Abstract
Transglutaminase 2 (TG2), a cross-linking enzyme, is involved in drug resistance and in the constitutive activation of nuclear factor kappa B (NF-κB). We investigated the association of non-small cell lung cancer (NSCLC) treatment efficacy with TG2 and NF-κB expression in 120 patients: 102 with adenocarcinoma and 18 with other histologic types. All patients underwent surgery; 88 received adjuvant chemotherapy, with 28 receiving platinum-based doublet chemotherapy as first-line treatment and 29 receiving epidermal growth factor receptor (EGFR)-tyrosine kinase inhibitor (TKI) therapy. Patients' TG2 and NF-κB expression values were calculated semiquantitatively. The median TG2 value was 50 (range, 0-300) and the median NF-κB value was 20 (range, 0-240). Disease-free survival did not differ between the low- and high-TG2 groups. Among patients who received palliative platinum-based doublet chemotherapy, progression free survival (PFS) was longer in the low-TG2 group than in the high-TG2 group (11.0 vs. 7.0 months; P=0.330). Among those who received EGFR-TKI therapy, PFS was also longer in the low-TG2 group than in the high-TG 2 group (11.0 vs. 2.0 months; P=0.013). Similarly, in EGFR wild-type patients treated with EGFR-TKI, PFS was longer in patients with low TG2 expression (9.0 vs. 2.0 months; P=0.013). TG2 expression levels can predict PFS in patients with NSCLC treated with EGFR-TKI.
Lung cancer is the most common cause of malignancy-related death in Korea. In 2011, an estimated 21 902 new cases of lung and bronchial cancer were diagnosed, in 15 496 men and 6406 women (1). Approximately 85% of lung malignancies are represented by non-small cell lung cancer (NSCLC). Recently, the presence of mutation in the epidermal growth factor receptor (EGFR) hasve been used as molecular predictive factors of treatment efficacy for EGFR-tyrosine kinase inhibitors (TKIs).
The nuclear factor kappa B (NF-κB) pathway is associated with lung cancer carcinogenesis, EGFR-TKI resistance, and cytotoxic chemotherapy resistance (2-7). The NF-κB family of transcription factors has 5 members in mammalian cells: RELA (also known as p65), REL (also known as c-REL), RELB, p50, and p52; these factors form either homodimers or heterodimers. In resting cells, NF-κB dimers are normally kept in an inactive state by their association with proteins of the inhibitor of κB (IκB) family.
Transglutaminases (TGs) are a family of calcium-dependent enzymes involved in the posttranslational modification of proteins. They catalyze cross-linking via ε-(γ-glutamyl) lysine isopeptide bonds or through incorporating primary amines at select glutamine residues. The 8 TGs that have been identified in mammals and humans all require proteolytic cleavage of propeptides, and 3 of these (TG2, TG3, and TG5) are inhibited by guanosine triphosphate. Tissue transglutaminase (TG2, also known as tTG) is the most diversely functioning and ubiquitous member of the TG family (8).
The interaction between TG2 and IκBα leads to constitutive activation of NF-κB and confers protection against stress-induced cell damage caused by reactive oxygen species, inflammatory cytokines, and chemotherapeutic drugs. This suggests a new paradigm for TG involvement in NF-κB activation that does not require kinase-ubiquitin-proteasome signaling for constitutive NF-κB activation. Interestingly, it has been reported that TG2 expression correlates with drug resistance (9,10). TG2 inhibition results in increased sensitivity to chemotherapeutic drugs; in other words, TG2 inhibition may represent a potentially useful treatment for certain cancers (11).
Methylation of the transglutaminase 2 gene (TGM2) promoter can predict patients' response to cisplatin treatment, and TG2 inhibition appears to be an effective cisplatin-sensitizing modality in patients with NSCLC (12). Furthermore, a higher level of TG2 expression is significantly correlated with recurrence and shorter disease-free survival (DFS) in patients with NSCLC.
