Abstract
DNA methylation is one of the main epigenetic mechanisms and hypermethylation of CpG islands at tumor suppressor genes switches off these genes. To find novel DNA methylation markers in hepatocellular carcinoma (HCC), we performed pharmacological unmasking (treatment with 5-aza-2'-deoxycytidine or trichostatin A) followed by microarray analysis in HCC cell lines. Of the 239 promoter CpG island loci hypermethylated in HCC cell lines (as revealed by methylation-specific PCR), 221 loci were found to be hypermethylated in HCC or nonneoplastic liver tissues. Thirty-three loci showed a 20% higher methylation frequency in tumors than in adjacent nonneoplastic tissues. Correlation of individual cancer-related methylation markers with clinicopathological features of HCC patients (n = 95) revealed that the number of hypermethylated genes in HCC tumors was higher in older than in younger patients. Univariate and multivariate survival analysis revealed that the HIST1H2AE methylation status is closely correlated with the patient's overall survival (P = 0.022 and P = 0.010, respectively). In conclusion, we identified 221 novel DNA methylation markers for HCC. One promising prognostic marker, HIST1H2AE, should be further validated in the prognostication of HCC patients.
CpG islands are CpG dinucleotide-rich areas found within the promoter and 5' exonic regions of about 60%-70% of the human genes (1, 2). Aberrant hypermethylation on promoter CpG island loci can lead to inactivation of genes that are actively expressed or increased silencing of genes that are inactive. While both genetic mutations and promoter CpG island hypermethylation changes are simultaneously found in human cancer cells, recent studies have demonstrated that the number of genes inactivated by promoter CpG island hypermethylation is four-times higher than the number of genes undergoing mutations in colorectal cancers (3, 4). Besides its importance as a gene inactivation mechanism, promoter CpG island hypermethylation is gaining attention as a potential tumor biomarker. In this respect, DNA methylation markers are being actively investigated to detect human cancers in blood, secretions, or exfoliated cytology specimens, and to predict the risk of cancer progression and development (5-9).
Primary liver cancer is the fifth most common cancer worldwide and the third most common cause of death by cancer (10, 11). The overall incidence and mortality of hepatocellular carcinoma (HCC) has been decreasing in areas with high incidence (12). However, both the incidence and mortality rates of HCC are increasing in Western countries (13). The development of HCC is the consequence of a multistep process that involves several morphologically recognizable lesions and accumulation of molecular changes at the genetic and epigenetic levels. Several studies have reported HCC-associated genetic changes, including mutations in several tumor suppressor genes (TP53, p16, PTEN, IGF2R, and RB), oncogenes (c-MYC, c-MET, PIK3CA, and CTNNB1), and other cancer-associated genes (CDH1 and CCND1) (14, 15). Not only genetic changes but also epigenetic alterations underlie the evolution of HCC. Since hypermethylation of the CDH1 promoter CpG island locus was reported in HCC (16), about 150 other genes have been found to be hypermethylated in HCC in a cancer-specific manner (17-19). However, more genes undergoing hypermethylation of promoter CpG island loci are likely to be identified in HCC. Schuebel and colleagues reported that about 400 genes actively expressed in normal colon epithelial cells are inactivated by promoter CpG island hypermethylation in colorectal cancers (3).
Of the increasingly higher number of genes being identified as undergoing promoter CpG island hypermethylation in HCC, only a few have clinical relevance and potential utility as biomarkers for prediction of recurrence or poor prognosis (20-23). The primary aim of the present study is to discover novel cancer-related DNA methylation markers for HCC. We performed a large-scale gene expression microarray analysis, which enables the simultaneous characterization of the transcription profile of tens of thousands of genes, on HCC cell lines treated with demethylating agents. Of the genes up-regulated by treatment with demethylating agents, we selected those harboring CpG island loci in their promoter sequences. We then performed methylation-specific PCR (MSP) to determine whether promoter CpG island loci of the candidate genes were hypermethylated in HCC and whether this modification was cancer-specific. We found 2 promising cancer-specific methylated genes (HIST1H2AE and EFEMP2) that are associated with poor prognosis in patients with HCC.
