Abstract
The aim of the present study was to evaluate the plaque components and the predictors of thin-cap fibroatheroma (TCFA) in anemic patients with acute coronary syndrome using virtual histology-intravascular ultrasound (VH-IVUS). Anemia was defined according to criteria of the World Health Organization, (i.e. , hemoglobin levels < 13 g/dL in men and < 12 g/dL in women) and we compared VH-IVUS findings between anemia group (171 patients, 260 lesions) and non-anemia group (569 patients, 881 lesions). Anemia group had greater % necrotic core (NC) volume (21% ± 9% vs 19% ± 9%, P = 0.001) compared with non-anemia group. Hemoglobin level correlated negatively with absolute NC volume (r = -0.235, P < 0.001) and %NC volume (r = -0.209, P < 0.001). Independent predictors of TCFA by multivariate analysis were diabetes mellitus (odds ratio [OR], 2.213; 95% confidence interval [CI], 1.403-3.612, P = 0.006), high-sensitivity C-reactive protein (OR, 1.143; 95% CI, 1.058-1.304, P = 0.012), microalbuminuria (albumin levels of 30 to 300 mg/g of creatinine) (OR, 2.124; 95% CI, 1.041-3.214, P = 0.018), and anemia (OR: 2.112; 95% CI 1.022-3.208, P = 0.028). VH-IVUS analysis demonstrates that anemia at the time of clinical presentation is associated with vulnerable plaque component in patients with acute coronary syndrome.
The incidence of anemia is relatively high in patients with coronary artery disease. Anemia is a cardiovascular risk factor in the general population (1), and was found to be associated with a significantly higher rate of cardiovascular events in patients with acute coronary syndrome (ACS) (2-4), heart failure (4, 5), in patients undergoing percutaneous coronary intervention (6, 7), and in patients undergoing coronary artery bypass graft surgery (8). Therefore, there is a possibility that anemia is associated with both development/progression of coronary atherosclerosis and coronary plaque vulnerability.
However, no study has examined the relation between anemia at the time of clinical presentation and coronary plaque characteristics in patients with ACS. Therefore, the purpose of the present study was to attempt to compare grey-scale and virtual histology-intravascular ultrasound (VH-IVUS) findings between patients with anemia and those without anemia at the time of clinical presentation of ACS.
We reviewed 740 consecutive patients with ACS who underwent grey-scale and VH-IVUS between January 2007 and September 2010. Anemia was defined according to criteria of the World Health Organization (i.e., hemoglobin levels < 13 g/dL in men and < 12 g/dL in women) (9). We compared baseline characteristics, coronary angiographic findings, and grey-scale and VH-IVUS findings between anemia group (171 patients, 260 lesions) and non-anemia group (569 patients, 881 lesions). The presence of unstable angina was determined by chest pain within the preceding 72 hr with or without ST-T-wave changes or positive cardiac biochemical markers (creatine kinase-myocardial band or cardiac specific troponin-I). The presence of ST-segment elevation myocardial infarction was determined by > 30 min of continuous chest pain, a new ST-segment elevation ≥ 2 mm on at least 2 contiguous electrocardiographic leads, and creatine kinase-myocardial band (MB) or cardiac-specific troponin I above 2 times of normal. The presence of non-ST-segment elevation myocardial infarction was diagnosed by chest pain and positive cardiac biochemical markers (creatine kinase-myocardial band or cardiac-specific troponin I) without new ST-segment elevation. Hypercholesterolemia was diagnosed in patients who received lipid-lowering medication or had a history of a low-density lipoprotein level > 130 mg/dL. Heart failure was defined as the presence of previous clinical signs and symptoms of pulmonary venous congestion or previous evidence of systolic dysfunction (left ventricular ejection fraction < 50%) on echocardiogram. We excluded patients with subacute or late stent thrombosis, totally occluded lesions, restenosis after stenting, coronary artery bypass graft failure, severe heart failure or cardiogenic shock, important systemic disease, or serum creatinine > 2.5 mg/dL, and patients in whom adequate IVUS images could not be obtained. Hospital records of all patients were reviewed to obtain clinical demographics and medical history.