The objectives of this study were 1) to immunohistochemically assess NF-κB and TG2 expression in NSCLC tumor samples; 2) to evaluate the relation between NF-κB and TG2 expression and the clinicopathological parameters of NSCLC; 3) to estimate the prognostic ability of these semiquantitative NF-κB and TG2 values for survival in patients with resected NSCLC tumors; and 4) to evaluate NF-κB and TG2 values as predictive factors of the efficacy of cytotoxic chemotherapy and EGFR-TKI treatment.
We retrospectively analyzed archived slides and medical records from the lung cancer registry of Yonsei University College of Medicine in Seoul, Korea. We selected 120 patients with resected lung cancer who were treated between 2004 and 2011. All patients had adenocarcinoma histology at diagnosis, EGFR mutation testing results available, and all underwent curative pulmonary resection. We excluded patients who died of a secondary malignancy during the study period. Clinical data were obtained from the medical records.
All study patients had paraffin-embedded tissue specimens available for immunohistochemical staining. The recipient blocks were made from purified agar in 3.8×2.2×0.5-cm frames. The paraffin donor blocks were prepared after a thorough evaluation of the hematoxylin and eosin-stained slides. Two adjacent areas of a carcinoma from matching donor blocks were transplanted to the recipient blocks using a 2 mm core needle. We also constructed an array of samples from adjacent normal tissue areas using paraffin-embedded formalin-fixed tissue blocks. Consecutive sections, with a thickness of 4 µm, were cut from the recipient blocks using an adhesive-coated slide system (Instrumedics Inc., Hackensack, NJ, USA). The 4 µm sections were placed on silane-coated slides, deparaffinized, immersed in phosphate-buffered saline (PBS) containing 0.3% (vol/vol) hydrogen peroxide, and then processed in a microwave oven (10 mmol/L sodium citrate buffer, pH 6.5, for 15 min at 700 W). After blocking with 1% (wt/wt) bovine serum albumin in PBS containing 0.05% (vol/vol) polysorbate 20 detergent solution for 30 min, the slides were incubated overnight at 4℃ with NF-κB p65 (Santa Cruz Biotechnology, Inc., Santa Cruz, CA, USA) and TG2 (NeoMarkers Inc., Fremont, CA, USA). The final reaction product was visualized with the addition of 0.03% (wt/vol) of 3,3'-diaminobenzidine tetrachloride for 5 to 20 min (13).
All immunostained tissue sections were evaluated and scored by a single investigator who was blinded to all clinical and pathological information. For each sample, 1,000 cells were assessed in 3 or 4 different fields at 400×magnification.
NF-κB expression was detected by brown cytoplasmic staining of varying intensity in the neoplastic cells. Positivity for NF-κB expression was defined as distinct cytoplasmic immunostaining. The staining intensity was scored on a scale of 0 to 3: 0, no staining of cancer cells; 1, weak staining; 2, moderate staining; 3, strong staining. The percentage of stained tumor cells was then scored from 0% to 100%, and these 2 scores were multiplied (14). NF-κB expression was defined as low if the result was less than the median value of all calculated products; expression was defined as high if the result was greater than the median value. Lymphocytes within the tissue sections were used as positive internal controls, as they consistently showed positive nuclear staining.
Immunostaining for TG2 was similarly graded semi-quantitatively, considering both the staining intensity and the percentage of positive tumor cells, by a single study pathologist blinded to the clinicopathologic variables. The staining intensity was scored on a scale of 0 to 3: 0, no staining of cancer cells; 1, weak staining; 2, moderate staining; 3, strong staining. The percentage of stained tumor cells was scored from 0% to 100%, and the 2 scores were multiplied (14). TG2 expression was defined as low if the result was less than the median value for TG2; TG2 expression was defined as high if the result was more than this median value. TG2 expression was found in cells of normal structure, specifically in smooth muscle cells and blood-vessel endothelial cells. However, there was no expression in alveolar- or bronchial epithelial cells.