A total of 8 HCC cell lines (SNU398, SNU475, SNU739, SNU761, SNU878, SNU886, HepG2, and Huh7) were obtained from the Korean Cell Line Bank (Seoul, Korea). All cell lines were maintained in RPMI-1640 medium with the exception of Huh7, which was maintained in Dulbecco's modified Eagle's medium (DMEM). HEPES buffer (25 mM), fetal bovine serum (FBS) (10%), penicillin (100 units/mL), and streptomycin (0.1 mg/mL) were added to the medium. We seeded 3 × 105 cells (from each of the cell lines) per milliliter of culture medium and treated them 24 hr later with 5 µM AZA (Sigma Chemical Co., St. Louis, MO, USA) for 96 hr, during which period media and drugs were changed every 24 hr. AZA was dissolved in acetic acid and diluted to 10 mg/mL. Cells were incubated for 72 hr and then treated with trichostatin A (TSA; Sigma) at 300 nM for 24 hr. For co-treatment of cells with AZA and TSA, AZA (5 µM) was added initially for 72 hr, after which it was removed, and AZA (5 µM) and TSA (300 nM) were added for an additional 24 hr. As a negative control, cell lines were treated with acetic acid in an equal volume of medium without the drug.
Formalin-fixed, paraffin-embedded archival tissues from 95 HCC patients (mean age 55 yr; 75 males and 20 females) were retrieved from the files of the Department of Pathology, Seoul National University Hospital (Seoul, Korea). These patients had undergone curative surgery for HCC from 2001 to 2004 at the Seoul National University Hospital. The degree of fibrosis in nonneoplastic livers was graded according to the Ishak scoring system. A liver with a fibrosis staging score of 5 or 6 was considered cirrhotic. For histological examination, tissue blocks containing tumors were selected and serially sectioned. Nonneoplastic liver tissues were obtained > 3 cm away from tumor regions and confirmed to be tumor-free by microscopic examination. We reviewed the electronic medical records of the patients to obtain clinicopathological information, including age, gender, Child-Pugh classification, and γ-glutamyltranspeptidase (GGT) and alpha-fetoprotein (AFP) levels. Fresh-frozen tissue samples of HCC and adjacent nonneoplastic liver were obtained from HCC patients (n = 10) who underwent curative resection for HCC at the Seoul National University Hospital in 2008.
Illumina's whole-genome expression arrays (Sentrix Human-Ref-8 Expression BeadChip; Illumina, Inc., San Diego, CA, USA) were used to analyze the RNA expression profiles of 5 HCC cell lines (SNU 398, SNU 761, SNU 878, HepG2, and Huh7) before and after treatment with AZA (5 µM) or TSA (300 nM).
For methylation analysis, 2 µg of genomic DNA obtained from HCC cell lines and tissue samples was modified using the EZ DNA Methylation™ Kit (Zymo Research, Orange, CA, USA) according to the manufacturer's protocol. The specific primers for bisulfite modified DNA were designed using MSPPrimer (http://www.mspprimer.org). For genes whose primer sequences could not be obtained by using the MSPPrimer software, MSP primers were designed using MethPrimer (http://www.urogene.org/methprimer). MSP primer sequences are available on request. MSP was performed as previously described (24).
We isolated total RNA from 8 HCC cell lines by using the RNeasy Mini Kit (Qiagen, Valencia, CA, USA) according to the manufacturer's protocol. A total of 5 µg of RNA was reverse-transcribed using Superscript III (Invitrogen, Carlsbad, CA, USA). Semi-quantitative real-time PCR was performed with 10 ng of cDNA in a 7300 Real-Time PCR System (Applied Biosystems, Foster City, CA, USA) by using the SYBR® Green PCR Master Mix (Applied Biosystems). Gene expression levels were normalized to the expression of GAPDH. Oligonucleotide sequences of primers and PCR conditions are available on request.
Associations between clinicopathological features and hypermethylated gene markers were analyzed using SPSS 13.0 for Windows. P values were based on Fisher's exact test or Pearson's chi-squared test. Survival was measured from the surgical resection day until death or the last clinical review before August 31, 2007. Overall survival was estimated by the method of the Kaplan-Meier log-rank test, and Cox proportional hazard analysis was used to estimate multivariate relationships between several clinicopathological and hypermethylated gene markers. P < 0.05 was considered significant.