Peripheral blood samples were obtained before coronary angiography using direct venipuncture. The blood samples were centrifuged, and serum was removed and stored at -70℃ until the assay could be performed. Absolute creatine kinase-MB levels were determined by radioimmunoassay (Dade Behring Inc., Miami, FL, USA). Cardiac-specific troponin I levels were measured by a paramagnetic particle, chemiluminescent immunoenzymatic assay (Beckman, Coulter Inc., Fullerton, CA, USA). The serum levels of total cholesterol, triglyceride, low-density lipoprotein cholesterol, and high-density lipoprotein cholesterol were measured by standard enzymatic methods. High-sensitivity C-reactive protein was analyzed turbidimetrically with sheep antibodies against human C-reactive protein; this has been validated against the Dade-Behring method (10). A random morning urine specimen was obtained. Urine creatinine and albumin were measured on a Kodak Ektachem 700 Analyzer and Beckman Array 360 CE protein Analyzer (Beckman Instruments, Fullerton, CA, USA), respectively. Patients with albumin levels of 30 to 300 mg/g of creatinine were defined as having microalbuminuria (11).
Coronary angiogram was analyzed with validated QCA system (Phillips H5000 or Allura DCI program, Philips Medical Systems, the Netherlands). With the outer diameter of the contrast-filled catheter as the calibration standard, the reference diameter and minimal lumen diameter were measured in diastolic frames from orthogonal projections. Perfusion was evaluated according to thrombolysis in myocardial infarction (TIMI) criteria (12).
All IVUS examinations were performed after intracoronary administration of 300 µg nitroglycerin using a commercially available IVUS system (Volcano Corp, Rancho Cordova, CA, USA). The IVUS catheter was advanced distal to the target lesion, and imaging was performed retrograde to the aorto-ostial junction at an automatic pullback speed of 0.5 mm/sec. In case of total occlusion, we opened the occluded artery by thrombus aspiration or balloon angioplasty using small balloon.
Gray-scale IVUS and VH-IVUS data were analyzed by 2 independent observers. The levels of reproducibility for external elastic membrane (EEM), lumen, and plaque plus media (P&M) cross-sectional areas (CSAs) using the Spearman rank-order correlation coefficients were 0.96, 0.97, and 0.97, respectively. Similarly, for plaque components by VH-IVUS, reproducibility for the fibrous (FT), fibro-fatty (FF), dense calcium (DC), and necrotic core (NC) volume measurements using the Spearman rank-order correlation coefficients were 0.93, 0.94, 0.93, and 0.93, respectively.
Quantitative volumetric gray-scale and VH-IVUS analyses were performed across the entire lesion segment, and cross-sectional analyses were performed at the minimum lumen sites and at the largest NC sites. Conventional quantitative volumetric gray-scale IVUS analysis was performed according to the American College of Cardiology Clinical Expert Consensus Document on Standards for Acquisition, Measurement and Reporting of Intravascular Ultrasound Studies (13). Measurements were made by every 1-mm interval for the region of interest, which was defined as the segment between distal to proximal reference sites that were the most normal looking within 5 mm proximal and distal to the lesion. References were the single slices with the largest lumen and smallest plaque CSAs within 10 mm proximally and distally. Hypoechoic plaque was less bright than the adventitia, hyperechoic noncalcified plaque was as bright as or brighter than the adventitia without acoustic shadowing, and hyperechoic calcified plaque was brighter than the adventitia with acoustic shadowing. When there was no dominant plaque composition, the plaque was classified as mixed.
Volumetric data were generated by the software using Simpson's method. EEM and lumen CSAs were measured. P&M CSA was calculated as EEM minus lumen CSA; and plaque burden was calculated as P&M divided by EEM CSA. VH-IVUS analysis classified the color-coded tissue into four major components: green (FT); yellow-green (FF); white (DC); and red (NC) (14-17). VH-IVUS analysis was reported as a percentage of plaque area or volume. We defined thin-cap fibroatheroma (TCFA) as a NC ≥ 10% of plaque area in at least 3 consecutive frames without overlying fibrous tissue in the presence of ≥ 40% plaque burden (15).
The statistical Package for Social Sciences (SPSS) for Windows, version 15.0 (Chicago, IL, USA) was used for all analyses. Continuous variables were presented as the mean ± SD; comparisons were conducted by Student's t-test or nonparametric Wilcoxon test if normality assumption was violated. Discrete variables were presented as percentages and relative frequencies; comparisons were conducted by chi-square statistics or Fisher's exact test as appropriate. Pearson's correlation coefficient was used to evaluate the associations between baseline hemoglobin level and each plaque components. Multivariate analysis was performed to determine the independent predictors of TCFA. A P value < 0.05 was considered statistically significant.
