Abstract
Effective drug to manage constipation has been unsatisfactory. We sought to determine whether methionine has effect on the human colon. Human colon tissues were obtained from the specimens of colon resection. Microelectrode recording was performed and contractile activity of muscle strips and the propagation of the contractions in the colon segment were measured. At 10 µM, methionine depolarized the resting membrane potential (RMP) of circular muscle (CM) cells. In the CM strip, methionine increased the amplitude and area under the curve (AUC) of contractions. In the whole segment of colon, methionine increased the amplitude and AUC of the high amplitude contractions in the CM. These effects on contraction were maximal at 10 µM and were not observed in longitudinal muscles in both the strip and the colon segment. Methionine reversed the effects of pretreatment with sodium nitroprusside, tetrodotoxin and Nw-oxide-L-arginine, resulting in depolarization of the RMP, and increased amplitude and AUC of contractions in the muscle strip. Methionine treatment affected the wave pattern of the colon segment by evoking small sized amplitude contractions superimposed on preexisting wave patterns. Our results indicate that a compound mimicking methionine may provide prokinetic functions in the human colon.
Chronic constipation is a major health problem. It is chronic in nature and dramatically affects the patient's quality of life, causing tremendous impact on both the individual patient and society as a whole (1). To cope with this problem, many kinds of prokinetic drugs with different mechanisms have been used. However, the effectiveness of the available drugs has been limited. Cholinergic agents such as bethanechol and neostigmine have a limited role in the management of constipation because they have broad muscarinic effects and need continuous monitoring of electrocardiography, blood pressure and oxygen saturation during medication use (2). Dopamine receptor antagonists such as metoclopromide (3, 4), and domperidone (5-7) have not shown clinically significant effects on large bowel motility (8). Tegaserod (9, 10) and cisapride (11), which are serotonin receptor modulators (12), emerged as the most promising drugs for chronic constipation. However, production of these drugs has been suspended due to their severe cardiac side effects (4, 13, 14). Successive results have shown that development of new effective drugs to manage chronic constipation has been unsatisfactory.
We have previously demonstrated that the sulfur-containing amino acid methionine acts as a specific blocker of the stretch-dependent potassium channels and nitrergic responses in the mouse colon (15). As such, methionine has the effect of depolarizing the resting membrane potential of the murine colon muscles and enhancing spontaneous contractions. Based on this data, we sought to determine whether methionine has any effect on the human colon in vitro. Since colonic motility is determined by both the electrophysiologic and mechanical characteristics, we examined the motility effects of methionine at the muscle cell level, muscle bundle level, and in the whole colon segment level.
Human colon tissues were obtained from the specimens of patients undergoing elective colon resections for non-obstructive neoplasms. The tissues were immediately stored in oxygenated Krebs-Ringer's bicarbonate (KRB) solution.
For intracellular recording of circular smooth muscle, the tissues were transferred to a Petri dish coated with Sylgard (Dow Corning Co., Midland, MI, USA) and first pinned downed with the mucosa side facing upward. The mucosal layer was removed by sharp dissection leaving the submucosal layer intact. For the final electrophysiologic recording of the inner circular muscle, the muscles were pinned with the submucosa facing down in the electrophysiologic chamber and longitudinal and outer circular muscles were finely removed by sharp dissection. For the intracellular recording from the longitudinal smooth muscle, thin strips (1.5 mm thick and 10 mm long) were cut parallel to the longitudinal muscle with a double-bladed scalpel, and pinned down to expose a cross section of the entire muscle layer. The electrophysiologic chamber was constantly perfused with prewarmed, preoxygenated Krebs solution, maintaining the temperature at 37.5 ± 0.5℃. The muscles were equilibrated for at least 2 hr before experiments. A conventional microelectrode recording was performed using a sharp microelectrode filled with 3 M/L KCl. Membrane potentials were measured with a high-input resistance electrometer, and outputs were displayed on oscilloscope. Agar bridge reference electrode (Warner instruments, Harvard Apparatus company, Hamden, CT, USA) was used because the sulfur compound in methionine reacts with Ag. Resting membrane potentials were measured using pClamp software (version 9.0. Axon Instruments, Foster City, CA, USA) and Origin software (MicroCal Software, Northampton, MA, USA) programs. Resting membrane potential (mV), amplitude (mV) and frequency (/min) of the slow waves were analyzed.