DNA was extracted from 5 paraffin sections, each 10 µm thick and containing a representative portion of each tumor block, using the QIAamp DNA Mini Kit (Qiagen, Hilden, Germany) (15). DNA samples of 100 ng were amplified in a 20 µL reaction solution containing 2 µL of 10X buffer (Roche Diagnostics GmbH, Mannheim, Germany), 1.7 to 2.5 mM/L of magnesium chloride, 0.3 µM of each primer pair (exon 18, F:5'-tccaaatgagctggcaagtg, R:5'-ccacacag-caaagcagaaactcac; exon 19, F:5'-atgtggcaccatctcacaaattgcc, R: 5'-ccacacagcaaagcagaaactcac; exon 21, F: 5'-gctcagagcctggcatgaa, R: 5'-catcctcccctgcatgtgt; exon 23, F: 5'-tgaagcaaattgcccaagac, R: 5'-tgacatttctccagggatgc), 250 µM of deoxynucleoside triphosphate, and 2.5 units of DNA polymerase (Roche Diagnostics GmbH, Mannheim, Germany). Amplifications were performed using a 5 min initial denaturation at 94℃; followed by 30 cycles of 1 min at 94℃, 1 min at 55℃, and 1 min at 72℃; and a 10 min final extension at 72℃. Polymerase chain reaction (PCR) products were then purified with a 2% QIAgen gel extraction kit (Qiagen, Hilden, Germany). The templates were processed for the DNA sequencing reaction using ABI-PRISM BigDye Terminator, version 3.1 (Applied Biosystems, Inc., Foster City, CA, USA) with both forward- and reverse sequence-specific primers. A total of 20 ng of purified PCR product was used in a 20 µL sequencing reaction solution containing 8 µL of the terminator solution and 0.1 µM of the same PCR primer. Sequencing reactions were performed using a 2 min initial denaturation at 96℃; followed by 25 cycles of 10 sec at 94℃, 15 sec at 50℃, and 3 min at 60℃. Sequence data were generated using the ABI PRISM 3100 genetic analyzer (Applied Biosystems Inc., Foster City, CA, USA). Sequence variation was analyzed using Sequencer 3.1.1. software (Applied Biosystems Inc., Foster City, CA, USA).
Statistical analyses were performed using Statistical Package for the Social Sciences (SPSS) software, version 18.0 for Windows (SPSS Inc., Chicago, IL, USA). The association between categorical variables, such as NF-κB and TG2 expression values, was estimated by the χ2 test or Fisher's exact test. Survival distribution was estimated by the Kaplan-Meier method. Significant differences in the probability of patients experiencing relapse, progression, or death was evaluated by the log-rank test. Cox multiple regression analysis was used to assess the role of NF-κB, TG2, and other clinicopathological factors as prognostic and predictive factors, after adjusting for those variables that resulted in significant differences on univariate analysis.
DFS was determined from the date of surgery to the time of relapse. Overall survival (OS) was defined as the interval between the date of diagnosis to the date of death or the last follow-up visit. Progression-free survival (PFS) was defined as the interval between the start of palliative treatment (platinum-based doublet or EGFR-TKI) and the time of clinical progression, death, or the last follow-up visit if the disease had not progressed.
Patient characteristics at baseline are shown in Table 1. A total of 60 men and 60 women with NSCLC were included in this study, with ages ranging from 41 to 84 yr (median, 64 yr). Although all patients had a preoperative diagnosis of adenocarcinoma, final analysis revealed 102 patients (85%) with adenocarcinoma; the remainder demonstrated sarcomatoid carcinoma (n=1), squamous cell carcinoma (n=3), large cell carcinoma (n=5), mucoepidermoid carcinoma (n=1), giant cell carcinoma (n=3), and other histology (n=3). Of the 120 patients, 11 received neoadjuvant chemotherapy. Twenty-five (20.8%) were current smokers, 16 (13.3%) were former smokers, and 79 (65.8%) never smoked. The median calculated TG2 value was 50 (range, 0-300), and the median NF-κB value was 20 (range, 0-240). Both NF-κB and TG2 staining mainly accumulated in the cytoplasm (Fig. 1). High TG2 expression was more frequently detected in non-adenocarcinoma samples. TG2 expression was also related to EGFR-mutation status: more patients with wild-type EGFR showed high levels of TG2 expression (P=0.017) (Table 1). NF-κB expression did not differ according to clinicopathological parameters (data not shown).