The expression profiles of 5 HCC cell lines (SNU398, SNU761, SNU878, HepG2, and Huh7) were obtained before and after treatment with either AZA or TSA by using a microarray platform (Fig. 1). To identify genes undergoing hypermethylation-dependent expression changes, we determined the expression fold changes of individual genes between mock-treated and TSA- or AZA-treated cells, and plotted TSA- and AZA-related fold changes on the x- and y-axis, respectively (Fig. 2). The DNA demethylating agent (AZA) induces reexpression of densely hypermethylated and transcriptionally inactive genes, whereas the class I and II histone deacetylase inhibitor (TSA) does not induce reexpression (25, 26). Of the genes that did not show an increase in expression with TSA treatment (< 1.4-fold), subsets of genes displayed a peak of AZA-induced gene expression (> 2-fold). We considered the genes showing both < 1.4-fold expression with TSA treatment and > 2-fold expression with AZA treatment as candidate genes that might be inactivated by hypermethylation. In at least one of 5 cell lines, 793 genes were found to meet the selection criteria. Of these, genes that have no CpG islands in their promoters and proximal transcriptional start sites (TSS) as well as those genes whose methylation status in HCC had already been reported, were excluded from subsequent analysis (Fig. 1).
To identify whether the candidate genes (n = 443) are methylated in their promoter CpG island loci, we tried to design MSP primers by using the MSPprimer or MethPrimer software programs and successfully designed optimal primers for 380 genes. We analyzed the methylation status of these 380 genes in 8 HCC cell lines (SNU398, SNU475, SNU739, SNU761, SNU878, SNU886, HepG2, and Huh7) using MSP and found that 239 of 380 genes were methylated in one or more cell lines (Fig. 3), and that 167 genes were methylated in at least four cell lines. To identify the factors that might influence in their predisposition to DNA methylation, we compared the occupancy rate of Polycomb proteins (EED2 and SUZ12) and the frequency of the H3K27me3 modification between methylated genes and unmethylated genes in HCC cell lines by using the occupancy maps published for embryonic stem cells (27). Genes methylated in HCC cell lines had a higher frequency of SUZ12, EED, and H3K27me3 targets compared to genes not methylated in HCC cell lines (Fig. 4). Next, we counted the frequency of LINE-1 and ALU in upstream and downstream sequences around the transcription start site of the 380 genes and then compared the number of LINE-1 or ALU repeats between methylated and unmethylated genes in HCC cell lines. The number of ALU repeats was significantly higher in unmethylated genes than in methylated genes, whereas the number of LINE-1 was similar (Fig. 5).
In order to confirm whether CpG island loci methylated in cell lines are also methylated in primary tumor tissues, we analyzed 10 paired HCC and adjacent nonneoplastic liver tissues for their methylation status in 239 genes using MSP (Fig. 6). We subdivided all the genes (n = 360) into 6 groups (named 0-5) based on the comparison of methylation frequencies of individual genes between HCC and nonneoplastic liver tissues: 1) higher methylation frequency in HCC than in nonneoplastic liver (group 1, n = 56), 2) fully methylated in both HCC and non-neoplastic liver (group 2, n = 96), 3) not fully methylated but identical methylation frequencies in HCC and non-neoplastic liver (group 3, n = 17), 4) genes not methylated in HCC or non-neoplastic liver (group 4, n = 18), 5) genes less frequently methylated in HCC than in non-neoplastic livers (group 5, n = 52), and 6) unmethylated genes in HCC cell lines (group 0, n = 141) (Fig. 6). To identify key biological functions associated with a list of genes within each group, we performed functional annotation analysis using the DAVID tool (http://david.abcc.ncifcrf.gov/) (Table 1). Group 1 genes showed a significant association with the neuropeptide signaling pathway, positive regulation of amine transport, and acid secretion, whereas group 5 genes exhibited a significant association with nucleosome assembly, chromatin assembly, protein-DNA complex assembly, chromatin assembly or disassembly, and tissue development.
To ensure that the microarray data represent real changes in expression, we selected 7 genes (CRABP2, EFEMP2, HIST1H2AE, INA, NETO2, RASGRP2, and TP53I13) and analyzed their expression changes in HCC cell lines treated with AZA, TSA, or a combination of AZA and TSA using semi-quantitative real-time PCR (Fig. 7). Treatment with AZA alone resulted in induction of mRNA expression of these 7 genes. In some cases, the effect of combined AZA and TSA treatment was stronger than that of AZA or TSA alone. These data implicate that histone deacetylation may also be involved in the inhibition of gene transcription by DNA methylation.