For preparation of the muscle strip, mucosa and submucosal layers were removed and longitudinal and circular muscle bundles were obtained by sharp dissection. The size of the muscle strips were 2 mm in width and 1 cm in length. For contractile activity recording, the muscle strips were attached with a suture to a force transducer in an organ bath and a resting force of 1 g was applied. The mechanical signals were digitized and recorded on Acknowledge software (Biopac Systems Inc., Goleta, CA, USA) for data analysis. Frequency (/min), amplitude (mN), and area under the curve (AUC, sec × mN/min) of contractions were analyzed. In the muscle strip recording, the area under the curve was defined as the integrated area under the waves occurring in one minute (AUC for one minute).
The colonic segment with the mucosa and submucosa intact was prepared by cutting the whole layer of the colon segment parallel to the longitudinal muscle. The size of the colon segment was 4 cm in length and 2 cm in width and included the taenia coli. A stainless steel rod was placed parallel to the longitudinal muscle and placed at the organ bath. To record the propagation pattern within the colonic segment, circular smooth muscle (CM) tension was recorded at three (oral, middle, aboral) sites, and longitudinal smooth muscle (LM) tension was recorded by perpendicular traction. The contractile activity was measured as described above. In colonic segment recordings, the area under the curve was defined as the integrated area under a single wave (AUC for one wave).
The KRB used in this study contained (in mM/L) 120.4 NaCl, 5.9 KCl, 15.5 NaHCO3, 11.5 glucose, 1.2 MgCl2, 1.2 NaH2PO4, and 2.5 CaCl2. This solution had a pH of 7.3-7.4 at 37.5℃ when bubbled to equilibrium with 97% O2-3% CO2.
L-methionine, sodium nitroprusside (SNP), tetrodotoxin (TTX) and Nw-oxide-L-arginine (L-NA) were obtained from Sigma Chemical Company (St Louis, MO, USA).
All data are expressed as mean ± standard deviation. Student's paired t-tests were used to determine if data sets differed, and P values of less than 0.05 were taken to indicate significant differences between sets of observations. The n values reported in the text refer to the number of recordings from the muscle strips, which is equivalent to the number of patients used unless otherwise stated.
Electrical activity in the normal human colonic circular smooth muscle (CM) is characterized by slow waves originating from interstitial cells of Cajal (ICC) in the submucosal layer (Fig. 1).
First, circular smooth muscle (CM) was treated with up scaling concentrations of methionine at 5, 10, 100, and 500 µM to 3, 12, 6, and 3 tissues, respectively. Methionine depolarized the resting membrane potential (RMP) of CM, and this was most significant when treated with 10 µM methionine (control -50.5 ± 6.4 vs 10 µM methionine -48.9 ± 7.0 mV; P = 0.018; n = 12) (Fig. 1). 10 µM methionine did not have a significant effect on the amplitude (control 29.87 ± 6.37 mV vs methionine 27.64 ± 10.31 mV, P = 0.164) and frequency (control 9.09 ± 6.70/min vs methionine 11.3 ± 13.31/min, P = 0.427) of the slow waves in the CM.
The NO donor SNP (100 µM) induced hyperpolarization of CM from -51.00 ± 12.29 mV to -55.45 ± 13.42 mV and subsequent application of 10 µM methionine induced depolarization to -48.43 ± 16.59 mV. Methionine seems to have a tendency (P = 0.064, n = 6) to reverse the effect of SNP (Fig. 2A) in CM.
TTX (1 µM) depolarized the membrane potential of CM from -62.10 ± 8.83 mV to -56.48 ± 8.75 mV and subsequent addition of 10 µM methionine caused significant (P = 0.033, n = 7) additional depolarization of the membrane potential to -51.81 ± 18.05 mV (Fig. 2B).