The baseline characteristics are summarized in Table 1. Patients with anemia were significantly older, were more females, and were more diabetics and were less smokers, and had more history of previous coronary intervention compared with those without anemia. Glucose level, high-sensitivity C-reactive protein, and urinary albumin-to-creatinine ratio were significantly higher in patients with anemia compared with those without anemia. Anemia group had significantly lower low-density lipoprotein cholesterol and apolipoprotein B levels compared with non-anemia group.
Angiographic findings are summarized in Table 2. Type B2/C lesions and multi-vessel diseases were observed more frequently and lesion length was significantly longer in patients with anemia compared with those without anemia. There were no significant differences in the target vessel, TIMI flow grade, minimal lumen diameter, and percent diameter stenosis between both groups.
Gray-scale IVUS results are summarized in Table 3. P&M CSAs were significantly greater at the proximal and distal references and at the minimum lumen site and at the largest NC site in patients with anemia compared with those without anemia. EEM CSAs were significantly greater at the distal references and at the largest NC site in patients with anemia compared with those without anemia. By volumetric analysis, anemia group had greater plaque volume and smaller lumen volume compared with non-anemia group.
At the minimum lumen site, absolute NC and DC areas were significantly greater and %NC area was significantly greater in patients with anemia compared with those without anemia (Fig. 1).
At the largest NC site, absolute NC, DC, and FT areas were significantly greater, and %NC and DC areas were significantly greater in patients with anemia compared with those without anemia; conversely %FT area was significantly smaller in patients with anemia compared with those without anemia (Fig. 2).
Absolute NC and DC volumes were significantly greater, and absolute FT and FF volumes were significantly smaller in patients with anemia compared with those without anemia; and %NC and DC volumes were significantly greater; and %FT and FF volumes were significantly smaller in patients with anemia compared with those without anemia (Fig. 3). TCFA was observed more frequently in patients with anemia compared with those without anemia (47% vs 35%, P < 0.001).
Hemoglobin level correlated negatively with absolute NC volume (r = -0.235, P < 0.001), %NC volume (r = -0.209, P < 0.001), absolute DC volume (r = -0.211, P < 0.001), %DC volume (r = -0.193, P < 0.001), and correlated positively with absolute FT volume (r = 0.205, P = 0.001), %FT volume (r = 0.164, P = 0.001), absolute FF volume (r = 0.187, P = 0.001), and %FF volume (r = 0.134, P < 0.001).
Multivariate analysis was performed to identify independent predictors of TCFA. The following variables were tested (all with P < 0.2 in univariate analysis) including microalbuminuria, age, male gender, diabetes mellitus, hypertension, smoking, ejection fraction, anemia, high-sensitivity C-reactive protein, and minimum lumen site plaque burden (all with P < 0.2 in univariable analysis). Independent predictors of TCFA included diabetes mellitus (odds ratio [OR], 2.213; 95% confidence interval [CI], 1.403-3.612, P = 0.006), high-sensitivity C-reactive protein (OR, 1.143; 95% CI, 1.058-1.304, P = 0.012), microalbuminuria (albumin levels of 30 to 300 mg/g of creatinine) (OR, 2.124; 95% CI, 1.041-3.214, P = 0.018), and anemia (OR, 2.112; 95% CI, 1.022-3.208, P = 0.028).
The present VH-IVUS study demonstrated that 1) patients with anemia had greater NC and DC components and had more TCFAs compared with those without anemia, 2) baseline hemoglobin level correlated negatively with NC and DC components and correlated positively with FT and FF components, and 3) anemia was the independent predictor of TCFA in patients with ACS.
Previous pathological studies have demonstrated that plaque rupture and subsequent thrombus formation is the most important mechanism leading to an ACS (18, 19). Plaque components may play a pivotal role in the plaque disruption and thrombosis that leads to acute coronary events (20-22). Several VH-IVUS studies have reported that ACS patients had more NC-containing lesions compared with stable angina patients (15, 23). In the present study which enrolled patients with ACS, mean %NC volume was about 21% and the proportion of patients with %NC volume > 20% was 53% of total patient population. Lesions with a large lipid core may have a higher risk for disruption than sclerotic plaques (24-26).
