Abstract
This study focused on the characterization of mesenchymal stromal cells (MSCs) from the chorion of human full term placenta from 15 donors. Chorionic MSCs revealed homologous fibroblast-like morphology and expressed CD73, CD29, CD105, and CD90. The hematopoietic stem cell markers including HLA DR, CD11b, CD34, CD79a, and CD45 were not expressed. The growth kinetics of their serial passage was steady at the later passages (passage 10). The multilineage capability of chorionic MSCs was demonstrated by successful adipogenic, osteogenic and chondrogenic differentiation and associated gene expression. Chorionic MSCs expressed genes associated with undifferentiated cells (NANOG, OCT4, REX1) and cardiogenic or neurogenic markers such as SOX2, FGF4, NES, MAP2, and NF. TERT was negative in all the samples. These findings suggest that chorionic MSCs undifferentiated stem cells and less likely to be transformed into cancer cells. A low HLA DR expression suggests that chorionic MSCs may serve as a great source of stem cells for transplantation because of their immune-privileged status and their immunosuppressive effect. Based on these unique properties, it is concluded that chorionic MSCs are pluripotent stem cells that are probably less differentiated than BM-MSCs, and they have considerable potential for use in cell-based therapies.
Adult stem cells are considered as an excellent source for research because they pose few ethical problems and limitations in terms of availability. Adult stem cells have been isolated from a variety of sources including bone marrow (BM), fat tissue, umbilical cord and different parts of the placenta. Although many types of stem cells are available, the sources of the stem cells selected for clinical use should possess the ability to renew, be easily isolated and be pluripotent. These properties can be satisfied with human term placental-derived stromal cells. It is essential to understand the expansion capability and potency of isolated stem cells. These stromal cells are available in abundance and they do not involve partum: because they are easy to procure, there is a large supply available and they are exempt from ethical debate (1).
This study focused on the characterization of mesenchymal stromal cells (MSCs) from the chorion. We characterized the surface markers and multilineage differentiation (adipogenic, osteogenic and chondrogenic induction) ability of cultured chorionic MSCs. We also present our findings regarding their growth rate and gene expressions.
Human placenta (n = 15) was collected following delivery at the Seoul St. Mary's Hospital. The chorion was separated from the amnion by peeling them apart. Because chorionic MSCs were derived from the reticular layer of the chorion, the decidual tissue was scrapped mechanically and washed in Dulbecco's phosphate-buffered saline (DPBS; Gibco, Grand Island, NY, USA) before being cut into small pieces (-2 × 2 cm). The chorion were chopped into small pieces and subjected in 0.5% trypsin-EDTA for 5 min, followed by digestion with 0.3% collagenase I (Gibco) in 37℃ incubator for 20 to 30 min. The mobilized cells were then collected and passed through a 100 µm cell strainer (BD Falcon, Bedford, MA, USA). The filtered cells were collected by centrifugation at 2,500 rpm for 5 min (Fig. 1). The cells were resuspended in α-modified minimum essential medium (α-MEM; Gibco) supplemented with 10% fetal bovine serum (FBS; Hyclone, South Logan, Utah, USA) and 1% penicillin-streptomycin (Gibco), and cultured in T25 flasks (BD Falcon) at 37℃ and 5% CO2. The media was changed every 3 days until the chorionic MSCs reached 70% confluency.
At 70% subconfluency, the cells were trypsinized and counted using an automated hematology analyzer (Coulter AC·T diff, Beckman Coulter, South Kraemer Boulevard, CA, USA). The proliferation potential was estimated using the number of cells at each passage. The time required to reach subconfluency at each passage was also analyzed.
Flow cytometry was performed on chrionic MSCs at 3rd passage. To evaluate the cell-surface marker expression, cell suspensions of 1 × 106 were incubated for 15 min at room temperature in the dark with fluorescein isochiocyanate- or phycoerythrin-conjugated monoclonal antibodies specific for the human markers associated with mesenchymal and hematopoietic lineages. The employed antibodies were CD73, CD90, CD105, HLA DR, CD11b, CD34, CD79a, and CD45 (BD Biosciences, San Jose, CA, USA). The samples were fixed using fixation buffer (BD Biosciences) and analyzed on a FACS Aria cytometer and the resulting data was processed using CellQuest software (BD Biosciences).