Most GI muscles have tonic inhibitory drive due to the spontaneous production and release of NO. 100 µM L-NA, an inhibitor of nitric oxide synthase, caused depolarization of CM from -66.80 ± 11.89 mV to -65.36 ± 13.00 mV, suggesting a substantial basal production of NO in human colonic muscles. In the presence of L-NA, treatment with 10 µM methionine caused small but significant (P = 0.038, n = 6) additional depolarization to -64.65 ± 11.82 mV (Fig. 2C).
In the circular muscle (CM) strip, 10 µM methionine increased the amplitude (control 17.2 ± 13.2 vs methionine 27.8 ± 11.2 mN; P = 0.04; n = 6) and the area under the curve (AUC, control 266.4 ± 159.3 vs methionine 420.3 ± 151.5 mN X sec/min; P = 0.006; n = 6) of contractions. The frequency (control 2.24 ± 1.39/min vs methionine 2.48 ± 1.85/min; P = 0.342; n = 6) of contractions increased but it was not significant (Fig. 3A).
10 µM methionine was applied to 7 longitudinal smooth muscle strips, but had no significant effect on frequency (control 1.30 ± 0.99 vs methionine 1.05 ± 0.97/min; P = 0.556), amplitude (22.74 ± 10.97 vs methionine 19.76 ± 10.34 mN; P = 0.163) and AUC (394.14 ± 235.66 vs 278.45 ± 167.35 mN × sec/min; P = 0.117).
In CM, 1 µM TTX attenuated the contractile activity and subsequent addition of 10 µM methionine reversed this effect and enhanced the contraction. 10 µM methionine overcame the neurogenic block effect of 1 µM TTX and increased the frequency (control 9.59 ± 5.44, TTX 3.65 ± 3.16, TTX + methionine 11.08 ± 5.84 mN; P = 0.011; n = 6) and AUC (control 84.45 ± 25.48, TTX 21.40 ± 16.73, TTX + methionine 99.66 ± 5.89 mN × sec/min; P = 0.011), significantly (Fig. 3B).
In CM, 100 µM L-NA enhanced the contractile activity, and subsequent treatment of 10 µM methionine resulted in an additional increase in the amplitude (control 12.45 ± 5.32, L-NA 16.03 ± 5.50, L-NA + methionine 17.74 ± 7.96 mN; P = 0.038; n = 4) and AUC, significantly (Fig. 3C).
In the contractile activity of the whole colonic segment, two different wave patterns were mixed in CM with variable ratios. We defined the one with higher amplitude as the dominant wave (DW) which was observed both in the CM and LM layer, and smaller amplitude waves were defined as non-dominant (non-DW) waves observed only in the CM layer.
First, the whole colonic segment was treated with upscaling concentrations of 5, 10, 100, and 500 µM methionine to 3, 9, 6, and 3 tissues, respectively. The methionine effect was maximal at the 10 µM concentration and increased the amplitude (control 18.61 ± 9.81 vs 10 µM methionine 22.92 ± 10.52 mN; P = 0.027; n = 9) and AUC in the DW (control 665.08 ± 87.23 vs 10 µM methionine 805.78 ± 649.69 mN × sec/wave; P = 0.026; n = 9) of the CM (Fig. 4).
10 µM methionine did not have a significant effect on the frequency, amplitude and AUC in the DW of the longitudinal smooth muscle (P = 0.427, 0.696, 0.915, respectively).
Methionine treatment also had an effect on the wave patterns of the colon segment by evoking small sized amplitude contractions (non-DWs), superimposed on preexisting wave patterns (DW). In 3 out of 11 samples, methionine treatment evoked non-DWs which were not present in the control state (Fig. 5). Significance of this finding is not clear at the moment, but since non-DWs are presumed to have a retrograde propagation function (our unpublished data), methionine may also enhance mixing of colonic contents.