Anemia is present in 15%-30% of patients with ACS, and it adversely affects the outcomes of ACS (2-4). Anemia has the potential to worsen the myocardial ischemic insult in ACS, both by decreasing the oxygen delivery to myocardium downstream of coronary stenosis and by increasing myocardial oxygen demand through necessitating a higher stroke volume and heart rate to maintain adequate systemic oxygen delivery. The combination of these processes may explain the pathophysiology of poor clinical outcomes in patients with ACS with lower hemoglobin levels (2).
The significance of anemia in ACS has been focused on the significance of hemorrhagic complications during the treatment of ACS and on the prognostic significance of transfusions (27-29). No special attention has been devoted to the significance of anemia on its' relation to plaque characteristics in patients with ACS. In the present study, patients with anemia had greater plaque burden compared with those without anemia, and NC components were significantly greater, and TCFAs were observed more frequently in patients with anemia compared with those without anemia, and anemia was the independent predictor of TCFA. There are several postulated mechanisms that low hemoglobin levels are related to the development and progression of atherosclerosis and to the plaque vulnerability in patients with ACS. Anemia may worsen arterial intima-media thickening, and activate the sympathetic nervous system and renin-angiotensin-aldosterone axis, and impair oxygen carrying capacity and enhance levels of proinflammatory cytokines (30). Especially, anemia is associated with underlying inflammatory processes, so it is likely that patients with low hemoglobin levels might be more at risk of developing atherosclerosis and may affect the plaque vulnerability in patients with ACS.
There are several limitations to be mentioned. First, the analyses were retrospective and are subject to limitations inherent in this type of clinical investigation. Second, gray-scale and VH-IVUS imaging were performed at the discretion of the individual operators, leading to potential selection bias. Third, we did not attempt to differentiate between atherosclerotic plaque and thrombus because IVUS could not determine the presence of thrombus. This may obscure the identification of TCFA. Fourth, heavily calcified plaques may induce an artifact regarding the codification of plaques by VH-IVUS resulting in an increase in NC content. This remains a potential limitation of the present VH study. Fifth, nature of anemia (acute or chronic, causes of anemia, etc) was not evaluated in the present study. Sixth, patients with anemia had more comorbidities (more elderly patients, more females, more diabetics, higher high-sensitivity C-reactive protein, higher microalbuminuria, and so on) compared with those without anemia in the present study. Therefore, these more comorbidities may affect more vulnerable plaque characteristics in patients with anemia. However, in the present study, anemia itself was an independent predictor of large NC-containing plaque by multivariate analysis. So, it may be important to recognize hemoglobin as an indicator of plaque vulnerability.
In conclusion, anemia at the time of clinical presentation of ACS is associated with large plaque burden and vulnerable plaque characteristics in patients with ACS. Therefore, intensive medical therapies like high-dose statins may be needed for plaque regression and stabilization in patients with ACS who accompanies with anemia.
Figures and Tables
Fig. 1
Plaque component analysis according to the presence or absence of anemia at the minimum lumen sites. Results are displayed for (A) absolute and (B) relative quantifications. FT, fibrotic; FF, fibro-fatty; DC, dense calcium; NC, necrotic core.
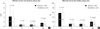
Fig. 2
Plaque component analysis according to the presence or absence of anemia at the largest necrotic core sites. Results are displayed for (A) absolute and (B) relative quantifications. FT, fibrotic; FF, fibro-fatty; DC, dense calcium; NC, necrotic core.

Fig. 3
Volumetric plaque component analysis according to the presence or absence of anemia. Results are displayed for (A) absolute and (B) relative quantifications. FT, fibrotic; FF, fibro-fatty; DC, dense calcium; NC, necrotic core.

Table 1
Baseline characteristics

Data are No. (%), or mean ± SD. NSTEMI, non-ST segment elevation myocardial infarction; STEMI, ST segment elevation myocardial infarction; CAD, coronary artery disease; PCI, percutaneous coronary intervention; hs-CRP, high-sensitivity C-reactive protein; LDL, low-density lipoprotein; HDL, high-density lipoprotein.
References
1. Sarnak MJ, Tighiouart H, Manjunath G, MacLeod B, Griffith J, Salem D, Levey AS. Anemia as a risk factor for cardiovascular disease in The Atherosclerosis Risk in Communities (ARIC) study. J Am Coll Cardiol. 2002. 40:27–33.