Genes with methylation frequencies higher in HCC than in nonneoplastic liver tissues are more likely to play an important role in hepatocarcinogenesis. To identify a possible correlation of gene hypermethylation with clinicopathological features of HCC patients, we selected 33 genes showing a methylation difference higher than 19% between HCC and nonneoplastic liver samples (Fig. 8) and analyzed their methylation statuses in another set of HCC tissue samples (n = 95) by MSP. Hypermethylation was detected in 1 or more genes in all HCC samples. RNASE4 showed the highest methylation frequency (90%), followed by DUOXA1 (82%), CRABP2 (66%), and NETO2 (61%) (Fig. 9). The methylation status of the DNA methylation markers was correlated with clinicopathological features such as age, gender, tumor size, AFP and GGT levels, microscopic vascular invasion, and clinical outcome. The results of these association studies are summarized in Table 2. The number of methylated genes was significantly higher in older (≥ 55 yr) than in younger patients (11.7 vs 8.8, P = 0.030 by Student's t-test). NETO2, DHDH, MAP6D1, C1orf59, CYB5R2, ULBP1, SPINT1, and ZNF342 were more frequently hypermethylated in tumors from patients older than 55 yr than in tumors from patients younger than 55 yr (P < 0.05 for all genes). HSPA12B was more frequently methylated in tumors from female patients than in tumors from male patients (P = 0.008). RNASE4 was more frequently methylated in tumors smaller than 6 cm than in HCC tumors bigger than 6 cm (P = 0.018). The methylation frequencies of NETO2, DHDH, and SPINT1 were significantly higher in HCC cases with AFP production smaller than 100 ng/mL than in HCC cases with AFP production higher than 100 ng/mL, whereas the methylation frequency of RASGRP2 and HSPA12B was significantly lower in HCCs with low AFP production (< 100 ng/mL). INA, PPP1R14A, and ECEL1 were more frequently methylated in HCC patients with low serum GGT levels (< 56 ng/mL) than in HCC patients with high serum GGT levels (≥ 56 ng/mL) (P < 0.05 for all genes). The methylation frequencies of C1orf59, OXTR, and CYB5R2 were significantly lower in HCC tumors with microscopic vein invasion than in HCCs without microscopic vein invasion (P < 0.05 for all genes).
Correlation of DNA methylation markers with patient survival was analyzed in 90 patients. Five patients were excluded because of follow-up loss. Of the 33 CpG loci, HIST1H2AE and EFEMP2 exhibited an association between gene hypermethylation and poor prognosis (P = 0.022 and P = 0.081, respectively, by Kaplan-Meir log-rank test) (Fig. 10, Table 3). Among several other clinicopathological factors, tumor size, serum GGT levels, and microscopic vascular invasion showed prognostic significance. Taking into account these 3 clinicopathological factors, HIST1H2AE and EFEMP2 were included into a multivariate analysis to identify independent predictors of overall survival (Table 4). Multivariate analysis by using the COX proportional hazards model revealed that HIST1H2AE (P = 0.010) and EFEMP2 (P = 0.020) methylation status are significant variables affecting the overall survival of HCC patients. The methylation status of these two genes suggested about 2.6-fold increased risk as compared to patients who had these genes unmethylated.
In this study, we conducted a genome-wide microarray expression screening in 5 HCC cell lines treated with AZA or TSA and characterized a set of methylation-regulated genes. We were able to identify 221 novel DNA methylation markers in HCC. At the same time, we observed that a significant number of genes up-regulated after AZA treatment, were actually not methylated in HCC cell lines and primary HCC tissue samples. While the mechanisms by which AZA reactivates expression of unmethylated genes has not been elucidated yet, several possibilities exist. First, the up-regulation of unmethylated genes might be secondary to AZA-induced expression of upstream genes that encode transcription factors and are inactivated by promoter CpG island hypermethylation, Second, the expression of these genes might be regulated by the methylation status of CpG island shores rather than promoter CpG islands (28). Lastly, AZA might induce degradation of retinoblastoma protein (pRb) through the MDM2-dependent proteasome pathway. Because loss of pRb protein induces a significant decrease in recruitment of G9a and SUV39H1 to histones around the promoter of target genes and thus reduced H3K9 di- and tri-methylation, pRb degradation might result in re-expression of several silenced genes (Dr. Wei-Guo Zhu, personal communication).