Prokinetic agents are drugs that enhance motor activity of the smooth muscle in the gastrointestinal tract. These drugs have been well used in upper gastrointestinal tract motility disorders such as gastroesophageal reflux disease, diabetic gastropathy, irritable bowel syndrome and bile acid reflux gastritis (11, 16-18). A clear mechanism of how these drugs work is not well known, but it is believed that these drugs enhance the motility agonists or antagonize inhibitory transmitters and thus improve motility (19, 20).
On the contrary, agents that could be used in colonic motility disorders have not been sufficiently investigated. Lower GI motility disorders that seem to have benefit from prokinetics are postoperative ileus, acute colonic pseudoobstruction, chronic intestinal pseudoobstruction, idiopathic constipation, and constipation secondary to a primary disease (such as dementia, multiple sclerosis, diabetic neuropathy, spinal cord injury, Hirschsprung's disease, Chagas' disease, myopathic intestinal pseudoobstruction) (21). Prokinetic agents used clinically are cholinergic agents, dopamine, receptor antagonists, and serotonin receptor modulators. Since cholinergic agents such as acetylcholine act on muscarinic cholinergic receptors, they also cause non-specific and broad muscarinic effects (2). Domperidone and metoclopromide, known dopamine D2 receptor antagonists, act most effectively on the upper GI tract. The only agents that are effective on colonic motility are cisapride (11) and tegaserod maleate (9, 10) which are serotonin receptor modulators (12). Cisapride is an antagonist to 5-HT (hydroxytryptamine) and acts as a 5-HT4 agonist to powerfully enhance GI motility. However, this agent caused serious cardiac arrthymias by acting on the HERG channel and was suspended from the market. Tegaserod maleate is a non-specific 5-HT4 receptor agonist, and has been widely used in Europe for managing irritable bowel syndrome and chronic idiopathic constipation but was suspended because it also caused serious cardiac side effects (13, 14). So for the time being, there seems to be no proper agents that can be used in colonic motility disorders.
Thorough understanding of the mechanism of human colonic motility is essential to develop drugs effective in colonic motility. There are studies on colonic manometry in which a probe is colonoscopically inserted into the whole colon and motility is scanned for 24 hr (22, 23). According to those studies, normal colonic function consists of frequent high pressure waves which propagate from the oral to the anal side, which are four times more frequent in the daytime, especially after waking up and after meals. There were also retrograde propagating waves from the anal to the oral side, which are two times more frequent after meals. However, there are limitations in these studies. Since colonic manometry studies are done in vivo, artificial manipulation such as drug treatment is impossible. So studies manipulating the human colonic tissues in vitro are indispensable. Most of the studies on colonic smooth muscle tissue are performed in animals such as mice, dogs and guinea pigs.
Not so much is known about the electromechanical properties of human colonic smooth muscle. In this study, the control group (records before treating drugs) showed no difference between the right and left colon at the muscle cell, muscle strip and colon segment levels. There was a mutual contraction between CM and LM, and the contraction waves had similar frequency, amplitude and area under the curve (which implies the actual contractile force).
The sulfur containing amino acid methionine is an essential amino acid, which has been known to enhance the contractile activity in coronary smooth muscle by increasing the N-methylation of phospholipids which comprise the cell membrane and control the intracellular calcium reaction (24-26). However, its effect in human gastrointestinal smooth muscle has not been studied in detail. In this paper, we sought to examine the effects of methionine, which has proved to have a prokinetic effect in murine colon. We have shown that methionine depolarized the resting membrane potential of the human colon CM in the electrophysiologic study. In contractile studies, methionine increased the amplitude and area of contractions in the circular muscle strip. The increased contractile effect of methionine was also evident in the whole colonic segment by increased amplitude and area of contractions in the circular muscle layer. The enhanced effect on the contractile activity was observed only in circular smooth muscle but not in the longitudinal smooth muscle.