2. Sabatine MS, Morrow DA, Giugliano RP, Burton PB, Murphy SA, McCabe CH, Gibson CM, Braunwald E. Association of hemoglobin levels with clinical outcomes in acute coronary syndromes. Circulation. 2005. 111:2042–2049.
3. Nikolsky E, Aymong ED, Halkin A, Grines CL, Cox DA, Garcia E, Mehran R, Tcheng JE, Griffin JJ, Guagliumi G, Stuckey T, Turco M, Cohen DA, Negoita M, Lansky AJ, Stone GW. Impact of anemia in patients with acute myocardial infarction undergoing primary percutaneous coronary intervention: analysis from the Controlled Abciximab and Device Investigation to Lower Late Angioplasty Complications (CADILLAC) Trial. J Am Coll Cardiol. 2004. 44:547–553.
4. Archbold RA, Balami D, Al-Hajiri A, Suliman A, Liew R, Cooper J, Ranjadayalan K, Knight CJ, Deaner A, Timmis AD. Hemoglobin concentration is an independent determinant of heart failure in acute coronary syndromes: cohort analysis of 2310 patients. Am Heart J. 2006. 152:1091–1095.
5. Ezekowitz JA, McAlister FA, Armstrong PW. Anemia is common in heart failure and is associated with poor outcomes: insights from a cohort of 12065 patients with new-onset heart failure. Circulation. 2003. 107:223–225.
6. McKechnie RS, Smith D, Montoye C, Kline-Rogers E, O'Donnell MJ, DeFranco AC, Meengs WL, McNamara R, McGinnity JG, Patel K, Share D, Riba A, Khanal S, Moscucci M. Blue Cross Blue Shield of Michigan Cardiovascular Consortium (BMC2). Prognostic implication of anemia on in-hospital outcomes after percutaneous coronary intervention. Circulation. 2004. 110:271–277.
7. Lee PC, Kini AS, Ahsan C, Fisher E, Sharma SK. Anemia is an independent predictor of mortality after percutaneous coronary intervention. J Am Coll Cardiol. 2004. 44:541–546.
8. DeFoe GR, Ross CS, Olmstead EM, Surgenor SD, Fillinger MP, Groom RC, Forest RJ, Pieroni JW, Warren CS, Bogosian ME, Krumholz CF, Clark C, Clough RA, Weldner PW, Lahey SJ, Leavitt BJ, Marrin CA, Charlesworth DC, Marshall P, O'Connor GT. Northern New England Cardiovascular Disease Study Group. Lowest hematocrit on bypass and adverse outcomes associated with coronary artery bypass grafting. Ann Thorac Surg. 2001. 71:769–776.
9. Nutritional Anaemias. Report of a WHO Scientific Group. World Health Organization Technical Report Series No. 405. 1968. Geneva: World Health Organisation;9–10.
10. Roberts WL, Moulton L, Law TC, Farrow G, Cooper-Anderson M, Savory J, Rifai N. Evaluation of nine automated high-sensitivity C-reactive protein methods: implications for clinical and epidemiological applications. Part 2. Clin Chem. 2001. 47:418–425.
11. Eknoyan G, Hostetter T, Bakris GL, Hebert L, Levey AS, Parving HH, Steffes MW, Toto R. Proteinuria and other markers of chronic kidney disease: a position statement of the National Kidney Foundation (NKF) and the National Institute of Diabetes and Digestive and Kidney diseases (NIDDK). Am J Kidney Dis. 2003. 42:617–622.
12. TIMI IIIB Investigators. Effects of tissue plasminogen activator and a comparison of early invasive and conservative strategies in unstable angina and non-Q-wave myocardial infarction. Results of the TIMI IIIB Trial. Thrombolysis in Myocardial Ischemia. Circulation. 1994. 89:1545–1556.
13. Mintz GS, Nissen SE, Anderson WD, Bailey SR, Erbel R, Fitzgerald PJ, Pinto FJ, Rosenfield K, Siegel RJ, Tuzcu EM, Yock PG. American College of Cardiology clinical expert consensus document on standards for acquisition, measurement and reporting of intravascular ultrasound studies (IVUS): a report of the American College of Cardiology Task Force on Clinical Expert Consensus Documents. J Am Coll Cardiol. 2001. 37:1478–1492.
