Abstract
This study aimed to evaluate the effects of volume adapted re-planning for radiotherapy (RT) after gross total resection (GTR) for glioblastoma. Nineteen patients with glioblastoma who underwent GTR and postoperative RT were analyzed. The volumes of the surgical cavity on computed tomography (CT) obtained one day after GTR (CT0), the first RT simulation CT (sim-CT1), and the second simulation CT for the boost RT plan (sim-CT2) were compared. The boost RT plan was based on the surgical cavity observed on the sim-CT2 (boost RTP2) and was compared with that based on the surgical cavity observed on the sim-CT1 (boost RTP1). The volume reduction ratios were 14.4%-51.3% (median, 29.0%) between CT0 and sim-CT1 and -7.9%-71.9% (median, 34.9%) between sim-CT1 and sim-CT2 (P < 0.001). The normal brain volumes in boost RTP1 were significantly reduced in boost RTP2, especially at high dose levels. Target volume in sim-CT2 which was not covered with the boost RTP1, developed in five cases (26.3%). The surgical cavity volume was reduced following surgery in patients with glioblastoma who underwent GTR. The application of volume-adapted re-planning during RT could decrease the irradiated volume of normal brain and prevent a target miss for boost RT.
Radiotherapy (RT) is an essential modality for the treatment of the patients with glioblastoma, and there have been many technical advances in RT techniques that have prolonged the survival period. Initially, the radiation volume encompassed the whole brain; however, as the main recurrence sites are just around the tumor, local brain RT has been commonly applied (1-4).
The gross tumor volume (GTV) for postoperative RT is defined as the surgical cavity and residual tumor. When the tumor is grossly totally resected, the GTV includes the surgical cavity only. This definition is consistent with that employed in the Radiation Therapy Oncology Group glioblastoma protocols (5). The recommended radiation dose is 60 Gy, and most institutes use the so-called "shrink-field technique" where the initial radiation field includes the clinical target volume (CTV) plus a margin up to 40-50 Gy; after that, the second radiation field is reduced to include the GTV with a dose of 10-20 Gy (6). During the entire RT period, RT planning computed tomography (CT) is performed several times, and a volumetric change in the surgical defect between the initial CT and follow-up scans is sometimes observed.
The purpose of the current study was to evaluate the changes in treatment volume according to changes in surgical defect volume during RT for patients with totally resected glioblastoma and to determine the effects of volume-adapted re-planning for RT.
We retrospectively analyzed 19 patients with glioblastoma who underwent surgical resection and postoperative RT from April 2007 to February 2011. All patients underwent gross total resection (GTR), defined by operative records and post-operative magnetic resonance imaging (MRI) demonstrating no residual tumor.
For postoperative RT, the first simulation CT was performed 3 to 4 weeks after surgery, and RT was planned using ADAC Pinnacle3 version 8.0 m Software (Philips Medical Systems, Andover, MA, USA). The second simulation CT for shrink-field technique was performed in the fifth week of RT.
GTV for initial RT (GTV1) was defined as the surgical defect in the first simulation CT (sim-CT1) (Fig. 1). The CTV for the initial RT was the GTV1 plus a 2.0 cm margin, and the planning target volume (PTV) was the CTV plus a 0.5 cm margin. The prescription dose to the PTV was 50 Gy with a daily fraction of 2 Gy. Following this treatment, the shrink-field technique was performed for a 10-Gy boost RT.
Two techniques could be performed for the boost RT. One in which the CTV for the boost RT was defined using the initial GTV1, itself derived from the sim-CT1. In this case, the CTV for boost RT was the GTV1 plus a 0.5 cm margin, and this was defined as the CTV1. The other method used at our institution, defined the CTV for the boost RT from a newly obtained simulation CT. In this case, the GTV for the boost RT (GTV2) was defined as the surgical defect in the second simulation CT (sim-CT2). The CTV for the boost RT (CTV2) was the GTV2 plus 0.5 cm. The PTV for the boost RT was a 0.5 cm margin from the CTV1 or CTV2. The CT obtained one day after surgery was defined as the CT0 and the entire treatment schedule and definitions of parameters are summarized in Fig. 1.
A boost RT plan (boost RTP2) based on the GTV2 derived from the sim-CT2 was compared with the boost RT plan (boost RTP1) based on the GTV1 (Fig. 2). Both boost RTP1 and boost RTP2 had identical treatment planning conditions, including the reference point of International Commission on Radiation Units and Measurements, type of linear accelerator, radiation energy, radiation beam number, and directions of the radiation beams. The bony structures in the sim-CT1 and the sim-CT2 had same position using the identical thermoplastic mask. The inclusion of the CTV2 in the 95% isodose curve in the boost RTP1 was determined, and the volume of the CTV2 not covered by a 95% isodose curve in boost RTP1 was measured and defined as missed target volume (Fig. 2C). The normal brain volume was considered as the whole brain volume minus the GTV2 in sim-CT2, and the normal brain volume encompassed by the 10% to 100% isodose curves (V10%-V100%) in the two different plans (boost RTP1 and boost RTP2) were compared.