In the normal state, gastrointestinal smooth muscle cells are kept in the inhibitory state through various inhibitory neuronal inputs, of which NO is the most prominent inhibitory input. NO is released by non-adrenergic, non-cholinergic (NANC) inhibitory neurons in gastrointestinal (GI) smooth muscles and serves as the primary enteric inhibitory neurotransmitter in GI muscles, and nitrergic neurons regulate gut tone, phasic contractile amplitude and frequency, and inhibitory reflexes (27, 28). NO induces hyperpolarization of membrane potential which results in reduced smooth muscle excitability. A recent study in mice suggests that part of the hyperpolarizing effects of NO may be mediated by stretch-dependent potassium (SDK) channels that are expressed in GI smooth muscles (29). In the mouse, the two-pore K+ channel, TREK-1, encodes SDK channels in murine GI smooth muscle cells, especially in the colon (29, 30). It has been noted that sulfur-containing amino acids block SDK channels in colonic myocytes, and that compounds of this class are effective in blocking NO dependent responses in intact muscles. In murine mice, methionine blocked SDK channels in colonic myocytes, depolarized membrane potentials and increased the frequency of contractions in intact muscles. These compounds also inhibited responses to the NO donor sodium nitroprusside (SNP) and neurally released NO (15).
In this paper, we tried to determine whether a similar phenomenon occurs in human colonic muscles. We pretreated with TTX (a sodium channel blocker which blocks all neurogenic control on gastrointestinal smooth muscle), L-NA (NO synthase inhibitor which enhances gastrointestinal smooth muscle contractility) and SNP (a spontaneous NO releasing compound which hyperpolarizes the resting membrane potential). Our results from this study indicated that methionine had the effect of overcoming the spontaneous neuronal effect and release of NO and reversed the effect of nitrergic stimulation in the human colonic muscle strip. In the whole colon segment, L-NA enhanced the contractions which were potentiated with the addition of methionine. However, effects of methionine after pretreatment with SNP and TTX in whole colon segments were variable (data not shown). This could be due to complex interactions of the intact intrinsic nervous system in the whole colon segment.
The sulfur containing amino acid methionine seems to be a compound that could be a candidate for prokinetic drugs in the human colon. However, methionine is transformed into homocysteine during bio-metabolism. Surplus accumulation of homocysteine in the human body induces atherosclerosis, and increases the risk of Alzheimer's disease and coronary artery disease (31-33). For this reason, methionine cannot be directly used to enhance colonic motility in humans. But based on our findings, development of a new compound that mimics methionine, devoid of cardiac side effects, may have a role as an effective prokinetic agent for the human colon.
Figures and Tables
Fig. 1
Effect of methionine on the membrane potential of human colon circular muscle. (A) Methionine at 10 µM concentration caused depolarization of the resting membrane potential. (B) Various concentrations of methionine (5, 10, 100, 500 µM) were used to treat circular muscle. 10 µM of methionine treatment resulted in maximal depolarization in the resting membrane potential of circular muscle. The figure shows the resting membrane potential according to the concentration of methionine.
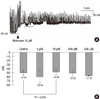
Fig. 2
Effect of methionine on the membrane potential of human colon circular muscle in the presence of pretreated drugs. Pretreatment drug was perfused at 15 min intervals to 10 µM methionine. (A) Pretreating with 100 µM SNP hyperpolarized the RMP. 10 µM of methionine depolarized the RMP and overcame the effect of SNP. (B) Pretreating with 1 µM TTX depolarized the RMP. 10 µM methionine depolarized the RMP and had additional effect over TTX. (C) Pretreating with 100 µM NOLA depolarized the RMP. 10 µM methionine depolarized the RMP and had additional effect over NOLA. *The horizontal line is the reference to compare the RMP after drug treatment.