A total of 76 patients relapsed and 50 patients died during the study period. The median DFS was 24.0 months (95% confidence interval [CI], 16.6-31.4), and there was no difference in DFS according to NF-κB and TG2 expression level (Fig. 2A, B). The median OS was not reached; the median follow-up duration was 38 months (range, 1.0-90.0 months).
Univariate analysis was performed for NF-κB and TG2 expression and for 9 clinicopathological factors: patient age, sex, the presence of weight loss, the Eastern Cooperative Oncology Group performance status, tumor histology, tumor stage, EGFR mutation status, and the use of adjuvant chemotherapy. We found that tumor stage, TG2 expression, age, weight loss, histology type, and EGFR mutation were statistically significant; we subsequently analyzed these in a multivariate model (Table 2). Lower tumor stage (hazard ratio [HR], 3.150; 95% CI, 1.830-5.423; P<0.001) was significantly associated with better DFS, whereas older age (HR, 1.743; 95% CI, 0.942-3.226; P=0.007), weight loss (HR, 3.732; 95% CI, 1.235-11.273; P=0.020), non-adenocarcinomatous histology (HR, 3.057; 95% CI, 1.469-6.361, P=0.003), and higher stage (HR, 4.093; 95% CI, 1.832-9.145; P=0.001) were independent factors associated with worse OS. EGFR mutation status was an independent factor associated with better OS (HR, 0.458; 95% CI, 0.236-0.888; P=0.021).
A total of 28 patients received platinum-based doublet chemotherapy as first-line treatment, and 29 patients received EGFR-TKI treatment (7 as first-line, 18 as second-line, 3 as third-line, and 1 as fourth-line). Of the 28 patients treated with platinum-based doublet chemotherapy, 25 patients experienced disease progression; their median PFS was 8.0 months (95% CI, 5.6-10.4 months). The median PFS for patients with low TG2 expression was longer than that of patients with high TG2 expression (11.0 vs 7.0 months; P=0.330) (Fig. 2C, D). No patients had a complete response to platinum-based doublet chemotherapy, 14.3% had a partial response, 57.1% had stable disease, and 21.4% had progressive disease; the overall response rate was 14.3%, and the disease-control rate was 71.4%.
Of the 29 patients treated with EGFR-TKI, 20 experienced disease progression, with a median PFS of 6.0 months (95% CI, 3.8-8.2 months). The median PFS for patients with low TG2 expression was significantly longer than that of patients with high TG2 expression (11.0 vs 2.0 months; P=0.013), however, EGFR mutation and NF-κB expression were not significant factors (Fig. 3A-C). A total of 3.4% of patients had a complete response to EGFR-TKI, 20.7% had a partial response, 34.5% had stable disease, 34.5% had progressive disease, and 6.0% were not evaluable; the overall response rate was 24.1%, and the disease-control rate was 58.6%.
As histology and TG2 expression levels were significant on univariate analysis, they were analyzed in a multivariate model (Table 3). In patients treated with platinum-based doublet chemotherapy, there were no significant predictive factors. PFS was longer in the low-TG2 group than in the high-TG2 group (11.0 vs 7.0 months; P=0.330), but this difference did not achieve statistical significance. In patients treated with EGFR-TKI, only high TG2 expression (HR, 2.637; 95% CI, 1.026-6.780; P=0.044) was associated with poor PFS. No factors were associated with the response rate or the disease-control rate (data not shown).
In patients who were positive for EGFR mutations and treated with EGFR-TKI, there was no difference in PFS according to NF-κB and TG2 expression levels (Fig. 3D, E). In EGFR wild-type patients treated with EGFR-TKI, there was no predictive factor for response- and disease-control rates, however, higher TG2 expression was a statistically significant prognostic factor for PFS on multivariate analysis (HR, 5.915; 95% CI, 1.157-30.241; P=0.033) (Fig. 3F, G). The PFS was 5.0 months (95% CI, 1.9-8.1 months), 8.0 months (95% CI, 4.1-11.9 months), 5.0 months, and 1.0 month in patients who received first-line, second-line, third-line, and fourth-line treatment, respectively. PFS was not different between the first-line and second-line groups (P=0.150). In the low-TG2 group, a total of 4, 11, 1, and 1 patients received EGFR-TKI as first-, second-, third-, and fourth-line treatment, respectively. In the high-TG2 group, a total of 3, 7, and 2 patients received EGFR-TKI as first-, second-, and third-line treatment, respectively. There was no difference in the percentage of patients with high TG2 expression between the first-line and second-line groups (42.9% vs 38.9%; P=1.000).