Paired sample t tests were used to test the significance of the change in surgical cavity volume, normal brain volume, and the irradiated volume of normal brain between the boost RTP1 and boost RTP2. Pearson's correlation test was used to investigate prognostic factors for volume change at the 0.05 significance level. The P values were from two-sided tests. The statistical package used in this analysis was SPSS software version 20.0 (SPSS Inc., Chicago, IL, USA).
The patient characteristics are given in Table 1. Steroids were used for the management of intracranial pressure in seven (36.8%) of 19 patients. Time from the day of surgery to that of obtaining CT1 and CT2 was 16 to 32 days (median, 21 days) and 48 to 66 days (median, 56 days), respectively. The total radiation dose was 60 Gy in all patients, with a 10 Gy of boost RT dose usually delivered after an initial 50 Gy dose.
The surgical defect volumes on CT0, GTV1, and GTV2 were 19.6-198.5 mL (median, 65.4 mL), 12.3-142.1 mL (median, 33.7 mL), and 7.9-96.3 mL (median, 25.1 mL), respectively. Fig. 3 shows an example of the changes in surgical defect after GTR (Fig. 3). The volume of the initial surgical cavity on CT0 was reduced by 6.48-56.40 mL (median, 21.86 mL) to GTV1 in all patients. The ratio of volumetric reduction was 14.4%-51.3% (median, 29.0%). The reduced volumes and the reduction ratios from GTV1 to GTV2 were -2.7~45.8 mL (median, 15.4 mL) and -7.9%-71.9% (median, 34.9%), respectively. The GTV was reduced in all but one patient. Overall, the surgical defect volumes were reduced from the surgical cavity on CT0 to GTV2 by 9.0%-79.7% (median, 56.1%). The differences between the surgical defect volumes on CT0, GTV1, and GTV2 were statistically significant (P < 0.001). The defect volume reduction ratio per day during the periods between the enforcement date of CT0 and sim-CT1, sim-CT1 and sim-CT2, and CT0 and sim-CT2 were 0.77%-2.70% (median 1.57%), -0.22%-1.99% (median 0.97%), and 0.16%-1.47% (median 0.99%), respectively.
The V10% to V100% of normal brain in boost RTP1 was significantly reduced in boost RTP2 (Table 2). The irradiated volume of normal brain decreased according to Vx%. The volume at boost RTP2 was significantly lower than the volume at boost RTP1 at all dose levels. As the dose level increased, the reduced volume decreased; however, the reduction ratio of the volume rose inversely. The dose-volume relationship of normal brain in the two-boost RTP in each patient is illustrated in Fig. 4. In five patients, a 95% isodose curve in boost RTP1 did not completely cover the CTV2, therefore the missed target volume developed in 26.3% of patients.
Glioblastoma is usually unifocal and can infiltrate surrounding tissue as an intra-parenchymal tumor. Therefore, the RT field should include the edema around the tumor or a constant margin extending from the tumor or surgical cavity (6-8). Radiologic images have been an important reference to the RT target volume, as there is a correlation between the GTV identified as tumor tissue on the histologic specimen and contrast enhancement on CT or MRI (9-14). Although there are two ways to determine the CTV, including the surrounding edema or not, neither method considers the volumetric changes in the RT target during periods of adjuvant RT, especially changes in the surgical cavity.
When the gross tumor is totally resected, the initial radiation target is the surgical cavity plus 2-3 cm a margin. For the boost plan, the GTV had been delineated based on the initial RT planning CT. However, during the period of RT, the volume of the surgical cavity can change and the radiation target may also be altered. Therefore, the boost plan could be implemented with newly obtained simulation CT scans based on the rationale that the surgical cavity may change during RT. The aim of the current study was to investigate the advantages of application of a second RT planning CT by analyzing the change in volume and the effects of volumetric change during RT.