Differentiation of each sample was performed according as follows; the cells were harvested at 3rd passage by trypsinization and seeded in 6-well culture dishes (BD Biosciences) as a concentration of 1 × 105/well. After expanding MSCs in growth medium to 70% confluency, media were changed into differentiation induction media. Cells were incubated at 37℃ for 3 weeks, and the fresh medium was replaced every 3 days. To evaluate adipogenic differentiation, the cells were cultured in adipogenesis differentiation basal medium (Gibco) containing adipogensis supplement such as 1 µM dexamethasone, 0.5 mM isobutylmethylxanthine and 200 µM indomethacin. The cytoplasmic inclusions of neutral lipids were then stained with oil red O (Sigma-Aldrich, St. Louis, MO, USA). Cells were fixed with 4% paraformaldehyde (PFA) solution and incubated in 60% isopropanol for 5 min at room temperature and then aspirate. Cells were stained with oil red O (Sigma) solution for 30-60 min.
Chondrogenic differentiation was assessed in a monolayer culture by incubating cells for 2-3 weeks in chondrogenesis differentiation basal medium (Gibco) containing chondrogensis supplement such as 10 ng/mL TGF-β1 and 50 nM ascorbic acid-2-phosphate. The presence of metachromatic matrix was visualized by alcian blue (Sigma-Aldrich) staining. Cells were fixed with 4% PFA solution and incubated in 1% alcian blue solution for 60 min at room temperature. After rinse, nuclei were stained using nuclear fast red (Sigma) for 5 min.
Osteogenic differentiation was assessed by incubating the cells in osteogenesis differentiation medium (Gibco) containing osteogenesis supplement such as 100 nM dexamethasone, 50 µM ascorbic acid-2-phosphate and 10 ng/mL recombinant human bone morphogenetic protein. The osteoblasts were identified by visualizing calcium deposits using the von Kossa method for calcium kit (Polysciences, Warrington, PA, USA). Cells were fixed with 4% PFA solution and incubated in 3% silver nitrate solution exposed UV light for 1 hr. After rinse, cells were incubated in 5% sodium thiosulfate for 2 min.
The total RNA was extracted from chorionic MSCs in 2nd (n = 6) and 3rd (n = 7) passage using a High Pure RNA Isolation Kit (Roche Applied Science, Mannheim, Germany) according to the manufacturer's recommendations. The stemness nature was visualized by detection of octamer-binding protein 4 (OCT4), SRY-related HMG-box 2 (SOX2), NANOG and reduced expression 1 (REX1). The extra-embryonic endoderm marker of GATA4 and chromatin modification and remodeling marker of telomerase reverse transcriptase (TERT) were analyzed. Fibroblast growth factor 4 (FGF4), HLA ABC, HLA DR and the markers associated with neurogenesis were also evaluated, including microtubule-associated protein 2 (MAP2), neurofilament (NF) and nestin (NES).
Cell differentiations were also assessed for 21 days by performing RT-qPCR on the extracted mRNAs. Adipogenic differentiation, chondrogenic differentiation and osteogenic differentiation were detected by the expression of lipoprotein lipase (LPL) and adiponectin (ADIPOQ), SOX9 and osteopontin (OPN), respectively. The relative mRNA expression from RT-qPCR experiments were estimated as the value 2-ΔΔCt using glyceraldehyde 3-phosphate dehydrogenase (GAPDH) as an internal control (2).
Human term placentas were obtained after receiving maternal informed consent. The experiment protocol was approved by the institutional review board of the Catholic Medical Center (IRB No. KC09WZZZ0173). All of the procedures followed the guidelines of the board. We received informed consents from all of the participants.