14 EGFR wild-type patients who received EGFR-TKI; 8 patients showed low TG2 expression and 6 patients had high TG2 expression. There was a significant difference in PFS between these groups (9.0 months vs 2.0 months; P=0.033; HR, 5.915; 95% CI, 1.157-30.241).
NSCLC is an aggressive disease characterized by rapid progression and very poor patient survival. The majority of patients show resistance to conventional first-line cytotoxic chemotherapy. High levels of TG2 produce constitutive NF-κB activation and are associated with drug resistance in patients with lung cancer (12). We found that the TG2 expression level represents the level of drug resistance, as represented by the PFS in patients with NSCLC treated with EGFR-TKI, especially in wild-type EGFR patients. Unfortunately, we were not able to show the prognostic role of NF-κB and TG2 values for DFS and OS.
Recently, a major phase III study compared EGFR-TKI treatment with cytotoxic chemotherapy and showed a superior response rate and better PFS for EGFR-TKI in EGFR mutation-positive patients. Several studies have shown that the EGFR-TKIs gefitinib and erlotinib have comparable efficacy and a favorable toxicity profile when used for pretreated NSCLC patients (16-22). In the present study, EGFR wild-type patients with low TG2 expression showed superior PFS.
We know that TG2 causes the constitutive activation of NF-κB, a primary resistance regulator, through IκB-α (23). TG2 is an epithelial-mesenchymal transition (EMT) inducer involved in drug resistance (24-27). EMT has been suggested as a mechanism of resistance to EGFR-TKI, regardless of EGFR-mutation status. Furthermore, loss of epithelial cadherin expression makes lung cancer more invasive and more advanced (28). This finding makes TG2 especially important in patients with wild-type EGFR. Recent data show that patients with advanced NSCLC, a wild-type EGFR status, and an epithelial phenotype benefit more from treatment with EGFR-TKIs, which indicates that EMT and TG2 may be used as a marker to guide individual EGFR-TKI therapy in this subpopulation (29). Thus, TG2 may be a pharmaceutical target for overcoming resistance to EGFR-TKI medications, and TG2 expression in patients with NSCLC may be a marker of EGFR-TKI efficacy. Future testing of a regimen comprising EGFR-TKIs and TG2 inhibitors, in several NSCLC cell lines and in a xenograft model, would help to realize the clinical implications of our findings.
TG2 shows potential as a prognostic factor in patients with NSCLS, with greater applicability than NF-κB, however statistical significance was not achieved in either DFS or OS. It is necessary to validate the prognostic role of TG2 and NF-κB in larger, prospective studies. In adenocarcinoma, unlike in squamous cell lung cancer, various kinds of driver mutations have been studied and various treatment options exist. In a previous study, TG2 was revealed to be a significant prognostic factor in squamous lung cancer (30). It is therefore necessary to study TG2 in the setting of squamous NSCLC.
In the present study, we found a longer PFS with first-line platinum-based chemotherapy than had been previously demonstrated (31); this could be because more patients in our study had adenocarcinoma histology, were male, and had EGFR mutations. Previous studies describe 85% of NSCLC patients as having adenocarcinoma (31), and 50% of patients as male (31, 32). Reportedly, 46% of patients have EGFR mutations.
EGFR mutations are known to be a strong predictive factor of EGFR-TKI efficacy; however, we found the opposite. In our study, EGFR mutation status was not a significantly predictive. This discrepancy is likely due to our small sample size, leading to a lack of ability to detect a significant difference in PFS.
Our study has several limitations. First, NF-κB is an inducible transcription factor, and immunohistochemistry cannot accurately represent the status of cell apoptosis. Second, our sample size is too small to show the association between drug resistance and the expression of TG2 and NF-κB. Third, EMT markers are not evaluated in this study. Fourth, with the use of surgical samples for immunohistochemistry, the specimens cannot represent the accurate NF-κB and TG2 status immediately before the start of treatment. Finally, immunohistochemistry scoring was performed by a single pathologist, possibly leading to bias.