In the current study, the surgical defect volumes were reduced from the surgical cavity on CT0 to GTV1 and from GTV1 to GTV2. However, the exact volume reduction ratios according to elapsed time from resection were not analyzed because the defect volumes were compared only on CT0, sim-CT1, and sim-CT2 imaging. More precisely and regularly implemented diagnostic images following resection would enable estimation of the ratios. In a study at the University of Wisconsin, a second (mid-treatment) MRI enhanced by the tumor-specific agent motexafin gadolinium (MGd) was obtained to define the boost volume (15). That study defined partial and complete geographic misses as escape of the GTV-mid from GTV-pre plus 1.0 cm and 2.0 cm, respectively, and found eight (53%) partial geographic misses and four (27%) complete geographic misses in the 15 MRI sets available for evaluation. These target volume mismatch data are difficult to compare directly to the results of the current study for two reasons. First, we produced hypothetical boost RT plan based on first simulation CT, and tried to find out whether altered target volume escape 95% isodose line of the hypothetical plan. Second, all enrolled patients underwent gross total resection in this study. Thus, more precise evaluation of surgical cavity change was possible. However, regarding the margin extension from GTV shown on the second planning image, the missed target volume defined in the present study could be substituted for the partial geometric miss of the Wisconsin study. Although all patients in the present study had GTR, a 26.3% of missed target volume ratio should be considered with caution, and a substantial number of patients may receive benefit from a volume adapted re-planning during RT.
In the present study, the volume of the tumor bed decreased continuously following resection, and the reduction ratio was higher in the pre-RT stage than during the RT stage. It seems that the degree of histologic alteration around the tumor bed may decrease in the earlier phase and become stable over time. This tumor bed volume reduction is likely the result of several factors including more serous fluid in the immediate postoperative period as a consequence of recent surgery, tissue contraction, and fibrosis with distortion as dynamic healing progresses. The irradiated volume of the normal brain decreased from boost RTP1 to boost RTP2 by 11.58% in V10% and 48.23% in V100%. Significantly, the ratio of reduction volume in higher dose levels may be more diminished than that in lower dose levels. Several studies have reported that the RT volume and dose in the treatment of brain malignancies are important factors for radiation-induced toxicity (16, 17). Lawrence et al (18). showed that, for standard fractionation RT to partial brain, a 5% and 10% risk of symptomatic radiation necrosis is predicted to occur at a biological equivalent dose of 120 Gy and 150 Gy with an α/β ratio of 3, respectively. It has also been documented that RT seems to induce neuropsychological impairment in patients with no clinical evidence of tumor recurrence on CT imaging (19-21). The volume of irradiated normal brain could be reduced by using volume-adapted re-planning for boost RT, particularly, an approximate 50% decrease in volume with high dose radiation. This volume reduction may contribute to reduced RT-induced necrosis and neuropsychological impairment.
There are some limitations to the current study. Even though volume-adapted re-planning likely provides benefits, the optimal time and frequency for RT re-planning is not clear and should be evaluated in future studies.
In summary, the volume of the surgical cavity was reduced following surgery and during RT in patients with glioblastoma who underwent GTR. The application of volume-adapted re-planning during RT may decrease the irradiated volume of normal brain and prevent a radiation target miss for boost RT.
Figures and Tables
Fig. 1
Treatment schedule and definitions of parameters: GTV1 and GTV2 were surgical defects on sim-CT1 and sim-CT2. Sim-CT, simulation CT; GTV, gross tumor volume; CTV, clinical target volume.
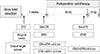
Fig. 2
The schematic presentations of each 95% isodose lines, GTV and CTV in sim-CT1 and sim-CT2; CTV2 may not be fully covered with 95% isodose line of boost RTP1 (C). RTP, radiation treatment planning.
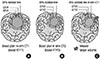
Fig. 3
The changes in surgical defect after surgery: The white circles show the changes of volumes of surgical defect. (A) CT image of one day after surgery. (B) CT image of first simulation for postoperative radiotherapy. (C) CT image of second simulation for boost radiotherapy.
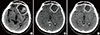
References
1. Seither RB, Jose B, Paris KJ, Lindberg RD, Spanos WJ. Results of irradiation in patients with high-grade gliomas evaluated by magnetic resonance imaging. Am J Clin Oncol. 1995; 18:297–299.
2. Shapiro WR, Green SB, Burger PC, Mahaley MS Jr, Selker RG, VanGilder JC, Robertson JT, Ransohoff J, Mealey J Jr, Strike TA, et al. Randomized trial of three chemotherapy regimens and two radiotherapy regimens and two radiotherapy regimens in postoperative treatment of malignant glioma: Brain Tumor Cooperative Group Trial 8001. J Neurosurg. 1989; 71:1–9.
3. Oppitz U, Maessen D, Zunterer H, Richter S, Flentje M. 3D-recurrence-patterns of glioblastomas after CT-planned postoperative irradiation. Radiother Oncol. 1999; 53:53–57.
4. Wallner KE, Galicich JH, Krol G, Arbit E, Malkin MG. Patterns of failure following treatment for glioblastoma multiforme and anaplastic astrocytoma. Int J Radiat Oncol Biol Phys. 1989; 16:1405–1409.