In the present study, of the 380 genes containing CpG island loci in their promoter sequences and for which MSP oligonucleotide primers could be designed, 239 genes were found to be methylated in at least 1 HCC cell line. The remaining genes were found to be unmethylated in all HCC cell lines. The causes of selective predisposition to methylation are at present unclear. However, genome architecture has been recently proposed to strongly correlate with predisposition to DNA methylation. Specifically, genes with low frequency of retrotransposons (ALU and LINE-1) near the transcription start site or regulated by Polycomb-group protein binding are more prone to DNA methylation (29, 30). When we matched these data with ours, we also could observe that ALU and Polycomb-group proteins were significantly correlated with methylated status, whereas LINE-1 was not.
We analyzed 95 HCC samples for their methylation status in 33 CpG island loci and found that HCC tumors from older patients harbored more methylated genes than HCC tumors from younger patients. In addition, we found that 2 DNA methylation markers (HIST1H2AE and EFEMP2) are closely associated with poor prognosis of HCC patients. HIST1H2AE is a member of the histone H2A family. Histones are responsible for nucleosome structure and their appropriate balance is required for the correct assembly of chromatin as well as for proper cell division and growth (31, 32). H2a, one of the core histones, has nonallelic variants that are related by simple amino acid substitution (33). It is not clear whether these variants have functional significance and how the expression of individual histone genes is controlled. Among the 16 replication-dependent histone H2a genes, HIST1H2AE is included in the largest histone gene cluster (HIST1, including 55 histone genes) located on human chromosome 6 (34, 35). Previous studies have found that the expression of histone H2a genes is related to histone gene clusters with different promoters and different transcription factor binding sites in mouse hepatoma cell lines. However, the structure in the promoters and transcription binding sites responsible for such differential expression has not been determined yet. On the other hand, it has been reported that the H2a genes are regulated by histone H3K9 acetylation levels in the promoter regions (36). Similarly, we suggest that HIST1H2AE transcription might be influenced by histone acetylation based on the experimental results obtained by RT-PCR, using AZA-, TSA- or combined AZA/TSA-treated cell lines. The combinatory treatment showed to act synergistically in the reexpression of HIST1H2AE.
EFEMP2, also known as fibulin-4, is a novel extracellular matrix protein that belongs to the fibulin protein family (fibulin1-7). EFEMP2 is involved in the stabilization and organization of the extracellular matrix. Moreover, missense mutations of EFEMP2 (G169A) cause human genetic disorders (37-39). However, the role of EFEMP2 in driving tumorigenesis is still unclear and requires further study. A recent study has reported limited EFEMP2 methylation in melanoma cell lines (40). In contrast, our data have demonstrated a correlation of its methylation status with poor survival of HCC patients.
In conclusion, we have identified 221 novel DNA methylation markers for HCC by using a pharmacological unmasking microarray approach along with MSP. Of these, 55 DNA methylation markers are more frequently methylated in HCC than in adjacent nonneoplastic liver tissues. The biological functions of these genes are associated with neuropeptide signaling pathways and positive regulation of amine transport. HIST1H2AE methylation is strongly associated with poor prognosis of HCC patients. A future independent study is required to validate HIST1H2AE methylation as a prognostic marker for HCC.
Figures and Tables
Fig. 1
Flow chart for selection of candidate genes. Screening of candidate tumor suppressor genes (TSGs) was performed in 5 hepatocellular carcinoma (HCC) cell lines treated with 5 µM 5-aza-2'-deoxycytidine (AZA) or 300 nM trichostatin A (TSA) by using a 24,526-oligonucleotide mRNA microarray. We obtained 793 candidates whose gene expression did not increase with TSA treatment (< 1.4-fold) but increased more than 2-fold after AZA treatment. We excluded genes that do not harbor CpG islands in their promoters or whose methylation status in HCC tumors had already been reported in the literature. We further excluded genes for which adequate oligonucleotide primers could not be designed by using the MSPprimer or MethPrimer software programs. As a result, we selected 380 genes to be examined for their methylation status in HCC cell lines by using methylation-specific PCR (MSP).

Fig. 2
Hypermethylation-dependent expression changes. Gene expression changes for the indicated cells treated with trichostatin A (TSA) (x-axis) or 5-aza-2'-deoxycytidine (AZA) (y-axis) are plotted by log-fold change, and individual genes are shown in circles.