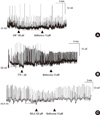
Fig. 3
Effect of methionine on the contraction of human colon CM strip. (A) After 10 µM methionine treatment on CM, amplitude and AUC for one minute increased. The black vertical line indicates the starting point of 10 µM methionine perfusion. (B) First KRB solution, then 1 µM TTX and 10 µM methionine were perfused at 45 min time intervals. TTX had a neurogenic blocking effect and inhibited the contraction in the CM strip. Note that after perfusing 10 µM methionine in the presence of TTX, the amplitude and area under the curve for one minute increased, indicating that 10 µM of methionine treatment had overcome the blocking effect of TTX and enhanced the contraction of the CM strip. The data in the open circle is magnified at the second row. (C) First KRB solution, then 100 µM NOLA and 10 µM methionine was perfused at 45 min time intervals. NOLA enhanced the contraction in the CM strip. Note that after perfusing 10 µM methionine, the amplitude and AUC increased further. 10 µM of methionine treatment had additional effect over NOLA.
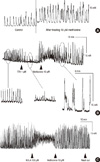
Fig. 4
Effect of methionine on human colon segment tension. (A) Methionine at 10 µM concentration caused and increase in amplitude and AUC in the dominant waves of the circular muscle. (B) Various concentrations of methionine (10, 50, 100 µM) were used \to treat the circular muscle of the human colon segment. 10 µM methionine treatment resulted in a maximal increase in amplitude and AUC of the dominant waves in the circular muscle of the colon segment. The figure shows the amplitude according to the concentration of treated methionine. (C) 10 µM of methionine had no effect on longitudinal muscle motility.
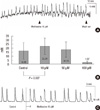
ACKNOWLEDGMENTS
The authors thank Professor In-suk So, Department of Physiology, Seoul National University College of Medicine, for kindly providing assistance for the experimental works.
References
1. Brandt LJ, Prather CM, Quigley EM, Schiller LR, Schoenfeld P, Talley NJ. Systematic review on the management of chronic constipation in North America. Am J Gastroenterol. 2005. 100:S5–S21.
2. Longo WE, Vernava AM 3rd. Prokinetic agents for lower gastrointestinal motility disorders. Dis Colon Rectum. 1993. 36:696–708.
3. Sanger GJ. Effects of metoclopramide and domperidone on cholinergically mediated contractions of human isolated stomach muscle. J Pharm Pharmacol. 1985. 37:661–664.
4. Hasler WL. Gastroparesis: symptoms, evaluation, and treatment. Gastroenterol Clin North Am. 2007. 36:619–647.
5. Prakash A, Wagstaff AJ. Domperidone. A review of its use in diabetic gastropathy. Drugs. 1998. 56:429–445.
6. Wiley J, Owyang C. Dopaminergic modulation of rectosigmoid motility: action of domperidone. J Pharmacol Exp Ther. 1987. 242:548–551.
7. Milo R. Use of the peripheral dopamine antagonist, domperidone, in the management of gastro-intestinal symptoms in patients with irritable bowel syndrome. Curr Med Res Opin. 1980. 6:577–584.
8. Karamanolis G, Tack J. Promotility medications: now and in the future. Dig Dis. 2006. 24:297–307.
9. Degen L, Matzinger D, Merz M, Appel-Dingemanse S, Osborne S, Lüchinger S, Bertold R, Maecke H, Beglinger C. Tegaserod, a 5-HT4 receptor partial agonist, accelerates gastric emptying and gastrointestinal transit in healthy male subjects. Aliment Pharmacol Ther. 2001. 15:1745–1751.
10. Prather CM, Camilleri M, Zinsmeister AR, McKinzie S, Thomforde G. Tegaserod accelerates orocecal transit in patients with constipation-predominant irritable bowel syndrome. Gastroenterology. 2000. 118:463–468.
11. Coremans G, Janssens J, Vantrappen G, Chaussade S, Ceccatelli P. Cisapride stimulates propulsive motility patterns in human jejunum. Dig Dis Sci. 1988. 33:1512–1519.
12. Spiller R. Serotonergic modulating drugs for functional gastrointestinal diseases. Br J Clin Pharmacol. 2002. 54:11–20.
13. Danielsson BR, Danielsson C, Nilsson MF. Embryonic cardiac arrhythmia and generation of reactive oxygen species: common teratogenic mechanism for IKr blocking drugs. Reprod Toxicol. 2007. 24:42–56.