14. Nair A, Kuban BD, Tuzcu EM, Schoenhagen P, Nissen SE, Vince DG. Coronary plaque classification with intravascular ultrasound radiofrequency data analysis. Circulation. 2002. 106:2200–2206.
15. Rodriguez-Granillo GA, García-García HM, Mc Fadden EP, Valgimigli M, Aoki J, de Feyter P, Serruys PW. In vivo intravascular ultrasound-derived thin-cap fibroatheroma detection using ultrasound radiofrequency data analysis. J Am Coll Cardiol. 2005. 46:2038–2042.
16. Okubo M, Kawasaki M, Ishihara Y, Takeyama U, Yasuda S, Kubota T, Tanaka S, Yamaki T, Ojio S, Nishigaki K, Takemura G, Saio M, Takami T, Fujiwara H, Minatoguchi S. Tissue characterization of coronary plaques: comparison of integrated backscatter intravascular ultrasound with virtual histology intravascular ultrasound. Circ J. 2008. 72:1631–1639.
17. Yamamoto M, Takano M, Okamatsu K, Murakami D, Inami S, Xie Y, Seimiya K, Ohba T, Seino Y, Mizuno K. Relationship between thin cap fibroatheroma identified by virtual histology and angioscopic yellow plaque in quantitative analysis with colorimetry. Circ J. 2009. 73:497–502.
18. Davies MJ, Thomas A. Thrombosis and acute coronary-artery lesions in sudden cardiac ischemic death. N Engl J Med. 1984. 310:1137–1140.
19. Farb A, Burke AP, Tang AL, Liang TY, Mannan P, Smialek J, Virmani R. Coronary plaque erosion without rupture into a lipid core. A frequent cause of coronary thrombosis in sudden coronary death. Circulation. 1996. 93:1354–1363.
20. Virmani R, Kolodgie FD, Burke AP, Farb A, Schwartz SM. Lessons from sudden coronary death: a comprehensive morphological classification scheme for atherosclerotic lesions. Arterioscler Thromb Vasc Biol. 2000. 20:1262–1275.
21. Falk E, Shah PK, Fuster V. Coronary plaque disruption. Circulation. 1995. 92:657–671.
22. Silva JA, Escobar A, Collins TJ, Ramee SR, White CJ. Unstable angina: a comparison of angioscopic findings between diabetic and nondiabetic patients. Circulation. 1995. 92:1731–1736.
23. Hong MK, Mintz GS, Lee CW, Suh J, Kim JH, Park DW, Lee SW, Kim YH, Cheong SS, Kim JJ, Park SW, Park SJ. Comparison of virtual histology to intravascular ultrasound of culprit coronary lesions in acute coronary syndrome and target coronary lesions in stable angina pectoris. Am J Cardiol. 2007. 100:953–959.
24. Libby P. Molecular bases of the acute coronary syndromes. Circulation. 1995. 91:2844–2850.
25. Davies MJ, Richardson PD, Woolf N, Katz DR, Mann J. Risk of thrombosis in human atherosclerotic plaques: role of extracellular lipid, macrophage, and smooth muscle cell content. Br Heart J. 1993. 69:377–381.
26. Buja LM, Willerson JT. Role of inflammation in coronary plaque disruption. Circulation. 1994. 89:503–505.
27. Moscucci M, Fox KA, Cannon CP, Klein W, López-Sendón J, Montalescot G, White K, Goldberg RJ. Predictors of major bleeding in acute coronary syndromes: the Global Registry of Acute Coronary Events (GRACE). Eur Heart J. 2003. 24:1815–1823.
28. Rao SV. Implications of bleeding and blood transfusion in percutaneous coronary intervention. Rev Cardiovasc Med. 2007. 8:S18–S26.
29. Bassand JP. Impact of anaemia, bleeding and transfusions in acute coronary syndromes: a shift in the paradigm. Eur Heart J. 2007. 28:1273–1274.
30. Go AS, Yang J, Ackerson LM, Lepper K, Robbins S, Massie BM, Shlipak MG. Hemoglobin level, chronic kidney disease, and the risks of death and hospitalization in adults with chronic heart failure: the Anemia in Chronic Heart Failure: Outcomes and Resource Utilization (ANCHOR) study. Circulation. 2006. 113:2713–2723.