Fig. 3
Methylation-specific PCR (MSP) analysis of 380 selected genes in 8 hepatocellular carcinoma (HCC) cell lines. The methylated and unmethylated status is indicated by a gray and a white box, respectively.

Fig. 4
Frequency of H3K27me3 modification and occupancy rate of SUZ12 and EED in methylated (n = 239) and unmethylated genes (n = 141) in human embryonic stem cells. The chi-squared test was conducted to analyze the significance of the association.

Fig. 5
Comparison of ALU and LINE-1 repeats between methylated and unmethylated genes. For ALU counting, the promoter sequence of a specific gene was divided into 20 bins of 1-kb sequence each (10 bins upstream and 10 bins downstream of each gene transcription start site), and the presence of ALU was annotated for each bin. We counted bins containing ALU within a 1-kb sequence. For LINE-1 counting, the promoter sequence of a specific gene was divided into 7 bins of 1-kb sequence each (2 bins upstream and 5 bins downstream of each gene transcription site), and the presence of LINE-1 was annotated for each bin. Bins containing LINE-1 within a 1-kb sequence were counted. Student's t-test was performed to determine the statistical significance of the difference of means between 2 groups.

Fig. 6
Methylation-specific PCR (MSP) analysis of 239 genes in 10 pairs of tumor and surrounding nontumor tissue. The methylated and unmethylated status is indicated by a gray and a white box, respectively. Genes were divided into subgroups based on the methylation pattern in hepatocellular carcinoma (HCC) tumors and surrounding nonneoplastic liver tissues (see main text).

Fig. 7
Effect of 5-aza-2'-deoxycytidine (AZA) and trichostatin A (TSA) on gene expression. RNA was isolated from hepatocellular carcinoma (HCC) cell lines left untreated or treated with AZA, TSA, or a combination of AZA and TSA. mRNA was reverse-transcribed, and gene expression was quantitated by real-time PCR and normalized to GAPDH expression.

Fig. 8
Methylation frequencies of 33 DNA methylation markers in HCC cell lines (n = 8) and tissue samples (10 paired HCC and non-neoplastic liver tissue samples). Methylation frequencies of these DNA methylation markers in HCC tissue samples were higher than those of non-neoplastic liver tissue samples: the differences were 20% or more.

Fig. 9
Methylation frequencies of 33 DNA methylation markers in hepatocellular carcinoma (HCC) tissue samples (n = 95). DNA methylation markers were distributed along the x-axis according to the decreasing order of methylation frequency.
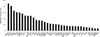
Fig. 10
Kaplan-Meier survival curves of 90 hepatocellular carcinoma (HCC) patients. Correlation of (A) HIST1H2AE, and (B) EFEMP2 methylation status with overall survival.

Table 1
Functional annotation analysis of classified genes. Gene ontology of hepatocellular carcinoma (HCC) tumors, surrounding nontumor tissues, and cell lines based on their DNA methylation patterns

References
1. Saxonov S, Berg P, Brutlag DL. A genome-wide analysis of CpG dinucleotides in the human genome distinguishes two distinct classes of promoters. Proc Natl Acad Sci U S A. 2006. 103:1412–1417.
2. Weber M, Hellmann I, Stadler MB, Ramos L, Pääbo S, Rebhan M, Schübeler D. Distribution, silencing potential and evolutionary impact of promoter DNA methylation in the human genome. Nat Genet. 2007. 39:457–466.
3. Schuebel KE, Chen W, Cope L, Glöckner SC, Suzuki H, Yi JM, Chan TA, Van Neste L, Van Criekinge W, van den Bosch S, et al. Comparing the DNA hypermethylome with gene mutations in human colorectal cancer. PLoS Genet. 2007. 3:1709–1723.
4. Wood LD, Parsons DW, Jones S, Lin J, Sjóblom T, Leary RJ, Shen D, Boca SM, Barber T, Ptak J, et al. The genomic landscapes of human breast and colorectal cancers. Science. 2007. 318:1108–1113.
5. Laird PW. The power and the promise of DNA methylation markers. Nat Rev Cancer. 2003. 3:253–266.
6. Maekita T, Nakazawa K, Mihara M, Nakajima T, Yanaoka K, Iguchi M, Arii K, Kaneda A, Tsukamoto T, Tatematsu M, et al. High levels of aberrant DNA methylation in Helicobacter pylori-infected gastric mucosae and its possible association with gastric cancer risk. Clin Cancer Res. 2006. 12:989–995.