Chorionic MSCs presented with a fibroblast-like morphology very similar to that of BM-MSCs after 3 days in culture. Attached colonies are detected 7 days after seeding. It takes an average of 12 days to subculture the colonies first. The next subcultures were usually performed after 5 days. The homologous population of MSCs continued to proliferate which characterized by a small cell body with a few cell processes that are long and thin. The cell population curve demonstrated that chorionic MSCs maintain a continuous proliferation potential up to 10 passages (Fig. 2). The proliferation capacity was highest at 6 to 7 passages with an average of 35 days after seeding (Fig. 3).
Chorion MSCs express multiple markers of MSCs. The cultured chorionic MSCs revealed positivity for the MSC markers CD73, CD90, and CD105 and they were negative for the hematopoietic stem cell markers HLA DR, CD11b, CD34, CD79a, and CD45 (Fig. 4).
Our study showed that chorionic MSCs possess the potential to differentiate into adipocytes, chondroblasts and osteoblasts. The cells differentiating towards adipocytes accumulated lipid-rich vacuoles in the cytoplasm and these vacuoles stained red with Oil Red O (Fig. 5A). The control cultures retained their morphology and did not show lipid accumulation. The ability to undergo chondrogenic differentiation was shown by alcian blue staining, which demonstrated cartilage-specific metachromasia after 3 weeks of monolayer culture with chondrogenic induction medium, and this was not observed when the cells were cultured in control medium (Fig. 5B). Matrix mineralization was observed after 3 weeks of culture in osteogenic induction medium, and this was characterized by the deposition of calcium deposits and these were colored brown-black with silver nitrate solution (Fig. 5C). After osteogenic, chondrogenic and adipogenic differentiation, the mRNA expression levels of the lineage-specific markers osteopontin, SOX9 and LPL and adiponectin were increased more than those of the control (Fig. 5D).
The human chorionic MSCs isolated in the present study expressed pluripotency markers such as OCT4, SOX2, and NANOG. GATA4, which are associated with embryogenesis and myocardial differentiation, and neurogenesis markers, which included MAP2, NF, and NES, were also expressed in all the samples. REX1 was expressed in all of the 2nd passage samples and in 4 samples of the seven 3rd passage samples. TERT was not expressed in any of the samples. HLA ABC was expressed in all the samples. HLA DR was weakly expressed in four samples among the six 2nd passage samples and it was not expressed in any of the 3rd passage samples (Fig. 6).
The fetal membranes of the human placenta contain stromal/mesenchymal tissue at the contact point between the trophoblastic region of the chorion and the amniotic epithelial layer. The chorion is the membrane attached to the amnion by a spongy layer of loosely arranged collagen fibers. It consists of the mesodermal and trophoblastic regions and it is easily separated from the amnion. To date, amniotic MSCs have been widely characterized and discussed, but there are few research reports on chorionic MSCs (3-5). A few reports have disclosed the multipotential and expansion capability of the stem cells isolated from this source (6, 7). The minimal criteria for defining MSCs from placenta are as follows: adherence to plastic, formation of fibroblast colony-forming units, a specific pattern of the surface antigen expressions, the differentiation potential toward one or more lineages, including the osteogenic, adipogenic, chondrogenic and vascular/endothelia lineages, and a fetal origin (1).
In the present study, we have demonstrated that chorionic MSCs from human full term placenta possess immune phenotypes and gene expression profiles that are largely characteristic of undifferentiated cells. We observed that chorionic MSCs showed spindle shaped fibroblastic features that have been described as stem cell morphology. MSCs expressed surface markers, including CD73, CD29, CD105, and CD90, and this is profile similar to that of BM MSCs. The growth kinetics of their serial passage in the present study was steady at the later passages. A low HLA DR expression, as seen in the results, suggests that chorionic MSCs may serve as a great source of stem cells for transplantation because of their immune-privileged status and their immunosuppressive effect (6-8). The multilineage capability of human chorionic MSCs was demonstrated by successful adipogenic, osteogenic and chondrogenic differentiation. These findings were further supported by the expression of lineage-specific genes.