In conclusion, it appears that in patients with NSCLC, the TG2 expression level is a promising predictive indicator of the efficacy of EGFR-TKI therapy. Larger clinical studies, as well as in vitro and in vivo studies, are needed to further investigate the role of TG2 in NSCLC.
Figures and Tables
![]() | Fig. 1Immunohistochemical staining for nuclear factor kappa B (NF-κB) subunit p65 (A) and transglutaminase 2 (TG2) (C) in patients with primary lung cancer. Cytoplasmic staining of varying intensity is evident in the cancer cells. Immunohistochemical-negative staining for NF-κB subunit p65 (B) and TG2 (D) in patients with primary lung cancer. |
![]() | Fig. 2Kaplan-Meier plot showing disease-free survival according to NF-κB level (A) and TG2 level (B). Progression-free survival (PFS) in patients with platinum-based doublet chemotherapy is shown according to NF-κB level (C) and TG2 level (D). |
![]() | Fig. 3Kaplan-Meier plot showing PFS in patients with epidermal growth factor receptor (EGFR)-tyrosine kinase inhibitor (TKI) therapy according to EGFR mutation status, NF-κB level, and TG2 level (A, B, C, respectively). Kaplan-Meier plot showing PFS in patients with EGFR-TKI therapy according to NF-κB level and TG2 level in patients positive for the EGFR mutation (D, E, respectively) and EGFR wild-type patients (F, G, respectively). NA, not available. |
Table 2
Univariate and multivariate analysis of prognostic factors for disease-free survival and overall survival
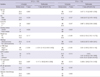
Table 3
Univariate and multivariate analysis of prognostic factors for progression-free survival
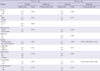
*PFS1, progression-free survival in patients treated with palliative platinum-based doublet chemotherapy; †PFS2, progression-free survival in patients treated with EGFR-TKI. PFS, progression-free survival; ECGO PS, Eastern Cooperative Oncology Group performance status; p-TNM, pathologic tumor/node/metastasis stage; EGFR, epidermal growth factor receptor; TG2, transglutaminase 2; NF-κB, nuclear factor kappa B.
Notes
References
1. Jung KW, Park S, Kong HJ, Won YJ, Lee JY, Park EC, Lee JS. Cancer statistics in Korea: incidence, mortality, survival, and prevalence in 2008. Cancer Res Treat. 2011; 43:1–11.
2. Arlt A, Gehrz A, Müerköster S, Vorndamm J, Kruse ML, Fölsch UR, Schäfer H. Role of NF-kappaB and Akt/PI3K in the resistance of pancreatic carcinoma cell lines against gemcitabine-induced cell death. Oncogene. 2003; 22:3243–3251.
3. Denlinger CE, Keller MD, Mayo MW, Broad RM, Jones DR. Combined proteasome and histone deacetylase inhibition in non-small cell lung cancer. J Thorac Cardiovasc Surg. 2004; 127:1078–1086.
4. Mabuchi S, Ohmichi M, Nishio Y, Hayasaka T, Kimura A, Ohta T, Saito M, Kawagoe J, Takahashi K, Yada-Hashimoto N, et al. Inhibition of NF-kappaB increases the efficacy of cisplatin in in vitro and in vivo ovarian cancer models. J Biol Chem. 2004; 279:23477–23485.
5. Motwani M, Delohery TM, Schwartz GK. Sequential dependent enhancement of caspase activation and apoptosis by flavopiridol on paclitaxel-treated human gastric and breast cancer cells. Clin Cancer Res. 1999; 5:1876–1883.
6. Patel NM, Nozaki S, Shortle NH, Bhat-Nakshatri P, Newton TR, Rice S, Gelfanov V, Boswell SH, Goulet RJ Jr, Sledge GW Jr, et al. Paclitaxel sensitivity of breast cancer cells with constitutively active NF-kappaB is enhanced by IkappaBalpha super-repressor and parthenolide. Oncogene. 2000; 19:4159–4169.