14. Schoenfeld P. Review article: the safety profile of tegaserod. Aliment Pharmacol Ther. 2004. 20:25–30.
15. Park KJ, Baker SA, Cho SY, Sanders KM, Koh SD. Sulfur-containing amino acids block stretch-dependent K+ channels and nitrergic responses in the murine colon. Br J Pharmacol. 2005. 144:1126–1137.
16. Bright-Asare P, El-Bassoussi M. Cimetidine, metoclopramide, or placebo in the treatment of symptomatic gastroesophageal reflux. J Clin Gastroenterol. 1980. 2:149–156.
17. McCallum RW. Review of the current status of prokinetic agents in gastroenterology. Am J Gastroenterol. 1985. 80:1008–1016.
18. Bailey LD Jr, Stewart WR Jr, McCallum RW. New directions in the irritable bowel syndrome. Gastroenterol Clin North Am. 1991. 20:335–349.
19. Reynolds JC. Prokinetic agents: a key in the future of gastroenterology. Gastroenterol Clin North Am. 1989. 18:437–457.
20. Reynolds JC, Putnam PE. Prokinetic agents. Gastroenterol Clin North Am. 1992. 21:567–596.
21. Lacy BE, Weiser K. Gastrointestinal motility disorders: an update. Dig Dis. 2006. 24:228–242.
22. Herbst F, Kamm MA, Morris GP, Britton K, Woloszko J, Nicholls RJ. Gastrointestinal transit and prolonged ambulatory colonic motility in health and faecal incontinence. Gut. 1997. 41:381–389.
23. Rao SS, Sadeghi P, Beaty J, Kavlock R, Ackerson K. Ambulatory 24-h colonic manometry in healthy humans. Am J Physiol Gastrointest Liver Physiol. 2001. 280:G629–G639.
24. Kihara Y, Inoko M, Sasayama S. L-methionine augments mammalian myocardial contraction by sensitizing the myofilament to Ca2+. Circ Res. 1995. 77:80–87.
25. Panagia V, Gupta MP, Ganguly PK, Dhalla NS. Methionine-induced positive inotropic effect in rat heart: possible role of phospholipid N-methylation. Circ Res. 1988. 62:51–55.
26. Gupta MP, Panagia V, Dhalla NS. Phospholipid N-methylation-dependent alterations of cardiac contractile function by L-methionine. J Pharmacol Exp Ther. 1988. 245:664–672.
27. Sanders KM, Ward SM. Nitric oxide as a mediator of nonadrenergic noncholinergic neurotransmission. Am J Physiol. 1992. 262:G379–G392.
28. Ward SM, Dalziel HH, Thornbury KD, Westfall DP, Sanders KM. Nonadrenergic, noncholinergic inhibition and rebound excitation in canine colon depend on nitric oxide. Am J Physiol. 1992. 262:G237–G243.
29. Koh SD, Sanders KM. Stretch-dependent potassium channels in murine colonic smooth muscle cells. J Physiol. 2001. 533:155–163.
30. Koh SD, Monaghan K, Sergeant GP, Ro S, Walker RL, Sanders KM, Horowitz B. TREK-1 regulation by nitric oxide and cGMP-dependent protein kinase. An essential role in smooth muscle inhibitory neurotransmission. J Biol Chem. 2001. 276:44338–44346.
31. Burrin DG, Stoll B. Emerging aspects of gut sulfur amino acid metabolism. Curr Opin Clin Nutr Metab Care. 2007. 10:63–68.
32. Stipanuk MH. Sulfur amino acid metabolism: pathways for production and removal of homocysteine and cysteine. Annu Rev Nutr. 2004. 24:539–577.
33. Hogeveen M, Blom HJ, Van Amerongen M, Boogmans B, Van Beynum IM, Van De Bor M. Hyperhomocysteinemia as risk factor for ischemic and hemorrhagic stroke in newborn infants. J Pediatr. 2002. 141:429–431.