The qualitative mRNA expression profile revealed the chorionic MSCs expressed genes associated with undifferentiated cells (NANOG, OCT4, REX1). Some of these genes are known to be expressed in embryonic stem cells, but they are not commonly expressed in BM MSCs (OCT4, REX1). Our findings that these markers of undifferentiated cells are also expressed in chorionic MSCs leads us to conclude that chorionic MSCs may be less differentiated than most BM MSCs, and chorionic MSCs may more closely resemble pluripotent ESCs (9). It has been reported that the different mRNAs of those genes in chorionic MSCs are deceased after serial passage (6). GATA4, which is not expressed in BM MSCs, is a critical regulator of the cardiac gene expression, it controls embryonic development and cardiomyocyte differentiation and it confers a stress responsiveness in the adult heart (10, 11). Chorionic MSCs also expressed high levels of neurogenic markers (12). SOX2, FGF4, and NES are known to be pluripotent and neural markers (13, 14). Kosaka et al. (14) reported that SOX2 regulates the FGF4 expression, which in turn promotes neural stem cell proliferation and neural differentiation in the postnatal brain. NES is a specific marker for neural stem cells, and it is also found in other developing cells, including muscle and myocardial cells and MAP2 and NF are used as markers for mature neurons (15). The neurogenic potential of chorionic MSCs was almost 2-fold higher compared with that of BM MSCs, amnion epithelial cells or amniotic MSCs (16). Thus, the spontaneous expression of all of these genes suggests that chorionic MSCs have the potential to readily differentiate into cardiomyocytes as well as other mesodermal derivatives, in addition to neural cells. It is interesting that HLA-DR mRNA was expressed in the majority of the 2nd passage chorionic MSCs, but not in the 3rd passage chorionic MSCs. One possible explanation for this phenomenon may have been that if the chorionic MSCs are passaged several times in vitro, then the expression of HLA-DR is diminished, as was observed in amniotic MSCs (5). Otherwise, some cultures contaminated with trophoblasts were negatively selected through the 2nd to 3rd passages (6). Alternatively, the difference may be considered as being due to the sensitivities of the methods used, that is, flow cytometry versus RT-PCR. It will be helpful to confirm those differences using several methods, including RT-qPCR. Therefore, chorionic MSCs may be more pluripotent and they can be effectively used rather than BM MSCs for the adequate indications. Telomerase is known to maintain the telomere length, and it has been indicated to play a role in the self-renewal and pluripotency of ESCs (16). A telomerase expression was found in the MSCs of the embryo and the early fetal developmental stage in the preterm human placenta, but not during the late fetal and adult stages of development (17). TERT was negative in all the samples of that study. These findings suggest that chorionic MSCs are less likely to be transformed into cancer cells (6). Hence, chorionic MSCs could be a safe cell source for cell therapy.
The results of the experiments described in this report demonstrate that chorionic MSCs can differentiate into adipocytes, osteocytes and chondrocytes. They can express many pluripotent stem cell-specific genes and proliferate well during the ex vivo expansion. Based on these unique properties, it is concluded that chorionic MSCs are pluripotent stem cells that are probably less differentiated than BM-MSCs, and they have considerable potential for use in cell-based therapies.
Figures and Tables
Fig. 1
Cell isolation from chorion of the human placenta. Transport container and placenta containing chorion and amnion (A, B). Chorion isolated from the amnion by peeling (C). After removal of decidual tissue (D) and chopped into small pieces (E). Morphology of the cells after enzyme digestion (F) (× 100).
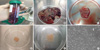
Fig. 2
Morphology of chorionic mesenchymal stromal cells in passage 0, 1, 2, 3, 5, 7, 9, and 10. (A-H) (× 40).

Fig. 4
Representative flow cytometry histogram of chorionic mesenchymal stromal cells. Colored histogram: isotype control, open histogram: signal for each specific antibody.
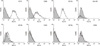
Fig. 5
Oil Red O staining of chroionic mesenchymal stromal cells (MSCs) after adipogenic induction (A). Alcian blue staining after chrondrogenic induction (B). Silver nitrate staining after osteogenic induction (C) (× 40). Expression of LPL, ADIPOQ, SOX9, and OPN (D). For this analysis, gene expression in chorionic MSCs before differentiation induction was set to 1.