7. Sánchez-Pérez I, Benitah SA, Martínez-Gomariz M, Lacal JC, Perona R. Cell stress and MEKK1-mediated c-Jun activation modulate NFkappaB activity and cell viability. Mol Biol Cell. 2002; 13:2933–2945.
8. Mehta K, Kumar A, Kim HI. Transglutaminase 2: a multi-tasking protein in the complex circuitry of inflammation and cancer. Biochem Pharmacol. 2010; 80:1921–1929.
9. Antonyak MA, Miller AM, Jansen JM, Boehm JE, Balkman CE, Wakshlag JJ, Page RL, Cerione RA. Augmentation of tissue transglutaminase expression and activation by epidermal growth factor inhibit doxorubicin-induced apoptosis in human breast cancer cells. J Biol Chem. 2004; 279:41461–41467.
10. Han JA, Park SC. Hydrogen peroxide mediates doxorubicin-induced transglutaminase 2 expression in PC-14 human lung cancer cell line. Exp Mol Med. 1999; 31:83–88.
11. Kim DS, Park SS, Nam BH, Kim IH, Kim SY. Reversal of drug resistance in breast cancer cells by transglutaminase 2 inhibition and nuclear factor-kappaB inactivation. Cancer Res. 2006; 66:10936–10943.
12. Park KS, Kim HK, Lee JH, Choi YB, Park SY, Yang SH, Kim SY, Hong KM. Transglutaminase 2 as a cisplatin resistance marker in non-small cell lung cancer. J Cancer Res Clin Oncol. 2010; 136:493–502.
13. Shim HS, Yoon BS, Cho NH. Prognostic significance of paired epithelial cell adhesion molecule and E-cadherin in ovarian serous carcinoma. Hum Pathol. 2009; 40:693–698.
14. Pirker R, Pereira JR, von Pawel J, Krzakowski M, Ramlau R, Park K, de Marinis F, Eberhardt WE, Paz-Ares L, Störkel S, et al. EGFR expression as a predictor of survival for first-line chemotherapy plus cetuximab in patients with advanced non-small-cell lung cancer: analysis of data from the phase 3 FLEX Study. Lancet Oncol. 2012; 13:33–42.
15. Han SW, Kim TY, Hwang PG, Jeong S, Kim J, Choi IS, Oh DY, Kim JH, Kim DW, Chung DH, et al. Predictive and prognostic impact of epidermal growth factor receptor mutation in non-small-cell lung cancer patients treated with gefitinib. J Clin Oncol. 2005; 23:2493–2501.
16. Ciuleanu T, Stelmakh L, Cicenas S, Miliauskas S, Grigorescu AC, Hillenbach C, Johannsdottir HK, Klughammer B, Gonzalez EE. Efficacy and safety of erlotinib versus chemotherapy in second-line treatment of patients with advanced, non-small-cell lung cancer with poor prognosis (TITAN): a randomised multicentre, open-label, phase 3 study. Lancet Oncol. 2012; 13:300–308.
17. Cufer T, Vrdoljak E, Gaafar R, Erensoy I, Pemberton K. SIGN Study Group. Phase II, open-label, randomized study (SIGN) of single-agent gefitinib (IRESSA) or docetaxel as second-line therapy in patients with advanced (stage IIIb or IV) non-small-cell lung cancer. Anticancer Drugs. 2006; 17:401–409.
18. Karampeazis A, Voutsina A, Souglakos J, Kentepozidis N, Giassas S, Christofillakis C, Kotsakis A, Papakotoulas P, Rapti A, Agelidou M, et al. Pemetrexed versus erlotinib in pretreated patients with advanced non-small cell lung cancer: a Hellenic Oncology Research Group (HORG) randomized phase 3 study. Cancer. 2013; doi: 10.1002/cncr.28132.
19. Kim ES, Hirsh V, Mok T, Socinski MA, Gervais R, Wu YL, Li LY, Watkins CL, Sellers MV, Lowe ES, et al. Gefitinib versus docetaxel in previously treated non-small-cell lung cancer (INTEREST): a randomised phase III trial. Lancet. 2008; 372:1809–1818.