7. deVos T, Tetzner R, Model F, Weiss G, Schuster M, Distler J, Steiger KV, Grützmann R, Pilarsky C, Habermann JK, et al. Circulating methylated SEPT9 DNA in plasma is a biomarker for colorectal cancer. Clin Chem. 2009. 55:1337–1346.
8. Tänzer M, Balluff B, Distler J, Hale K, Leodolter A, Röcken C, Molnar B, Schmid R, Lofton-Day C, Schuster T, et al. Performance of epigenetic markers SEPT9 and ALX4 in plasma for detection of colorectal precancerous lesions. PLoS One. 2010. 5:e9061.
9. Herbst A, Rahmig K, Stieber P, Philipp A, Jung A, Ofner A, Crispin A, Neumann J, Lamerz R, Kolligs FT. Methylation of NEUROG1 in serum is a sensitive marker for the detection of early colorectal cancer. Am J Gastroenterol. 2011. 106:1110–1118.
10. Parkin DM. Global cancer statistics in the year 2000. Lancet Oncol. 2001. 2:533–543.
11. El-Serag HB, Rudolph KL. Hepatocellular carcinoma: epidemiology and molecular carcinogenesis. Gastroenterology. 2007. 132:2557–2576.
12. Chang MH, You SL, Chen CJ, Liu CJ, Lee CM, Lin SM, Chu HC, Wu TC, Yang SS, Kuo HS, et al. Decreased incidence of hepatocellular carcinoma in hepatitis B vaccinees: a 20-year follow-up study. J Natl Cancer Inst. 2009. 101:1348–1355.
13. Jemal A, Siegel R, Ward E, Hao Y, Xu J, Thun MJ. Cancer statistics, 2009. CA Cancer J Clin. 2009. 59:225–249.
14. Herath NI, Leggett BA, MacDonald GA. Review of genetic and epigenetic alterations in hepatocarcinogenesis. J Gastroenterol Hepatol. 2006. 21:15–21.
15. Villanueva A, Newell P, Chiang DY, Friedman SL, Llovet JM. Genomics and signaling pathways in hepatocellular carcinoma. Semin Liver Dis. 2007. 27:55–76.
16. Kanai Y, Ushijima S, Hui AM, Ochiai A, Tsuda H, Sakamoto M, Hirohashi S. The E-cadherin gene is silenced by CpG methylation in human hepatocellular carcinomas. Int J Cancer. 1997. 71:355–359.
17. Calvisi DF, Ladu S, Gorden A, Farina M, Lee JS, Conner EA, Schroeder I, Factor VM, Thorgeirsson SS. Mechanistic and prognostic significance of aberrant methylation in the molecular pathogenesis of human hepatocellular carcinoma. J Clin Invest. 2007. 117:2713–2722.
18. Nishida N, Nagasaka T, Nishimura T, Ikai I, Boland CR, Goel A. Aberrant methylation of multiple tumor suppressor genes in aging liver, chronic hepatitis, and hepatocellular carcinoma. Hepatology. 2008. 47:908–918.
19. Shin SH, Kim BH, Jang JJ, Suh KS, Kang GH. Identification of novel methylation markers in hepatocellular carcinoma using a methylation array. J Korean Med Sci. 2010. 25:1152–1159.
20. Yuan Y, Wang J, Li J, Wang L, Li M, Yang Z, Zhang C, Dai JL. Frequent epigenetic inactivation of spleen tyrosine kinase gene in human hepatocellular carcinoma. Clin Cancer Res. 2006. 12:6687–6695.
21. Lu B, Ma Y, Wu G, Tong X, Guo H, Liang A, Cong W, Liu C, Wang H, Wu M, et al. Methylation of Tip30 promoter is associated with poor prognosis in human hepatocellular carcinoma. Clin Cancer Res. 2008. 14:7405–7412.
22. Lee HS, Kim BH, Cho NY, Yoo EJ, Choi M, Shin SH, Jang JJ, Suh KS, Kim YS, Kang GH. Prognostic implications of and relationship between CpG island hypermethylation and repetitive DNA hypomethylation in hepatocellular carcinoma. Clin Cancer Res. 2009. 15:812–820.