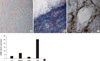
References
1. Parolini O, Alviano F, Bagnara GP, Gilic G, Bühring HJ, Evangerlista M, Hennerbichler S, Liu B, Magatti M, Mao N, et al. Concise review: isolation and characterization of cells from human term placenta: outcome of the first international workshop on placenta derived stem cells. Stem Cells. 2008. 26:300–311.
2. Livak KJ, Schmittgen TD. Analysis of relative gene expression data using real-time quantitative PCR and the 22DDCT method. Methods. 2001. 25:402–408.
3. Miki T, Lehmann T, Cai H, Stolz DB, Strom SC. Stem cell characteristics of amniotic epithelial cells. Stem Cells. 2005. 23:1549–1559.
4. Soncini M, Vertua E, Gibelli L, Zorzi F, Denegri M, Albertini A, Wengler GS, Parolini O. Isolation and characterization of mesenchymal cells from human fetal membrane. J Tissue Eng Regen Med. 2007. 1:296–305.
5. Kim J, Kang HM, Kim H, Kim MR, Kwon HC, Gye MC, Kang SG, Yang HS, You J. Ex vivo characteristic of human amniotic membrane-derived stem cells. Cloning Stem Cells. 2007. 9:581–594.
6. Fariha MM, Chua KH, Tan GC, Tan AE, Hayati AR. Human chorion-derived stem cells: changes in stem cell properties during serial passage. Cytotherapy. 2011. 13:582–593.
7. Ilancheran S, Moodley Y, Manuelpillai U. Human fetal membranes: a source of stem cells for tissue regeneration and repair? Placenta. 2009. 30:2–10.
8. Le Blanc K. Immunomodulatory effects of fetal and adult mesenchymal stem cells. Cytotherapy. 2003. 5:485–489.
9. Kim J, Lee Y, Hwang KJ, Kwon HC, Kim SK, Cho DJ, Kang SG, You J. Human amniotic fluid-derived stem cells have characteristics of multipotent stem cells. Cell Prolif. 2007. 40:75–90.
10. Kuo CT, Morrisey EE, Anandappa R, Sigrist K, Lu MM, Parmacek MS, Soudais C, Leiden JM. GATA4 transcription factor is required for ventral morphogenesis and heart tube formation. Genes Dev. 1997. 11:1048–1060.
11. Molkentin JD, Lin Q, Duncan SA, Olson EN. Requirement of the transcription factor GATA4 for heart tube formation and ventral morphogenesis. Genes Dev. 1997. 11:1061–1072.
12. Portmann-Lanz CB, Schoeberlein A, Huber A, Sager R, Malek A, Holzgreve W, Surbek DV. Placental mesenchymal stem cells as potential autologous graft for pre- and perinatal neuroregeneration. Am J Obstet Gynecol. 2006. 194:664–673.
13. Lensch MW, Daheron L, Schlaeger TM. Pluripotent stem cells and their niches. Stem Cell Rev. 2006. 2:185–201.
14. Kosaka N, Kodama M, Sasaki H, Yamamoto Y, Takeshita F, Takahama Y, Sakamoto H, Kato T, Terada M, Ochiya T. FGF-4 regulates neural progenitor cell proliferation and neuronal differentiation. FASEB J. 2006. 20:E623–E629.
15. Sjöberg G, Jiang WQ, Ringertz NR, Lendahl U, Sejersen T. Colocalization of nestin and vimentin/desmin in skeletal muscle cells demonstrated by three-dimensional fluorescence digital imaging microscopy. Exp Cell Res. 1994. 214:447–458.
16. Amit M, Carpenter MK, Inokuma MS, Chiu CP, Harris CP, Waknitz MA, Itskovitz-Eldor J, Thomson JA. Clonally derived human embryonic stem cell lines maintain pluripotency and proliferative potential for prolonged periods of culture. Dev Biol. 2000. 227:271–278.
17. Sung HJ, Hong SH, Yoo JH, Oh JH, Shin HJ, Choi IY, Ahn KH, Kim SH, Park Y, Kim BS. Stemness evaluation of mesenchymal stem cells from placentas according to developmental stage: comparison to those from adult bone marrow. J Korean Med Sci. 2010. 25:1418–1426.