20. Lee DH, Park K, Kim JH, Lee JS, Shin SW, Kang JH, Ahn MJ, Ahn JS, Suh C, Kim SW. Randomized Phase III trial of gefitinib versus docetaxel in non-small cell lung cancer patients who have previously received platinum-based chemotherapy. Clin Cancer Res. 2010; 16:1307–1314.
21. Maruyama R, Nishiwaki Y, Tamura T, Yamamoto N, Tsuboi M, Nakagawa K, Shinkai T, Negoro S, Imamura F, Eguchi K, et al. Phase III study, V-15-32, of gefitinib versus docetaxel in previously treated Japanese patients with non-small-cell lung cancer. J Clin Oncol. 2008; 26:4244–4252.
22. Sun J, Lee KH, Kim S, Lee DH, Min YJ, Yun HJ, Kim HK, Song HS, Kim YH, Kim B, et al. Gefitinib versus pemetrexed as second-line treatment in patients with nonsmall cell lung cancer previously treated with platinum-based chemotherapy (KCSG-LU08-01). Cancer. 2012; 118:6234–6242.
23. Bivona TG, Hieronymus H, Parker J, Chang K, Taron M, Rosell R, Moonsamy P, Dahlman K, Miller VA, Costa C, et al. FAS and NF-[kgr]B signalling modulate dependence of lung cancers on mutant EGFR. Nature. 2011; 471:523–526.
24. Cao L, Shao M, Schilder J, Guise T, Mohammad KS, Matei D. Tissue transglutaminase links TGF-[beta], epithelial to mesenchymal transition and a stem cell phenotype in ovarian cancer. Oncogene. 2012; 31:2521–2534.
25. Kumar A, Xu J, Sung B, Kumar S, Yu D, Aggarwal B, Mehta K. Evidence that GTP-binding domain but not catalytic domain of transglutaminase 2 is essential for epithelial-to-mesenchymal transition in mammary epithelial cells. Breast Cancer Res. 2012; 14:R4.
26. Lin CY, Tsai PH, Kandaswami CC, Chang GD, Cheng CH, Huang CJ, Lee PP, Hwang JJ, Lee MT. Role of tissue transglutaminase 2 in the acquisition of a mesenchymal-like phenotype in highly invasive A431 tumor cells. Mol Cancer. 2011; 10:87.
27. Kumar A, Xu J, Brady S, Gao H, Yu D, Reuben J, Mehta K. Tissue transglutaminase promotes drug resistance and invasion by inducing mesenchymal transition in mammary epithelial cells. PLoS One. 2010; 5:e13390.
28. Lim SC, Jang IG, Kim YC, Park KO. The role of E-cadherin expression in non-small cell lung cancer. J Korean Med Sci. 2000; 15:501–506.
29. Ren S, Su C, Chen X, Li J, Li B, Li X, Zhou C. Association of epithelial to mesenchymal transition with efficacy of EGFR-TKIs in non-small cell lung cancer patients with EGFR wild type. In : ASCO Annual Meeting Abstracts; 2012. 30:p. 7535.
30. Choi C, Jang S, Park S, Choi Y, Jeong J, Kim D, Kim H, Park K, Nam B, Kim H, et al. Transglutaminase 2 as an independent prognostic marker for survival of patients with non-adenocarcinoma subtype of non-small cell lung cancer. Mol Cancer. 2011; 10:119.
31. Schiller JH, Harrington D, Belani CP, Langer C, Sandler A, Krook J, Zhu J, Johnson DH. Comparison of four chemotherapy regimens for advanced non-small-cell lung cancer. N Engl J Med. 2002; 346:92–98.
32. Ohe Y, Ohashi Y, Kubota K, Tamura T, Nakagawa K, Negoro S, Nishiwaki Y, Saijo N, Ariyoshi Y, Fukuoka M. Randomized phase III study of cisplatin plus irinotecan versus carboplatin plus paclitaxel, cisplatin plus gemcitabine, and cisplatin plus vinorelbine for advanced non-small-cell lung cancer: Four-Arm Cooperative Study in Japan. Ann Oncol. 2007; 18:317–323.