5. Nelson DF, Curran WJ Jr, Scott C, Nelson JS, Weinstein AS, Ahmad K, Constine LS, Murray K, Powlis WD, Mohiuddin M, et al. Hyperfractionated radiation therapy and bis-chlorethyl nitrosourea in the treatment of malignant glioma: possible advantage observed at 72.0 Gy in 1.2 Gy B.I.D. fractions: report of the Radiation Therapy Oncology Group Protocol 8302. Int J Radiat Oncol Biol Phys. 1993; 25:193–207.
6. Jansen EP, Dewit LG, van Herk M, Bartelink H. Target volumes in radiotherapy for high-grade malignant glioma of the brain. Radiother Oncol. 2000; 56:151–156.
7. Chang EL, Akyurek S, Avalos T, Rebueno N, Spicer C, Garcia J, Famiglietti R, Allen PK, Chao KS, Mahajan A, et al. Evaluation of peritumoral edema in the delineation of radiotherapy clinical target volumes for glioblastoma. Int J Radiat Oncol Biol Phys. 2007; 68:144–150.
8. Giraud P, Kantor G, Loiseau H, Rosenzweig KE. Target definition in the thorax and central nervous system. Semin Radiat Oncol. 2005; 15:146–156.
9. Halperin EC, Bentel G, Heinz ER, Burger PC. Radiation therapy treatment planning in supratentorial glioblastoma multiforme: an analysis based on post mortem topographic anatomy with CT correlations. Int J Radiat Oncol Biol Phys. 1989; 17:1347–1350.
10. Burger PC, Dubois PJ, Schold SC Jr, Smith KR Jr, Odom GL, Crafts DC, Giangaspero F. Computerized tomographic and pathologic studies of the untreated, quiescent, and recurrent glioblastoma multiforme. J Neurosurg. 1983; 58:159–169.
11. Johnson PC, Hunt SJ, Drayer BP. Human cerebral gliomas: correlation of postmortem MR imaging and neuropathologic findings. Radiology. 1989; 170:211–217.
12. Kelly PJ, Daumas-Duport C, Kispert DB, Kall BA, Scheithauer BW, Illig JJ. Imaging-based stereotaxic serial biopsies in untreated intracranial glial neoplasms. J Neurosurg. 1987; 66:865–874.
13. Park I, Tamai G, Lee MC, Chuang CF, Chang SM, Berger MS, Nelson SJ, Pirzkall A. Patterns of recurrence analysis in newly diagnosed glioblastoma multiforme after three-dimensional conformal radiation therapy with respect to pre-radiation therapy magnetic resonance spectroscopic findings. Int J Radiat Oncol Biol Phys. 2007; 69:381–389.
14. Datta NR, David R, Gupta RK, Lal P. Implications of contrast-enhanced CT-based and MRI-based target volume delineations in radiotherapy treatment planning for brain tumors. J Cancer Res Ther. 2008; 4:9–13.
15. Manon R, Hui S, Chinnaiyan P, Suh J, Chang E, Timmerman R, Phan S, Das R, Mehta M. The impact of mid-treatment MRI on defining boost volumes in the radiation treatment of glioblastoma multiforme. Technol Cancer Res Treat. 2004; 3:303–307.
16. Marks JE, Baglan RJ, Prassad SC, Blank WF. Cerebral radionecrosis: incidence and risk in relation to dose, time, fractionation and volume. Int J Radiat Oncol Biol Phys. 1981; 7:243–252.
17. Constine LS, Konski A, Ekholm S, McDonald S, Rubin P. Adverse effects of brain irradiation correlated with MR and CT imaging. Int J Radiat Oncol Biol Phys. 1988; 15:319–330.
18. Lawrence YR, Li XA, el Naqa I, Hahn CA, Marks LB, Merchant TE, Dicker AP. Radiation dose-volume effects in the brain. Int J Radiat Oncol Biol Phys. 2010; 76:S20–S27.
19. Maire JP, Coudin B, Guérin J, Caudry M. Neuropsychologic impairment in adults with brain tumors. Am J Clin Oncol. 1987; 10:156–162.
20. Hochberg FH, Slotnick B. Neuropsychologic impairment in astrocytoma survivors. Neurology. 1980; 30:172–177.
21. Merchant TE, Kiehna EN, Li C, Shukla H, Sengupta S, Xiong X, Gajjar A, Mulhern RK. Modeling radiation dosimetry to predict cognitive outcomes in pediatric patients with CNS embryonal tumors including medulloblastoma. Int J Radiat Oncol Biol Phys. 2006; 65:210–221.