23. Zhang W, Zhou L, Ding SM, Xie HY, Xu X, Wu J, Chen QX, Zhang F, Wei BJ, Eldin AT, et al. Aberrant methylation of the CADM1 promoter is associated with poor prognosis in hepatocellular carcinoma treated with liver transplantation. Oncol Rep. 2011. 25:1053–1062.
24. Herman JG, Graff JR, Myöhänen S, Nelkin BD, Baylin SB. Methylation-specific PCR: a novel PCR assay for methylation status of CpG islands. Proc Natl Acad Sci U S A. 1996. 93:9821–9826.
25. Suzuki H, Gabrielson E, Chen W, Anbazhagan R, van Engeland M, Weijenberg MP, Herman JG, Baylin SB. A genomic screen for genes upregulated by demethylation and histone deacetylase inhibition in human colorectal cancer. Nat Genet. 2002. 31:141–149.
26. Christman JK. 5-Azacytidine and 5-aza-2'-deoxycytidine as inhibitors of DNA methylation: mechanistic studies and their implications for cancer therapy. Oncogene. 2002. 21:5483–5495.
27. Lee TI, Jenner RG, Boyer LA, Guenther MG, Levine SS, Kumar RM, Chevalier B, Johnstone SE, Cole MF, Isono K, et al. Control of developmental regulators by Polycomb in human embryonic stem cells. Cell. 2006. 125:301–313.
28. Irizarry RA, Ladd-Acosta C, Wen B, Wu Z, Montano C, Onyango P, Cui H, Gabo K, Rongione M, Webster M, et al. The human colon cancer methylome shows similar hypo- and hypermethylation at conserved tissue-specific CpG island shores. Nat Genet. 2009. 41:178–186.
29. Schlesinger Y, Straussman R, Keshet I, Farkash S, Hecht M, Zimmerman J, Eden E, Yakhini Z, Ben-Shushan E, Reubinoff BE, et al. Polycomb-mediated methylation on Lys27 of histone H3 pre-marks genes for de novo methylation in cancer. Nat Genet. 2007. 39:232–236.
30. Estécio MR, Gallegos J, Vallot C, Castoro RJ, Chung W, Maegawa S, Oki Y, Kondo Y, Jelinek J, Shen L, et al. Genome architecture marked by retrotransposons modulates predisposition to DNA methylation in cancer. Genome Res. 2010. 20:1369–1382.
31. Hereford LM, Osley MA, Ludwig TR 2nd, McLaughlin CS. Cell-cycle regulation of yeast histone mRNA. Cell. 1981. 24:367–375.
32. Marzluff WF, Duronio RJ. Histone mRNA expression: multiple levels of cell cycle regulation and important developmental consequences. Curr Opin Cell Biol. 2002. 14:692–699.
33. Nishida H, Suzuki T, Tomaru Y, Hayashizaki Y. A novel replication-independent histone H2a gene in mouse. BMC Genet. 2005. 6:10.
34. Albig W, Doenecke D. The human histone gene cluster at the D6S105 locus. Hum Genet. 1997. 101:284–294.
35. Albig W, Kioschis P, Poustka A, Meergans K, Doenecke D. Human histone gene organization: nonregular arrangement within a large cluster. Genomics. 1997. 40:314–322.
36. Nishida H, Suzuki T, Ookawa H, Tomaru Y, Hayashizaki Y. Comparative analysis of expression of histone H2a genes in mouse. BMC Genomics. 2005. 6:108.
37. Chu M, Tsuda T. Fibulins in development and heritable disease. Birth Defects Res C Embryo Today. 2004. 72:25–36.
38. de Vega S, Iwamoto T, Yamada Y. Fibulins: multiple roles in matrix structures and tissue functions. Cell Mol Life Sci. 2009. 66:1890–1902.
39. Hucthagowder V, Sausgruber N, Kim KH, Angle B, Marmorstein LY, Urban Z. Fibulin-4: a novel gene for an autosomal recessive cutis laxa syndrome. Am J Hum Genet. 2006. 78:1075–1080.
40. Furuta J, Nobeyama Y, Umebayashi Y, Otsuka F, Kikuchi K, Ushijima T. Silencing of Peroxiredoxin 2 and aberrant methylation of 33 CpG islands in putative promoter regions in human malignant melanomas. Cancer Res. 2006. 66:6080–6086.