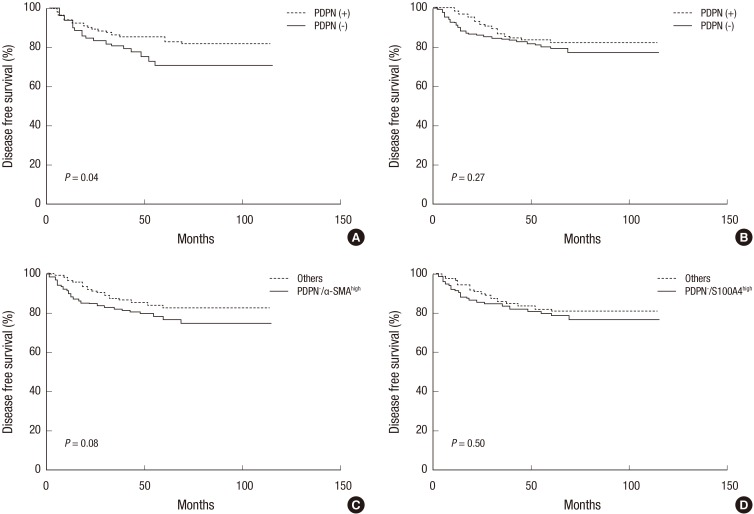
Table 2
Percentage of cases presenting with cancer associated fibroblasts expressing podoplanin (PDPN), α-SMA, S100A4, PDPN/S100A4, and α-SMA/S100A4 in colorectal cancers

Table 3
Relation between clinicopathological parameters and cancer associated fibroblast subpopulations in colorectal carcinomas
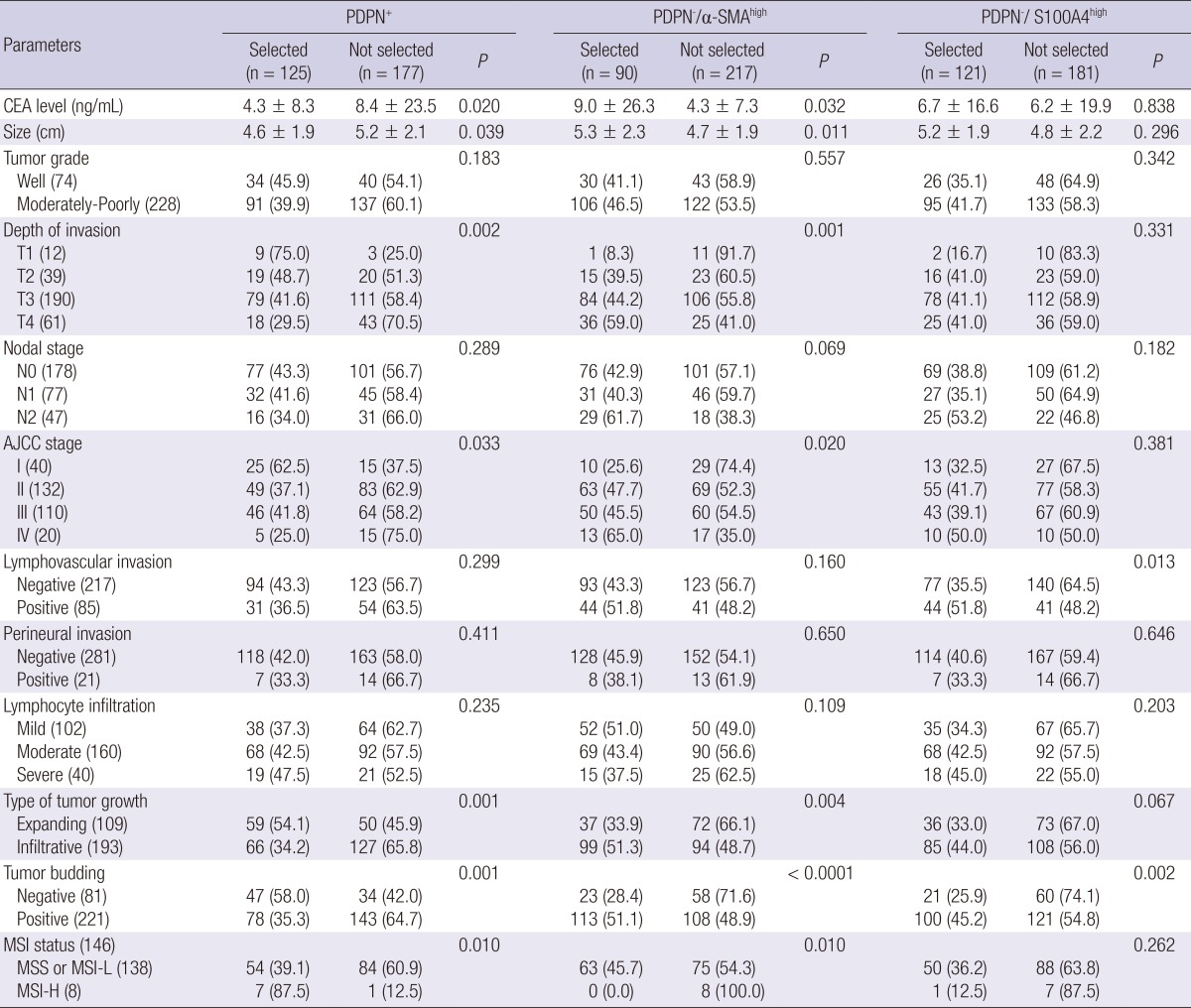
PDPN+, podoplanin positive; PDPN-/α-SMAhigh; podoplanin negative and α-SMA high expression; PDPN-/S100A4high, podoplanin negative and S100A4 high expression CEA, carcinoembryonic antigen serum level; MSI, microsatellite instability; MSS, microsatellite stable; MSI-L. microsatellite instable-low; MSI-H, microsatellite instable-high.